Abstract
In a context of job rotation, this study determined the extent to which the difficulty of a cognitive task (CT) interspersed between bouts of repetitive, low-intensity work (pipetting) influences recovery from fatigue. Fifteen participants performed three experimental sessions, each comprising 10 repeats of a 7 min + 3 min combination of pipetting and CT. The CT was easy, moderate or hard. Surface electromyography (EMG amplitude of the forearm extensor and trapezius muscles) and self-reports was used to assess fatigability. Perceived fatigue and trapezius EMG amplitude increased during sessions. CT difficulty influenced fatigue development only little, besides forearm extensor EMG increasing more in CT3 than in CT1 and CT2. During CT bouts, fatigability recovered, and to a similar extent irrespective of CT. Thus, CT difficulty influenced recovery of perceived as well as performance fatigability to a minor extent, and may not be a critical issue in job rotation comprising alternating physical and cognitive tasks.
Practitioner summary: Alternations between physical and cognitive tasks may be an attractive option for job rotation. In this study on women, we show that the difficulty of the cognitive task influences recovery from fatigue only little and we conclude that cognitive difficulty, within reasonable limits, may be a minor issue in job rotation.
Introduction
Researchers and practitioners in ergonomics generally agree that variation in physical workload is important, as a prerequisite for the worker to recover from periods of intense exposure, and thus prevent musculoskeletal disorders (Mathiassen Citation2006). In Sweden, the work environment act expresses this concern by stating, in the ordinance on Ergonomics (The Swedish Work Environment Authority Citation2012), that the employer shall ensure that work which is repetitive, closely controlled or restricted does not normally occur, and that prevention must lead to increased variation, for instance through job rotation, job enlargement or breaks. Thus, a strategy for obtaining variation is to have the worker do tasks with diverse exposures, but available research does not, so far, allow any explicit conclusions as to which tasks to combine and in which temporal patterns to secure an optimal balance between exposure and recovery (Luger et al. Citation2014; Padula et al. Citation2017; Leider et al. Citation2015).
Introducing rest breaks is the most commonly investigated approach of how to achieve variation and recovery from fatiguing physical tasks (Konz Citation1998). Studies investigating the effects of increased rest allowances or re-organized rest breaks on recovery from fatigue in real occupational settings show, however, ambiguous results (Mathiassen Citation2006; Luger et al. Citation2014). One reason may be that rest allowances beyond a certain duration are not feasible since it would be associated with too large decreases in productivity; another explanation may be that rest breaks do not offer any significant increase in variation in a job that already comprises tasks with low exposures (Mathiassen Citation2006).
An alternative strategy to increase variation could be to introduce mentally demanding tasks in periods between physical work bouts. A questionnaire study by Jahncke et al. (Citation2017), describing the actual and desired numbers of alternations between physical and mental work tasks in selected blue-collar occupations, concluded that such alternations could be a viable option for future interventions aiming at increased variation and better recovery. The workers generally preferred more alternations between physical and mental tasks in their work than they had at present, and in general, they preferred to perform a mental task after a physical task, and vice versa (Jahncke et al. Citation2017).
Thus, a job rotation scheme in a job otherwise dominated by physically demanding tasks could include periods of tasks requiring mental activity so as to obtain sufficient recovery from fatigue caused by the physical task, exemplified by workers at an assembly plant performing administrative work in between bouts of assembly (Christmansson, Fridén, and Sollerman Citation1999). Neurophysiological theories and some empirical evidence even suggest that periods with mental load interspersed between bouts of a physical task can lead to better recovery from fatigue than a passive break (Asmussen and Mazin Citation1978a, Citation1978b; Stock, Beck, and DeFreitas Citation2011). Thus, in a study conducted by Asmussen and Mazin (Citation1978b), participants performed two bouts of repeated elbow or finger flexor contractions until exhaustion, with an interspersed break comprising a cognitive arithmetic task, a diverting physical task, or passive rest. The authors observed that the amount of work that could be carried out after an active break was larger than that after passive rest (Asmussen and Mazin Citation1978b). A more recent study by Stock, Beck, and DeFreitas (2011) reported results in line with this. The participants got either an active or a passive break in between two bouts of repeated, maximal isokinetic knee extensions, and the results showed that initial peak torque had recovered completely after the break with a physical or cognitive activity (a mental arithmetic task) while it had not after the passive break (Stock, Beck, and DeFreitas Citation2011). Since local physiologic responses did not differ between work with and without breaks with a cognitive task, Asmussen and Mazin suggested that the enhanced performance after a cognitive task compared to that after a passive break was mainly due to effects in the central nervous system. This explanation is consistent with later neuroimaging studies showing that cognitive activities can activate the motor and prefrontal cortex in the brain, i.e. regions also known to be involved in fatigue processes (Mandrick et al. Citation2013; Tomporowski Citation2003; Owen et al. Citation2005).
