Abstract
The aim of this study was to analyse the convergence of two methods by comparing exposure and the assessed risk of developing musculoskeletal disorders at 18 repetitive task workstations. The already established occupational repetitive actions (OCRA) and the recently developed upper limb risk assessment (ULRA) produce correlated results (R = 0.84, p = 0.0001). A discussion of the factors that influence the values of the OCRA index and ULRA's repetitive task indicator shows that both similarities and differences in the results produced by the two methods can arise from the concepts that underlie them. The assessment procedure and mathematical calculations that the basic parameters are subjected to are crucial to the results of risk assessment. The way the basic parameters are defined influences the assessment of exposure and risk assessment to a lesser degree. The analysis also proved that not always do great differences in load indicator values result in differences in risk zones.
Abstract
Practitioner Summary: We focused on comparing methods that, even though based on different concepts, serve the same purpose. The results proved that different methods with different assumptions can produce similar assessment of upper limb load; sharp criteria in risk assessment are not the best solution.
Keywords::
Introduction
Work-related musculoskeletal load is considered the main risk factor in the development of musculoskeletal disorders (MSDs) (Kumar Citation2001; Sande et al. Citation2001; Coury and Padula Citation2002). Therefore, exposure assessment is a crucial step in protecting workers from developing MSDs. Repeated effort, resulting in continuous loading of tissue structures or lack of recovery, triggers a pathological process (Kim and Lee Citation2010). Repetitive tasks mostly involve the upper limbs, so the upper limbs are at greatest risk of MSDs caused by repetitive movements (Zetterberg et al. Citation1997; Bernaards et al. Citation2007). Procedures and methods for assessing the musculoskeletal load of the upper limbs and thus for predicting the risk of developing upper limb MSDs depend on parameters related to biomechanical factors, i.e. the exerted force and posture in given time sequences (van der Beek and Frings-Dressen Citation1998; Kee and Karwowski Citation2001). Musculoskeletal load leading to the development of MSDs can have its source in work with high forces and/or awkward postures (Karwowski et al. Citation1999; Keir and Brown Citation2012). However, MSDs also result from work tasks in which, e.g., a motionless posture is sustained for a long time (Johnston et al. 2008) or repetitive tasks with low exerted forces are performed (Quintana and Hernandez-Masser Citation2003). This justifies the need for assessment based on these three factors. Therefore, in assessing the load of the musculoskeletal system and the risk of developing MSDs parameters related to posture, exerted forces and time in which tasks are completed should be considered jointly.
Upper limb exposure caused by repetitive tasks can be assessed, e.g. by applying occupational repetitive actions (OCRA) (EN Citation1005-5:2007) or upper limb risk assessment (ULRA) (Roman-Liu Citation2005, Citation2007).
OCRA is a commonly applied method of evaluating the musculoskeletal load of the upper limbs caused by repetitive tasks and the risk of developing MSDs (Occhipintini Citation1998). OCRA is rather dedicated to movements of the arms below the shoulder level. It focuses on movements of the forearms without differentiating exposure caused by the posture of the arms. Standard EN1005-5:2007 includes procedures for assessing risk with OCRA.
The recently developed ULRA focuses on a precise definition of any upper limb posture and considers the arm, the forearm and the hand in each phase of the cycle. The parameters that define the upper limb posture, force and repetitiveness produce the repetitive task indicator (RTI), the value of which defines the upper limb load.
OCRA and ULRA are based on different concepts; however, they assess the same measure, i.e. the upper limb load resulting from repetitive tasks. Both methods assess the upper limb load on the basis of body posture, exerted forces and time. However, these methods primarily differ in how they identify variables that describe posture, forces and time sequences. The assumption underlying this study was that even if different methods use the same input data in assessing the same work tasks, they can still produce different load assessments. Therefore, the aim of this study was to analyse the convergence of the two methods by comparing their assessment of the upper limb load and the risk of developing MSDs at 18 repetitive task workstations.
Methods
Ocra And Ulra
Both OCRA and ULRA rely on parameters that define the upper limb posture, force and duration of each activity. However, the way they combine and weight each parameter is different. Moreover, the methods define posture, force and task duration differently.
The OCRA index estimates exposure and evaluates occupational risk of developing MSDs caused by repetitive work of the upper limbs (EN 1005-5:2007). The basic concept in OCRA is technical action, defined as a complex movement to complete a work task, which involves one or more joints of the upper limb. The OCRA index is calculated as a ratio of the foreseeable frequency (FF) of technical actions per minute and the corresponding number of recommended actions, called the reference frequency (RF) of technical actions per minute. RF is the product of seven multipliers: posture multiplier (PoM), force multiplier (FoM), repetitiveness multiplier (ReM), recovery multiplier (RcM), duration multiplier (DuM), additional factors multiplier (AdM) and a constant of frequency (30 actions per minute) of technical actions per minute (CF).
