Abstract
We review the continuous monitoring of a qubit through its spontaneous emission, at an introductory level. Contemporary experiments have been able to collect the fluorescence of an artificial atom in a cavity and transmission line, and then make measurements of that emission to obtain diffusive quantum trajectories in the qubit's state. We give a straightforward theoretical overview of such scenarios, using a framework based on Kraus operators derived from a Bayesian update concept; we apply this flexible framework across common types of measurements including photodetection, homodyne, and heterodyne monitoring and illustrate its equivalence to the stochastic master equation formalism throughout. Special emphasis is given to homodyne (phase-sensitive) monitoring of fluorescence. The examples we develop are used to illustrate basic methods in quantum trajectories, but also to introduce some more advanced topics of contemporary interest, including the arrow of time in quantum measurement, and trajectories following optimal measurement records derived from a variational principle. The derivations we perform lead directly from the development of a simple model to an understanding of recent experimental results.
Acknowledgements
We are grateful to Joe Murphree for helpful comments and suggestions. Our numerical methods are written in Python (version 2.7.16). P.L. thanks the Quantum Information Machines school at École de Physique des Houches for their hospitality during part of this article's preparation.
Disclosure statement
No potential conflict of interest was reported by the author(s).
Notes
1 The quadrature space of the field is effectively the phase space of the quantum harmonic oscillator describing the field mode in question. In other words, a quadrature is analogous to the ‘position’ or the ‘momentum’ of a quantum harmonic oscillator, and the product of the noise in orthogonal directions in quadrature space is bounded by the Heisenberg uncertainty principle. A reader unfamiliar with a quadrature phase space representation of a field mode may benefit from perusing e.g. Refs. [Citation65,Citation66]
2 Photodetection, as considered above, constitutes a particular ‘unravelling’ of the master equation into stochastic trajectories; the heterodyne and homodyne measurements we subsequently consider are additional possible ‘unravelings’.
3 Strictly speaking, writing is an odd mathematical statement, because
is pure noise and non-differentiable. In practice such substitutions does not cause us a problem in writing down sensible stochastic calculus however. For details, refer e.g. to the books by Gardiner [Citation9,Citation63], or other references on stochastic differential equations, such as [Citation64].
4 Formally, is more appropriate; the way the integration of the diffusion term, over
, is carried out is both significant and potentially ambiguous. See chapter 4 of [Citation63] for rigorous derivations and more detailed comments.
5 Note that this description is associated with a frame rotating at frequency , or equivalently the interaction picture with respect to
. In the fixed frame, the qubit Hamiltonian in presence of the drive reads
[Citation56].
6 Quantum state smoothing is closely related to quantum trajectories; SQTs, as we have presented them in the present text, are a form of ‘quantum filtering’ which goes forward in time; in other words, we here only use the measurement record from the system's past to estimate a qubit's state. In the event of an inefficient measurement, quantum state smoothing often allows for a more pure estimate of the system's state to be made at some time, by using the measurement record both before and after the time at which the state is estimated.
7 We are grateful for comments by Prof. Miguel Alonso which helped us to clarify this point in our own thinking.
8 The LM in question has primarily been used in the context of multipath dynamics [Citation30,Citation48] there the main concern is whether the projection of the time-evolved LM out of the full OP phase space down into the -space of final quantum states is one-to-one (a single MLP connects the initial state to the chosen final state) or many-to-one (in which case many OPs may connect the boundary conditions, typically corresponding to different clusters of SQTs in the post-selected distribution).
9 Note that we have derived both and
using regular calculus, and are thereby effectively using the Stratonovich form of
, not the Itô form which arises directly from the SME (Equation21
(21)
(21) ). Using a form of
which does not transform according to regular calculus would prevent us from performing our OP analysis using typical approaches of classical mechanics (e.g. canonical transformations), which we find quite undesirable.
10 For example, starting at , a globally more-likely path which we would regard as typical might arise from post-selecting on
(≈ ground) at some later time, picking out a solution from X+. However, it is possible to post-select on some state like
(also ≈ ground) instead, selecting a path from X- or Y-; this reveals the possibility of a much rarer set of events, corresponding to an OP which circles back through the excited state before decaying towards ground, from the opposite direction as compared with the more typical set of paths. Post-selections drawing out these dynamics can generically select OPs from regions X or Y of the phase portrait, as detailed in Figure ; those in region Z can only partially re-excite, before turning around to decay.