The cited studies by Asmussen and Mazin (Citation1978a, Citation1978b) and by Stock, Beck, and DeFreitas (Citation2011) addressed physical tasks that, in different respects, required the participants to deliver a maximal performance. This may not be particularly relevant in an occupational context. In order to better approach occupational relevance, Mathiassen et al. (Citation2014) used a low-intensity, repetitive physical task involving the upper extremity reflecting tasks that occur frequently in, e.g. industrial settings and are suspected to lead to increased risks for musculoskeletal disorders if performed for prolonged periods of time (Ferguson et al. Citation2013). Mathiassen et al. (Citation2014) investigated if cognitive activities during breaks could accelerate recovery from fatigue. Participants performed six consecutive 10-min bouts consisting of 7 min of a reaching task (moving a 300 g manipulandum between a near and a far location with a cycle time of 2 s) followed by 3 min of a memory task with no notable physical load. This protocol was tested with three different levels of difficulty in the cognitive task. The results suggested that cognitive task difficulty did not influence the recovery of muscle activity (EMG), but that cardiovascular parameters and perceived fatigue were, to some extent, more favourable with the most difficult cognitive task. The authors concluded that cognitive tasks in between bouts of repetitive physical work could accelerate recovery of fatigue to some extent, even though the effects appeared to be moderate.
The study by Mathiassen et al. is, to our knowledge, the only so far to address whether the difficulty of a mental task is important to its effects on recovery (Mathiassen et al. Citation2014). Considering that a variety of mentally demanding tasks may be considered as part of job rotation, this issue deserves more attention in an occupational context. However, while the physical task used in that study was, indeed, submaximal, in contrast to the tasks studied by Asmussen and Mazin (Citation1978a, Citation1978b) and by Stock, Beck, and DeFreitas (Citation2011), it was still of limited ecologic validity as a model of low-intensity repetitive work in occupational life. In addition, previous studies, including that by Mathiassen et al., were performed using male participants and thus did not address how women would respond to alternations. Studies suggest that women use potentially more harmful motor strategies than men when performing repetitive, low-intensity physical tasks (Johansen et al. Citation2013; Srinivasan et al. Citation2016), and so studies specifically on women are motivated.
The present study on women aimed to determine the extent to which the difficulty of a cognitive task interspersed between repeated bouts of a low-intensity repetitive physical work task influences recovery from fatigue caused by the physical task. In the context of the study, fatigue was defined according to Enoka and Duchateau (Citation2016) to be a process encompassing two attributes, performance fatigability and perceived fatigability, where the former refers to a decline in performance over a discrete period of time while the latter refers to changes in the sensation of the performer. Both perceived fatigability and performance fatigability may occur from the very start of physical activity, likely long before any decrement in external force performance, let alone task failure (Enoka Citation2012). Since fatigability includes components related to both performance and perception, it should be assessed by methods addressing both of these attributes. We hypothesise that recovery from fatigue after the physical task – in terms of performance as well as perception – will be more pronounced with a more difficult cognitive task than with a very easy cognitive task.