ULRA expresses the upper limb load and the risk of developing MSDs with RTI. RTI is a mathematical equation that expresses the upper limb load as a function of the duration of an individual cycle (CT), the number of activities in one cycle (k) and the duration of a given activity. Each change in the upper limb posture or force is defined as an individual phase. This means that one OCRA's technical action can be an equivalent of more than one ULRA's phase. The force required in each cycle phase is determined by the relative force, i.e. a ratio of the value of exerted force to maximum force (Roman-Liu Citation2007). As ULRA is a new method, it is described in more detail in Appendix.
OCRA is described well in standard EN 1005-5:2007 with a three-zone risk system. Occhipinti and Colombini (Citation2007) updated three risk zone values and introduced subzones creating a six-zone risk system. In this way, there are two risk assessment systems: a three-zone risk system and a six-zone risk system. When considering OCRA, presented in EN1005-5:2007, both methods locate the obtained value of the musculoskeletal exposure indicator, the OCRA index or RTI, in one of the three zones (green, yellow and red) that define the risk of developing MSD as acceptable, conditionally acceptable or not acceptable (Table ). When considering the six-zone risk system (presented in Occhipinti and Colombini Citation2007), in order to make results of OCRA and ULRA comparable, RTI values were proportionally adjusted to OCRA zones.
Table 1 Risk zones in OCRA and ULRA (RTI).
Workstations
Eighteen workstations were analysed. The first step in assessing the upper limb exposure resulting from a specific work task is to identify the work schedule comprising all activities in a work cycle and their duration. The characteristics of the tasks that differentiated the workstations were cycle duration, the number of movements per minute, the exerted force and upper limb posture. Table briefly describes the workstations. All of them were assessed with the same procedure, with the two upper limbs considered separately. The limbs were involved in different work activities; therefore, their basic parameters were different. Consequently, the indicators and risk zone assessments were also frequently different.
Table 2 Workstations.
Basic parameters
The basic parameters that define the workstations discussed in this study are different in OCRA and ULRA, because the basic concepts underlying these methods are different. However, in both methods, the basic parameters identify postures, duration of work activities and force.
OCRA's technical action, which corresponds to ULRA's cycle phase, is defined as a complex movement to complete a work task involving more than one joint of the upper limb. This means that only upper limb postures involved in an activity are technical actions. Therefore, although foreseeable duration of the cycle time in seconds (FCT) equals CT in ULRA, the number of cycle phases (k) and the number of technical actions (NTC) can differ.
In ULRA, each cycle phase is differentiated by changes in the posture of the upper limb and/or in the exerted force. An upper limb posture is assigned to each cycle phase; seven angles and the type and value of force exerted during that phase define it. A cycle phase lasts as long as there is no variation in the upper limb posture and/or in the exerted force. If there is no activity and the upper limb is supported (this is a cycle phase, too), the force related to the upper limb posture is 0. The relative values of forces of specific cycle phases and the duration of each cycle phase were used in calculating integrated cycle load (ICL).
Data collection
In order to obtain basic parameters, work tasks performed at all 18 analysed workstations were filmed. In addition, angles and forces were measured. Each cycle phase was assigned the value of angles present in this phase. To measure the duration of cycle phases, the recorded film was analysed frame by frame.
The angles in the wrist and elbow were measured with electrogoniometers. The angles defining the arm posture were determined on the basis of the frames (pictures) filmed from different directions. The accepted values were averaged over three repetitions of randomly selected cycles. The angles of the joints were measured with a Biometrics (UK) goniometric system. Data were recorded on the data logger with the sampling frequency of 100/min. Then, data were sent to a personal computer via an RS232C serial port using DL1001 software v. 3.11.
Values of exerted forces were accepted on the basis of documentation, mass of tools or they were measured with a dynamometer (ZPC system from JBA, Poland). The subject was asked to press the dynamometer with the same force as when working. Force tests were repeated twice; those with the higher values were selected. When negligible forces were present during the task, the lowest value (according to ULRA) was assigned, which was 0.5 N. In order to calculate the relative value of force, the force value was related to the maximum force calculated for a given upper limb posture and a given force type (see Appendix).