11 One could say that we, the observer, are updating our probabilistic prediction about outcomes of a future measurement; this ‘best guess’ about possible future outcomes is made based on some preparation procedure, past measurement record, and the rules we typically refer to as ‘quantum mechanics’.
12 A coherent state mimics the classical behaviour we associate with a simple harmonic oscillator, to the extent allowed by quantum mechanics. It follows the same phase-space (quadrature space) ellipses as in classical mechanics, just as a Gaussian packet with the minimum quadrature uncertainty product allowed by the Heisenberg uncertainty principle. One could associate the oscillatory behaviour of the coherent state, here used to describe the beam of e.g. a monochromatic laser, with whatever ‘waves’ in the classical free-space electromagnetic description of light. Mathematically is an eigenstate of the photon destruction operator, i.e.
.
Additional information
Funding
Notes on contributors
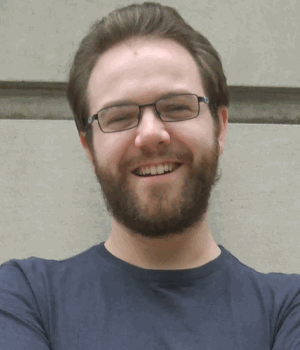
Philippe Lewalle
Philippe Lewalle is a Ph.D. Candidate at the University of Rochester, studying quantum measurement under Prof. Andrew N. Jordan. He also did his undergraduate studies at the University of Rochester, graduating with degrees in music and physics in 2014. His research, within the area of quantum information, has included investigations of the behaviour of extremal-probability quantum trajectories, time-continuous measurements of multi-qubit systems that entangle those qubits, and applications of weak measurements and weak values to precision measurement.
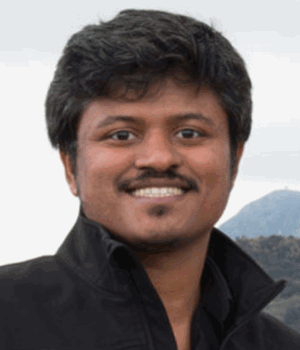
Sreenath K. Manikandan
Sreenath K. Manikandan is a graduate student at the University of Rochester, NY with Prof. Andrew N. Jordan. He completed his integrated Bachelor of Science-Master of Science degree from the Indian Institute of Science Education and Research – Thiruvananthapuram, Kerala, India, in 2015. His research interests include topics in quantum thermodynamics, quantum measurements, and applications to mesoscopic quantum heat engines and refrigerators.
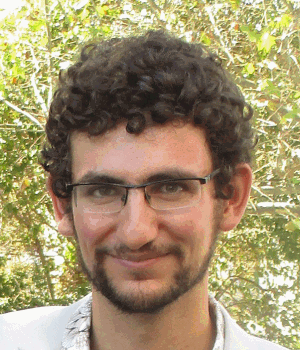
Cyril Elouard
Cyril Elouard is a Postdoctoral Associate at the University of Rochester, NY since 2017. He did his undergraduate studies at the Ecole Normale Superieure of Lyon, France. He has defended his PhD ‘Thermodynamic of Quantum Open Systems: Applications in Quantum Optics and Quantum Optomechanics’ at the University Grenoble-Alpes in 2017, in the group of Alexia Auffèves. His research interests cover quantum measurement, quantum optics, thermodynamics of quantum systems and optomechanical and nanoelectronic setups.
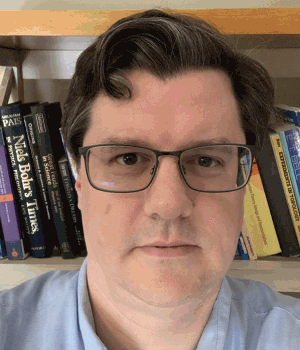
Andrew N. Jordan
Prof. Andrew N. Jordan received his B.S. in Physics and Mathematics (1997) from Texas A&M University and his Ph.D. in Theoretical Physics (2002) from the University of California, Santa Barbara, supervised by Prof. Mark Srednicki. He was a postdoctoral fellow at the University of Geneva (2002–2005) with Prof. Markus Büttiker, and a research scientist at Texas A&M (2005–2006) with Prof. Marlan Scully. He joined the University of Rochester as Assistant Professor of Physics in 2006, was promoted to Associate Professor with Tenure in 2012, and full Professor in 2015. His research interests are in Quantum Physics, Condensed Matter Physics, and Quantum Optics. Themes of interest include nanophysics, quantum measurement and control, quantum information, and quantum thermodynamics.