Methods
Participants
Fifteen healthy women with mean age 26.5 years (SD 4.7), mean stature 1.67 m (SD 0.06), mean body weight 66.4 kg (SD 4.9) and mean BMI 23.7 kg m−2 (SD 1.9) participated in the study. Participants were recruited through announcements on the University campus. Inclusion criteria for participation were; woman, age 20–50 years, right-handed, and some previous experience of pipetting. Exclusion criteria included pregnancy, chronic pain, previous trauma to the neck or back or chronic illness affecting locomotion or the nervous system. Inclusion and exclusion criteria were checked during initial telephone contact. Participants reported to have, on average, 2 weeks (ranging from 1 up to 40 days) of previous pipetting experience, and a majority (9 out of 15) rated their skills to be medium on a 3-grade scale: no, medium, excellent skills. All participants were right-handed, as confirmed by the Edinburgh Handedness Inventory (Oldfield Citation1971). Pipetting proficiency was checked as described below (‘physical task’). All participants signed informed consent and were informed that they could end their participation at any time, without giving any reason. The study was approved by the Regional Ethical Review Board in Uppsala, Sweden (2014/002).
Protocol
Participants visited the laboratory for a one-hour training session (see below) and three experimental sessions, each interspersed by between 3 and 7 days, which was assumed sufficient to minimise possible carry-over effects on fatigue.
For a particular participant (with two exceptions), all three experimental sessions were carried out either in the morning or in the afternoon, according to her preference. Each experimental session consisted of a pre-test battery, a work period comprising 10 consecutive bouts of a 7-minute physical task followed by a 3-minute cognitive task, and a post-test battery (). In total, one experimental session lasted approximately four hours. The pipetting task was equal in all three experimental sessions (‘physical task’ below), while the cognitive task (CT) differed in difficulty (easy (CT1), moderate (CT2), hard (CT3)) between sessions (‘cognitive task’ below). Participants were assigned consecutive participant numbers at enrollment, and the order of CT difficulties was balanced across participants according to these numbers; participant 1 would perform the CTs in the order CT1, CT2, CT3; participant 2 in order CT3, CT1, CT2, participant 3 in the order CT2, CT3, CT1; etc. Participants were not informed in advance about the order of the CT difficulty levels. Participants were instructed to avoid intense physical activity 24 h prior to each experiment and to avoid intake of tobacco products 1 h prior to each experimental session.
Physical task (pipetting)
The physical pipetting task consisted of repeatedly aspiring liquid from a pickup tube (Ø 20 mm) and delivering it to one of four target tubes (Ø 6 mm) in an array of 10 × 10 tubes (), at a cycle time of 2.8 s paced by a metronome. The pace corresponds to 100MTM (Methods-Time Measurement Manyard, Stegemerten, and Schwab Citation1948), thus reflecting a standard work pace in the industry. Led lamps signalled where the liquid was to be dispensed, in a standardised clockwise sequence moving between the four tubes. The work station and pipetting task have been used in previous studies of motor variability and physiological responses in repetitive work (e.g. Srinivasan et al. Citation2015a).
The participants were seated in a rigid chair with a knee angle of 90° and with the table surface height fixed at elbow height, following procedures described in Srinivasan et al. Citation2015a. The chair was fixed in a position being determined by the participant’s wrist being above the pickup tube when her right arm reached out for that tube. Participants were kept from leaning forward by strapping their torso to the chair.
Cognitive task (n-back)
As a standardised CT engaging working memory, we used n-back (Kane et al. Citation2007). One of seven consonants was presented in black on a white computer screen for 2 s, followed by a blank screen for 0.5 s, after which a new consonant was shown. In the ‘easy’ difficulty level (1-back, CT1), the participant was instructed to press a button when the present consonant was identical to the preceding one. The 1-back was used as a control condition, engaging the participant, yet very easy to accomplish. In the moderate (2-back, CT2) and hard (3-back, CT3) levels, the study participant was instructed to press the button if the consonant on the screen matched that presented two or three steps back, respectively. To avoid biomechanical strain on the right body side, the participant pressed the button with her left hand. The performance was measured by the number of correct positive answers, false positive answers (i.e. if the button was pressed when there was, in fact, no match), and response time.
Training session
To minimise learning effects during the actual experiments, participants performed a one-hour training session prior to the three experimental sessions. To demonstrate sufficient pipetting proficiency, the participant had to perform at least 70 cycles of pipetting without mistakes (about half of a 7-min work bout), a criterion also used in previous studies (Srinivasan et al. Citation2015a). All participants eventually met this requirement on skills. Participants also performed a 10-min practice session at each of the three n-back difficulty levels.