Analysis
The analysis aimed at finding to what extent OCRA and ULRA were in step. Calculated upper limb exposure indicators (OCRA index and RTI) for both the left and right upper limbs and 18 workstations were the basis for analysing the influence of force and repetitiveness (determined by NCT and k), on the values of the risk indicators as well as for analysing the relationship between those indicators and the risk zones determined by their values.
Table presents the basic parameters for both methods, for each workstation. RTI is calculated as a function of ICL, CT and k (Appendix). In OCRA, FoM is estimated on the basis of the percentage of maximum force, assumed in the same way as in ULRA, i.e. as the same as ICL.
Table 3 Parameters defining repetitive tasks in ULRA (CT, cycle time; k, number of cycle phases in one cycle) and in OCRA (NTC, number of technical actions per cycle; FCT, foreseeable duration of the cycle time) at 18 workstations.
The OCRA index is calculated as a ratio of the FF of technical actions needed to carry out the task and the number of recommended actions (RF). RF is calculated as the product of CF (constant of frequency of technical actions) and the multipliers (PoM, FoM, ReM, AdM, RcM and DuM). In this analysis, PoM, FoM and ReM were assessed for each workstation. Three other multipliers were assumed to be the same for each workstation (AdM = 1, RcM = 0.6, DuM = 1). In case of each workstation, CF = 30 actions per minute.
In order to analyse the extent to which basic parameters affected indicators of exposure (OCRA, RTI), the indicators were expressed as a linear function of the number of actions per minute and as a function of the force of a cycle (ICL). The number of technical actions per minute (FF) is the numerator in the formula for the OCRA index. In ULRA, the number of actions per minute is represented with k multiplied by 60 and divided by CT.
In order to analyse the relationship between indicators, non-parametric Spearman correlation was used. In order to illustrate the relationship between risk zones, OCRA and ULRA risk zones were compared. Analysis also focused on percentage of at risk agreement and percentage of perfect agreement (Jones and Kumar Citation2010). Percentage of at risk agreement was calculated by considering cases when risk zones were divided into two areas: no risk (green) and at risk (other than no-risk zones). Percentage of perfect agreement was calculated by considering only the cases of exact agreement. The agreement between the assessments done with OCRA and ULRA was also analysed based on the cases of OCRA zones against ULRA's three-zone and six-zone systems (Kee and Karwowski Citation2007).
In order to compare the upper limb exposure and the risk assessment with OCRA and ULRA, the values of RTI were modified to obtain a linear relationship with a slope of 1 between OCRA and RTI. In this way, parameter RTT was obtained, the values of which were compared with the OCRA index. OCRA zones were set against ULRA zones.
Results
Table presents the results of an analysis done with OCRA and ULRA. It also lists intermediate parameters: PoM, RF and FF according to OCRA and ICL and the number of phases per minute according to ULRA.
Table 4 Indicators of musculoskeletal load of the left (L) and right (R) upper limbs in ULRA and OCRA.
RF, the crucial parameter in calculating the OCRA index, is the product of multipliers that depend on posture, repetitiveness and force. Because there were no additional factors on any of the workstations and repetitive work was done in each case for the whole 8 h, the three multipliers (AdM, RcM and DuM) were the same for each workstation. This means that they did not produce any differences in the risk assessment. PoM was different for different workstations (Table ). For most workstations ReM = 1, except for the Scanning and Circuit board assembly for both upper limbs, where ReM = 0.7.
The influence of force and repetitiveness on the final assessment conducted with OCRA and ULRA was analysed by establishing the relationship between risk indicators and the parameters (ICL and the number of actions per minute).
Figure (a) illustrates how the OCRA and RTI indices change their values as a function of number of actions per minute. The results show a slightly stronger Spearman correlation in RTI (0.95), than in OCRA (0.82). Figure (b) illustrates an influence of force on indicators; it shows values of the OCRA index and RTI as a function of ICL. Spearman correlation equalled 0.75 in all cases; p < 0.001.
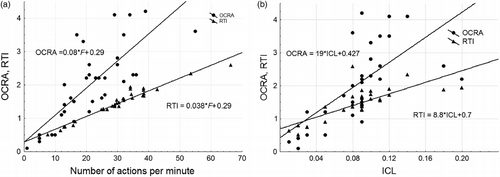
Although there is a correlation between OCRA indexes and ULRA (0.84 at p < 0.001), their individual values differ (Figure ). The slope in a linear equation, expressing relationship between the OCRA index and RTI, was 1 when RTI was multiplied by 2.2 and value of 1.06 was subtracted. Analysis of differences between OCRA index and RTT showed that in half of all cases the differences were below 20%, whereas in 42% of cases they were between 21% and 50% (Table ). Only in 33% of cases, the differences between OCRA and ULRA indicators corresponded to changes in the number of actions per minute. When comparing differences between indicators with differences in number of actions per minute in both methods, correlation was 0.34 with p = 0.041. Analysis proved lack of correlation between the values representing differences between OCRA index and RTT and ICL.
Table 5 Differences in indicators of upper limb exposure obtained with OCRA and ULRA (values with – indicate that values obtained with OCRA are higher than the values of RTT: RTT = 2.2 * RTI − 1.06).
The greatest differences between the values of the indicators for OCRA and RTT were for the right limb on Controller workstation (111%). The second greatest was Circuit board operator – left limb (61%). In both cases, the assessment according to OCRA and the assessment according to ULRA located risk in the green area (Tables and ). On the other hand, for the right upper limb, the differences between OCRA index and RTT were below 20% in Circuit board assembly, Sewing headrest and Socket assembly. For the left upper limb, the differences were below 20% in circuit board operator, manual assembly, sewing car seat and checking appearance workstations. However, in all these cases, OCRA assessed risk in the green area, whereas ULRA in the yellow area.
Figure presents cases of OCRA zones against ULRA zones. Figure (a),(b) illustrates the parts of analysis that focused on a three- and six-zone risk assessment, respectively.
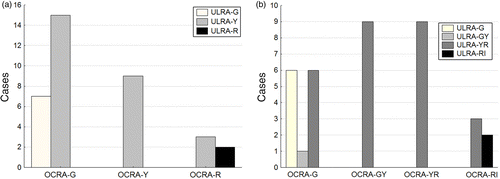
When the relationship between risk assessment zones was compared, there was 64% in at risk agreement and 47% in perfect agreement. When a three-zone risk system was taken into account, at risk agreement and perfect agreement showed 39% and 58%, respectively.
Discussion
In most cases, the values of the indicators obtained with OCRA and ULRA were similar. In addition, the correlation between these indicators was quite strong. However, in 8% of the cases, there were differences of above 50%, with sometimes one and sometimes the other indicator higher.
The differences can be discussed with respect to the values of indices and with respect to risk assessment. There were cases when great differences in the upper limb exposure did not result in different risk zones (circuit board operator – left limb and controller – right limb), whereas small differences (below 20%) between the values of the indicators for OCRA and RTT resulted in risk classified in different zones (seven cases). This suggests that using different methods can produce not just differences in exposure assessment but also differences in assignment to risk zones. Thus, a sharp distinction between the zones may not be a good solution in workstation assessment. This supports the thesis that the researcher or practitioner should not rely on the output of a single risk assessment only.
The concepts adopted in OCRA and ULRA might underlie both their similarities and differences. In OCRA, FCT and NTC determine the duration and number of activities. In ULRA, CT and k define them. FCT and CT were the same for individual workstation. However, NTC and k differed at most of them (Table ). As a result of different definitions, OCRA counts technical actions, whereas ULRA counts cycle phases. NTC counts only performed activities, and k expresses all tasks, also when the upper limb is supported and no force is exerted. These parameters determined the differences in the number of actions per minute in both methods, which could have influenced the values of the indicators (Figure (a)). The values of the indicators are also influenced by ICL (Figure (b)). Both force and number of actions per minute influence the OCRA index more than RTI.
Another difference is that OCRA specifies ‘organisational’ factors such as daily duration of repetitive tasks and a pattern of recovery periods. These factors affect the values of the OCRA index at workstations. In this study, these factors were considered constant not influencing either the OCRA index or the RTI. ULRA considers those aspects indirectly, rather than directly. The concept adopted in ULRA considers all activities performed during the work day. ULRA assesses the work load related not only to repetitive tasks but also to all tasks performed during the work day. So, in this way, this issue is addressed when work at the examined workstations consists of various types of tasks, not just repetitive tasks.
The fact that OCRA index depends on the number of activities per minute to a greater extent than RTI can be responsible for cases when OCRA has higher values and the results are not in step. If the number of actions per minute is the source of discrepancies between methods, differences between the number of actions would be proportional to differences in indexes (Table ). However, it is not. The greatest differences between OCRA and RTT occurred for the Controller operator workstation for the right upper limb (Table ). For that case, there were no differences between the methods in the number of actions per minute. On the other hand, there were cases where those differences were over 20% without strong differences in the values of the indicators. In seven cases, the differences between the number of actions per minute counted according to RTT and OCRA were below 20%. This supports the hypothesis that differences between the counted number of actions per minute could have been one of the sources of differences in the assessment of the upper limb exposure (Table ). However, this parameter is not the only factor responsible for differences between the OCRA and ULRA results; other factors are meaningful too.