Pre- and post-test battery
The pre-test battery included ratings of perceived fatigue and pain, a rest period, a 5-min practicing session of the cognitive task, Reference Voluntary Contractions (RVC), Maximal Voluntary Contractions (MVC), measurements of Pressure Pain Threshold (PPT), and additional ratings of perceived fatigue and pain (). The post-test battery included measurements of MVC and RVC for the right trapezius and the forearm extensors, PPT and ratings of perceived fatigue and pain. Thus, the test battery included measures of perceived fatigability (ratings of fatigue and pain, and PPT) as well as performance fatigability (MVC).
Ratings
Participants rated fatigue using The Swedish Occupational Fatigue Inventory (SOFI) (Åhsberg Citation2000) and the Borg CR-10 scale (Borg Citation1998) (cf. ). SOFI measures five dimensions of perceived fatigue on a seven-grade scale from 0 (not at all) to 6 (to a very high degree), i.e. lack of energy, physical exertion, physical discomfort, lack of motivation, and sleepiness. We used a short version of the original SOFI with only one expression per fatigue dimension, as even applied by Mathiassen et al (Citation2014). On the Borg CR-10 scale (Borg Citation1998), participants rated mental fatigue, fatigue in the right shoulder, pain in the right shoulder, fatigue in right lower arm and pain in the right lower arm. Ratings were expressed verbally and noted by the examiner.
Maximal voluntary contraction (MVC)
For the right shoulder MVC, the participant, sitting with straight torso and arms straight alongside the body, grabbed handles at each side of the chair, which were connected to chains attached to a dynamometer. She then performed three maximal vertical shoulder elevations, each lasting five seconds and interspersed by a 60 s rest break. MVC was measured only for the right body side.
MVC for the right forearm was measured in a maximal handgrip while the participant was seated in a chair with a handgrip force transducer fixed to the table on her right side. Participants performed these handgrips in the same temporal sequence as that used for the shoulder.
Reference voluntary contraction (RVC)
For the shoulder RVC, the participant was instructed to hold both arms straight at 90° abduction in the frontal plane, palms facing down; first two times, 15 s each with 30 s of rest in between, and then once for a full minute.
For the forearm extensors, the RVC consisted in exerting a 15% MVC handgrip, visually guided on a computer screen, with repetitions as in the shoulder procedure.
Pressure pain threshold (PPT)
PPT was measured using an algometer (Somedic Production AB, Sollentuna, Sweden) by which pressure was applied to the right trapezius muscle, halfway between acromion and C7. The pressure was increased from nil by approximately 50 kPa/s and the subject was instructed to press a button as soon as the sensation of pressure turned into pain. PPT was measured three times, and the mean was calculated for further use.
Physiological and psychophysical measurements during the work period
Ratings
During the last minute of each pipetting work bout, and after each CT work bout, the participant verbally rated fatigue in right shoulder, pain in right shoulder, fatigue in right lower arm, pain in right lower arm and perceived mental effort, i.e. variables describing perceived fatigability, on the Borg CR-10 scale (Borg Citation1990, Citation1998). The CR-10 scale, ranging from 0 (nothing at all) to 10 (extremely strong, almost max), has good psychometric properties and is commonly used to measure the intensity of perceived pain, discomfort or fatigue (e.g. Borg Citation1990; Mathiassen Citation1993; Yassierli, Iridiastadi, and Wojcik Citation2007). Perceived mental effort was rated after n-back work bouts only, using the Borg CR-10 scale. The examiner noted the participant’s verbal ratings.
Electromyography (EMG)
EMG was recorded continuously throughout the experiment from the trapezius muscle bilaterally, and from the right forearm extensors, in order to assess the physical workload associated with performing the pipetting task, as well as any possible change in EMG amplitude as a measure of performance fatigability (Hägg, Luttmann, and Jäger Citation2000). For the trapezius muscle, a pair of surface self-adhesive electrodes was placed lateral to the midpoint between vertebrae C7 and acromion with a 20 mm inter-electrode distance, as proposed by Mathiassen, Winkel, and Hägg (Citation1995). For the forearm extensors, an electrode pair was placed with a 20 mm inter-electrode distance on the muscle belly, at 1/3 of the distance between the elbow and the hand (Nordander et al. Citation2004). Before application, the skin area was prepared by shaving, gentle rugging and cleaning with alcohol.
The EMG signal was sampled at 2000 Hz using customary software (Platon version 8.1), amplified (Noraxon MyoSystem 1400 A), and band-pass filtered at 10–1000 Hz. Post-sampling, the data file was imported to Spike (Spike 2, Cambridge Electronics 2015) and visually cleaned from obvious artefacts. Thereafter, the signal was root mean square (RMS) converted in consecutive 250 ms windows. The EMG RMS value was then normalised by the mean EMG amplitude during the RVC in the pre-test battery and expressed in percent of this reference voluntary exertion, i.e. %RVE.
In addition, heart rate and saliva cortisol and alpha-amylase were monitored during the experiment, but these data will be reported elsewhere.
Statistical methods
All variables were described in terms of group means and standard deviations between participants. Differences in initial baseline values (MVC, RVC, PPT, ratings with SOFI and Borg CR-10) between experimental days were evaluated using repeated measures ANOVA with CT (CT1-3) as a within-subject factor.
To determine the effect of CT difficulty on fatigue development across repeated work bouts of pipetting, a set of repeated measures analysis of variance (ANOVA) was carried out. The ANOVA models addressing EMG as dependent variables were constructed using CT (3 levels, CT1–3), timebetween (consecutive pipetting work bouts; 10 levels) and timewithin (consecutive minutes within each pipetting work bout; 7 levels) as within-subject factors, and the order of CT1, CT2 and CT3 across sessions as a between-subjects factor. The models also included the interaction effects CT × timebetween × and timebetween × timewithin. Similar ANOVA models were constructed for ratings of fatigue as dependent variables, but without including the timewithin effect. If the ANOVA models turned out to be significant for the effect of CT and/or the interaction effect of CT × timebetween, a set of post-hoc-tests were carried out to examine individual effects of the three CT.
To evaluate the recovery of EMG during the CT between work bouts of pipetting, the EMG amplitude during the first minute of one pipetting work bout was subtracted from the value during the last minute of the preceding work bout. For ratings of perceived fatigue and pain, the recovery effect was determined by calculating the difference between ratings during pipetting and the following n-back work bout. In the ANOVA-model, CT (three levels), timebetween (9 levels), and the interaction CT × timebetween were then entered as within-subject factors.
To determine the effect of CT on pre-to-post test battery differences in MVC, PPT, ratings of SOFI and Borg CR-10, a set of repeated measures ANOVA was carried out with CT (three levels), time (pre vs post, 2 levels), and the interaction CT × time as within subject factors.
Effect sizes for all variables were expressed as partial eta squared (ηp2).
All statistical analyses were performed in SPSS version 24.0 (IBM SPSS Statistics for Windows, Version 24.0. Armonk, NY: IBM Corp).
Results
Baseline values and exposure in the initial work bout
Pre-test battery
Baseline pre-test measurements of MVC and PPT did not differ between CT1-3 (MVC right shoulder F = 0.18, p = .83; right forearm extensors F = 0.10, p = .38; PPT right trapezius F = 0.01, p = .99). Baseline ratings of perceived fatigue and pain () did not differ between days (fatigue in right shoulder F = 0.98 p = .39; pain in right shoulder F = 0.66, p = .53; fatigue in right lower arm F = 1.90, p = .17; pain in right lower arm F = 1.85, p = .18. SOFI: sleepy F = 1.25, p = .30; exhausted F = 0.93, p = .40; aching F = 1.00, p = .38; breathing heavily F = 0.00, p = 1.00; uninterested F = 0.74, p = .49).
Table 1. Maximal voluntary contraction (MVC), pressure pain threshold (PPT), and perceived fatigue and pain at baseline (pre-test) and after the work period (post-test). Six right-most columns: results of ANOVA with main effects of time (pre vs post) and CT (CT1-CT3), and their interaction (p < .05 marked in bold). Effect sizes are presented as partial eta squared (ηp2).
Initial work bouts of pipetting and n-back
The mean EMG activity during the first minute of the first pipetting bout was 47%RVE (SD 19) and 89%RVE (SD 29) for the right trapezius and the forearm extensors, respectively; with no significant differences between CTs (right trapezius: F = 0.10, p = 1.00; forearm extensors: F = 1.90, p = .17).