FoM in OCRA can be estimated on the basis of the Borg scale or maximum isometric force (Borg 1985). Force in ULRA is defined as the relative value of force, which refers strictly to a given type of force and a given upper limb posture. Attempts have been made to ask workers to rate their effort on a Borg scale. However, they usually reported not on force only, but on a combination of force and repetitiveness. Therefore, ICL was accepted for estimating force and determining FoM in OCRA. In this respect, calculations according to ULRA have a common point with OCRA. Thus, it is possible that this element should reduce the differences between the methods. On the other hand, ICL expresses percentage of maximum force, and the force assessed by the worker should be in step with that calculated as ICL. Both the Borg scale and ICL express the relative force. Both are good measures; however, both can be biased. Force, as the basic parameter, was the same in both methods. However, Figure (b) indicates that it has stronger influence on OCRA.
The type of workstation might influence the results too. The workstations were divided into those where mostly movements of the forearms and hands were involved (12 workstations) and those where movement was up to the shoulder level (6 workstations), without passing that level, though. An analysis of differences between the indicators obtained with both methods showed lack of statistical differences.
All this suggests that the differences between definitions of basic parameters like number of actions per minute or relative force in cycle can be one of the factors influencing differences in indictors and that its influence on the values of the indices is also determined by other factors that are important in assessing the upper limb exposure. This indicates that the procedure, which combines parameters in the upper limb exposure assessment, determines the differences. In OCRA, the procedure can be described as more discrete where multipliers adopt one of a limited number of values. In ULRA, the procedure permits a more continuous calculation of RTI. This can also influence the differences between the methods. ULRA differentiates duration of the cycle and repeatability of movement independently of one another. The value of RTI increases gradually with an increase in the repetitiveness of movements. However, for the same value of the parameter that expresses repetitiveness, RTI for a longer cycle adopts lower values than for a shorter cycle. In OCRA, repetitiveness is expressed with ReM, the value of which decreases from 1 to 0.7 when the cycle is under 15 s or when the task requires doing the same technical actions of the upper limbs for at least 50% of CT. Force in ULRA is represented by ICL, which changes continuously together with change in exerted force and/or in upper limb posture. In OCRA, force multipliers are discrete and represent ranges of force.
The discrete nature of OCRA and the more continuous nature of ULRA may then be a source of the differences. A meticulous definition can improve the precision and reliability of a method. However, a method can use the interval of posture, time or force, and still be reliable. The precision of a method is not always related to its reliability. Even though lack of precision does not necessarily imply lack of accuracy, the length of the interval can be important. In the case of OCRA, the range of angles defining wrist and elbow posture is divided into two areas only. Moreover, these ranges are substituted by multipliers. Precision is lower than when discrete values substitute ranges.
It can then be concluded that discrepancies between the assessments obtained with OCRA and ULRA are mostly related to the type of calculation procedure and a combination of basic parameters are responsible for them. This further leads to the conclusion that although a good risk assessment tool should provide insight into which variables need to be changed to obtain the greatest reduction in risk, this is not always simple. Parameters that describe the body posture, forces and duration of activities combine, and the relationship between them and a risk indicator is not always linear. Therefore, in order to reduce risk, a combination of parameters rather than a single parameter should be considered.
Data from OCRA and ULRA were compared by assessing the percentage of agreement and the linear relationship. When comparing the linear relationship between the data characterising the tasks and indicators of the upper limb load (OCRA index and RTI), the comparison was equivalent, e.g. data on the number of actions per minute and force were common for both methods. However, it is necessary to remember that the different definitions of input data, regarding the frequency of movements, could affect the validity of a comparison of the OCRA index and RTI in terms of values and zones. Thus, differences between the OCRA index and RTI could be shown for some workstations only, as discussed earlier.
The choice of methods should always consider requirements such as data, accuracy and the investigator's precision and skill (David Citation2005). When comparing the methods, the amount of time necessary to complete an assessment is also important. In this study, input parameters were a basis for preparing a work schedule, which was the most time-consuming part of analysis, in both methods. The fact that input data were quantitative and resulted from objective measurements increased the time it took to assess a workstation. However, this also meant that risk assessment was more precise. The work schedule required recognising sequences of operations, their duration and the posture of the upper limb. In comparison with OCRA, ULRA requires determining the upper limb posture in the arm region. This, admittedly, requires slightly more time. In spite of that the time necessary to conduct analysis in both methods is quite similar; it mostly depends on the number of operations and tasks in one cycle. It also depends on the skills of the expert, who can be more proficient in one of the methods.
Correlation between OCRA and RTI obtained in this study is high, even though there are discrepancies between OCRA and RTI. OCRA was often used for the ULRA; it was also compared with other methods. Jones and Kumar (Citation2010) found quite high-risk agreement between OCRA and RULA and REBA. However, when using OCRA and RULA (McAtamney and Corlett Citation1993), Serranheira and de Sousa Uva (Citation2008) obtained divergent results of risk assessment. In that study, OCRA was moderately correlated with SI (Moore and Garg Citation1995) and with HAL (Latko et al. Citation1997). Drinkaus et al. (Citation2003), Russell et al. (Citation2007), and van der Beek et al. (Citation2005) also found differences between the methods. Also Joseph, Imbeau, and Nastasia (Citation2011) showed that OCRA was fairly consistent in describing the exposure. Those results, like the results of this study, suggest that proper workstation assessment may require using more than one method.
Both OCRA and ULRA are dedicated to assessing exposure of the upper limb only. Proper risk assessment should involve assessment of risk related to the whole body. Therefore, assessment with OCRA or ULRA should be supplemented with other methods dedicated to, i.e., trunk exposure assessment. In the process of assessing, the location of the upper limbs in relation to the trunk is also important. Registering arm posture makes that possible when ULRA is used. In the case of OCRA, the definition of the location of the upper limb relates to the forearm and wrist only, which restricts OCRA to workstations where workers' upper limbs are in front of them. OCRA can provide a separate index for the shoulder (Chiasson et al. Citation2012). According to Occhipintini (Citation1998), the index for the shoulder should be used only when the task requires large, dynamic movements of the shoulder, in movements exceeding 50% of the full joint range.
This study's limitations mostly lie in only 18 workstations that were analysed. More workstations would make it possible to study more relationships between the parameters that describe work tasks in each method. This would produce more data to help determine the influence of those parameters on OCRA and RTI indicators and to analyse the correlation of the OCRA index and RTI. The fact that ULRA is based on a complicated equation is a limitation too. However, as nowadays computerisation makes calculations quick, that limitation is less crucial.
Conclusions
The results of this study indicate that force has a stronger influence on OCRA than on ULRA. The way the basic parameters are defined influences exposure and risk assessment, which means that it is important to assess musculoskeletal load and risk. However, the assessment procedure and mathematical calculations that the basic parameters are subjected to are crucial. The results also prove that great differences in the values of load indicators do not always result in differences in risk zones.
Acknowledgements
This paper was prepared on the basis of the results of a research task carried out within the scope of the first stage of the National Programme ‘Improvement of safety and working conditions’ partly supported by the Ministry of Labour and Social Policy. The Central Institute for Labour Protection – National Research Institute was the Programme's co-ordinator.
The authors would like to thank Jadwiga Kozińska-Korczak for her technical help in the preparation of this paper.
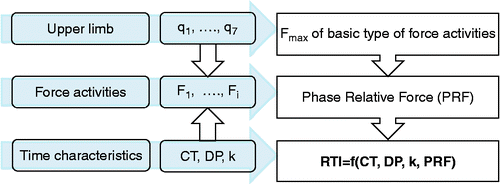
REFERENCES
- Bernaards, C. M., G. A. M.Ariëns, D. L.Knol, and V. H.Hildebrandt. 2007. “The Effectiveness of a Work Style Intervention and a Lifestyle Physical Activity Intervention on the Recovery from Neck and Upper Limb Symptoms in Computer Workers.” Pain132 (1–2): 142–153.
- Borg, G.1985. An Introduction to Borg's RPE-Scale. Ithaca, NY: Movement Publications.
- Chiasson, M.-E., D.Imbeau, K.Aubry, and A.Delisle. 2012. “Comparing the Results of Eight Methods Used to Evaluate Risk Factors Associated with Musculoskeletal Disorders.” International Journal of Industrial Ergonomics42: 478 − 488.
- Coury, H. G., and R.Padula. 2002. “Trunk Movements and Load Support Strategy in Simulated Handling Tasks Carried Out by Workers with and without Musculoskeletal Symptoms.” Clinical Biomechanics17 (4): 309–311.
- David, G. C.2005. “Ergonomic Methods for Assessing Exposure to Risk Factors for Work-Related Musculoskeletal Disorders.” Occupational Medicine55 (3): 190–199.
- Drinkaus, P., R.Sesek, D.Bloswick, T.Bernard, B.Walton, B.Joseph, G.Reeve, and J. H.Countus. 2003. “Comparison of Ergonomic Risk Assessment Outputs from Rapid Upper Limb Assessment and the Strain Index for Tasks in Automotive Assembly Plants.” Work21 (2): 165–172.
- European Committee for Standardization (CEN). 2007. EN 1005-5:2007. Safety of Machinery – Human Physical Performance – Part 5: Risk Assessment for Repetitive Handling at High Frequency.
- Johnston, V., G. Jull, R. Darnell, N. Jimmieson, and T. Souvlis. 2008. “Alterations in Cervical Muscle Activity in Functional and Stressful Tasks in Female Office Workers with Neck Pain.” European Journal of Applied Physiology 103 (3): 253–264.
- Jones, T., and S.Kumar. 2010. “Comparison of Ergonomic Risk Assessment Output in Four Sawmill Jobs.” International Journal of Occupational Safety and Ergonomics16 (1): 105–111.
- Joseph, C., D.Imbeau, and I.Nastasia. 2011. “Measurement Consistency Among Observational Job Analysis Methods During an Intervention Study.” International Journal of Occupational Safety and Ergonomics17 (2): 139–146.
- Karwowski, W., W.Lee, B.Jamala, P.Gaddie, R. L.Jang, and K. K.Alquesani. 1999. “Beyond Psychophysics: The Need for a Cognitive Engineering Approach to Setting Limits in Manual Lifting Tasks.” Ergonomics42 (1): 40–60.
- Kee, D., and W.Karwowski. 2001. “LUBA: An Assessment Technique for Postural Loading on the Upper Body Based on Joint Motion Discomfort and Maximum Holding Time.” Applied Ergonomics32 (4): 357–366.
- Kee, D., and W.Karwowski. 2007. “A Comparison of Three Observational Techniques for Assessing Postural Loads in Industry.” International Journal of Occupational Safety and Ergonomics13 (1): 3–14.
- Keir, P. J., and M. M.Brown. 2012. “Force, Frequency and Gripping Alter Upper Extremity Muscle Activity during a Cyclic Push Task.” Ergonomics55 (7): 813–824.
- Kim, S. L., and J. E.Lee. 2010. “Development of an Intervention to Prevent Work-Related Musculoskeletal Disorders among Hospital Nurses Based on the Participatory Approach.” Applied Ergonomics41 (3): 454–460.
- Kumar, S.2001. “Theories of Musculoskeletal Injury Causation.” Ergonomics44 (1): 17–47.
- Latko, W. A., T. J.Armstrong, J. A.Foulke, G. D.Herrin, R. A.Rabourn, and S. S.Ulin. 1997. “Development and Evaluation of an Observational Method for Assessing Repetition in Hand Tasks.” American Industrial Hygiene Association Journal58 (4): 278–285.
- McAtamney, L., and E. N.Corlett. 1993. “RULA: A Survey Method for the Investigation of Work-Related Upper Limb Disorders.” Applied Ergonomics24 (2): 91–99.
- Moore, J., and A.Garg. 1995. “The Strain Index: A Proposed Method to Analyze Jobs for Risk of Distal Upper Extremity Disorders.” American Industrial Hygiene Association Journal56 (5): 442–458.
- Occhipintini, E.1998. “OCRA: A Concise Index for the Assessment of Exposure to Repetitive Movements of the Upper Limb.” Ergonomics41: 1290–1311.
- Occhipinti, E., and D.Colombini. 2007. “Updating Reference Values and Predictive Models of the OCRA Method in the Risk Assessment of Work-Related Musculoskeletal Disorders of the Upper Limbs.” Ergonomics50 (11): 1727–1739.
- Quintana, R., and V.Hernandez-Masser. 2003. “Limiting Design Criteria Framework for Manual Electronics Assembly.” Human Factors and Ergonomics in Manufacturing13 (2): 165–179.
- Roman-Liu, D.2005. “Upper Limb Load as a Function of Repetitive Task Parameters: Part 1 – A Model of the Upper Limb Load.” International Journal of Occupational Safety and Ergonomics11 (1): 93–102.
- Roman-Liu, D.2007. “Repetitive Task Indicator as a Tool for Assessment of Upper Limb Musculoskeletal Load Induced by Repetitive Tasks.” Ergonomics50 (11): 1740–1760.
- Russell, S. J., L.Winnemuller, J. E.Camp, and W.Johnson. 2007. “Comparing the Results of Five Lifting Analysis Tools.” Applied Ergonomics38 (1): 91–97.
- Sande, L. P., H. J. G.Coury, J.Oishi, and S.Kumar. 2001. “Effect of Musculoskeletal Disorders on Prehension Strength.” Applied Ergonomics32 (6): 609–616.
- Serranheira, F., and A.de Sousa Uva. 2008. “Work-Related Upper Limb Musculoskeletal Disorders (WRULMSDs) Risk Assessment: Different Tools, Different Results! What are We Measuring?” Medicina y Seguridad del Trabajo54 (212): 35–44.
- van der Beek, A. J., and M. H. V.Frings-Dressen. 1998. “Assessment of Mechanical Exposure in Ergonomic Epidemiology.” Occupational and Environmental Medicine55 (5): 291–299.
- van der Beek, A. J., S. E.Mathiassen, J.Windhorst, and A.Burdorf. 2005. “An Evaluation of Methods Assessing the Physical Demands of Manual Lifting in Scaffolding.” Applied Ergonomics36 (2): 213–222.
- Zetterberg, C., A.Forsberg, E.Hansson, H.Johansson, P.Nielsen, B.Danielsson, G.Inge, and B. M.Olsson. 1997. “Neck and Upper Extremity Problems in Car Assembly Workers. A Comparison of Subjective Complaints, Work Satisfaction, Physical Examination and Gender.” International Journal of Industrial Ergonomics199 (4): 277–289.
Appendix: Description of application of ULRA to assessment of unpacking circuit board workstation
ULRA assesses upper limb load based on parameters that describe upper limb posture, exerted forces and duration of particular activities. The RTI expresses upper limb load, which results from work described with those parameters. Assessment procedures of the ULRA method have already been described elsewhere (Roman-Liu Citation2005, Citation2007). Unpacking circuit board workstation is example of how ULRA is used to assess risk.
ULRA calculations are based on basic parameters, which refer to upper limb posture, forces, duration of activities and number of activities (Figure ).
The unpacking circuit board workstation is an example of a situation with a various number of tasks during an operation; some operations are repeated (Table ). The left and right upper limbs often perform different numbers of phases.
Table A1 Work schedule of tasks in each cycle phase at the unpacking circuit board workstation.
The first step in assessing the upper limb load resulting from a specific work task is to identify the work schedule comprising all activities of a work cycle and their duration. A cycle often consists of different operations, which are repeated during the cycle. Each operation consists of activities, which can be repeated during the operation. Activities are identified by differentiating the posture of the upper limb and/or the exerted force. Activities correspond to cycle phase. Each repetition increases the number of phases (k) and the time of the cycle (CT).
An upper limb posture assigned to each cycle phase is defined by seven angles: (q1) the angle of arm horizontal adduction/abduction; (q2) the angle of arm extension/flexion; (q3) the angle of forearm medial/lateral rotation along the long axis of arm; (q4) the angle of elbow flexion; (q5) the angle of forearm pronation/supination; (q6) the angle of wrist ulnar/radial deviation and (q7) the angle of extension/flexion.
All the seven angles that define upper limb posture is 0° in the natural position, which means with upper limbs hanging naturally down.
Table lists values of angles defining upper limb posture during cycle phases at the unpacking circuit board workstation.
Table A2 Values of angles defining the location of the right and left upper limbs in consecutive cycle phases at the unpacking circuit board workstation.
Force exerted at the workstation can be measured or taken from the documentation of the work process. The force expressing maximum force capabilities can be measured, too; however, it can also be calculated from an equation as a function of the seven angles of the upper limb posture that identify the location of the arm, the forearm and the hand. Predictive equations for such force types are presented in Table .
Table A3 Equations expressing basic force activities as a function of seven angles defining upper limb posture.
Each cycle phase considers all types of exerted forces. Performing any task is connected with at least the necessity of lifting the weight of the upper limb. However, usually there is also exerted force like pushing or handgrip. If there is no activity and the upper limb is supported (this is a cycle phase, too), the force related to upper limb posture equals 0. Table presents forces exerted in each cycle phase at the unpacking circuit board workstation.
Table A4 Absolute values of forces accepted for calculating reference force for each type of force at the unpacking circuit board workstation.
Each of the cycle phase should take into account all types of force activity exerted. The root mean square of the sum of squares of component relative forces determines the phase relative force (PRF). Table presents the PRF for each phase of the work cycle for both upper limbs at the unpacking circuit board workstation.
Table A5 Final assessment of upper limb load.
The ULRA method express upper limb load by value of the RTI, which constitutes the result of calculation in accordance with the independent variables characterising the repetitive work: the values of parameters referring to upper limb posture, exerted forces and duration of the specific cycle phases.
ICL and RTI calculated for unpacking circuit board workstation for left and right upper limb are presented in Table A5.