Ratings of fatigue and pain during the last minute of the first pipetting work bout, i.e. just before the first CT bout, were tested for differences between CT difficulties. The test confirmed that ratings were, at this point, not related to the upcoming CT; fatigue in right shoulder F = 0.02, p = .98; pain in right shoulder F = 0.55, p = .59; fatigue in lower arm F = 1.17, p = .33; pain in lower arm F = 0.93, p = .41. Ratings of perceived mental fatigue after the first CT work bout did not differ among CT1–3 (F = 1.88, p = .17). Mental effort was, however, affected by CT difficulty (F = 4.39, p = .02), with higher ratings after the initial CT3 bout than after both CT1 and CT2.
During the first CT work bout, participants scored on average 14.0 (SD 0.4) correct positive answers (out of 15 in total) for CT1; 12.8 for CT2 (SD 2.3) and 8.5 for CT3 (SD 2.9). The average number of false positive answers was 0.0 for CT1 (SD 0.0), 0.6 for CT2 (SD 1.6) and 2.4 for CT3 (SD 2.1). The average reaction time was 886 ms (SD 126) for CT1, 1048 ms (SD 146) for CT2 and 1196 ms (SD 157) for CT3. All performance indicators differed significantly between CT1–3 (correct positive F = 28.29, p ≤ .001; false positive F = 14.45, p ≤ .001; reaction time F = 57.78, p ≤ .001).
Exposure and response during repeated bouts of pipetting and n-back
EMG levels in the right trapezius increased within each work bout of pipetting (), while EMG levels in the forearm extensors decreased within work bouts, with a significant timewithin effect and with a partial eta squared, ηp2, of 0.25 and 0.28, respectively (). Cohen (Citation1988) suggested benchmarks for classifying a partial eta squared as small, medium and large, i.e. 0.01, 0.06 and 0.14, respectively. Thus, the effects of timewithin on both trapezius and forearm extensor EMG can be considered ‘large’. EMG levels in the right trapezius increased gradually across the 10 pipetting work bouts (), with a significant main effect of timebetween and a ηp2 of 0.21, i.e. a large effect size (). For the forearm extensors, the timebetween effect was not significant (). CT difficulty did not have any significant main effect on EMG levels (, ). However, a significant interaction effect CT × timebetween was found for forearm extensor EMG (); that is, EMG-activity increased slightly across work bouts during CT3, while the EMG-activity during CT1 and CT2 decreased slightly (). The effect size for the interaction, 0.12, would be graded as medium according to Cohen’s benchmarks. Pairwise post-hoc comparisons revealed a significant CT × timebetween interaction effect for CT2 and CT3 (F = 2.32, p = .02).
Figure 3. EMG activity in right forearm extensors and right trapezius during pipetting work bouts. Mean values across all participants and cognitive task conditions. Full-drawn line, squares: right forearm extensors EMG; dashed line, circles: right trapezius EMG. SD: pooled estimates of standard deviation between subjects within each measurement occasion. The sequence of pipetting and n-back bouts is illustrated at the bottom of the figure.
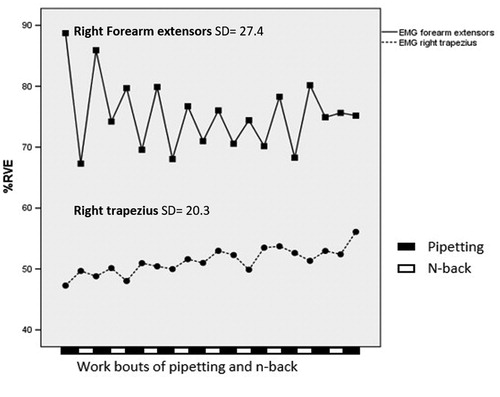
Figure 4. EMG activity in right forearm extensors and right trapezius during pipetting. Top half of figure (squares): right forearm extensor EMG, with each cognitive task protocol (CT1–CT3) illustrated by separate lines. Bottom half of figure (circles): EMG activity in right trapezius, also with CT1–CT3 illustrated by separate lines. SD: pooled estimates of standard deviation between subjects within each measurement occasion.
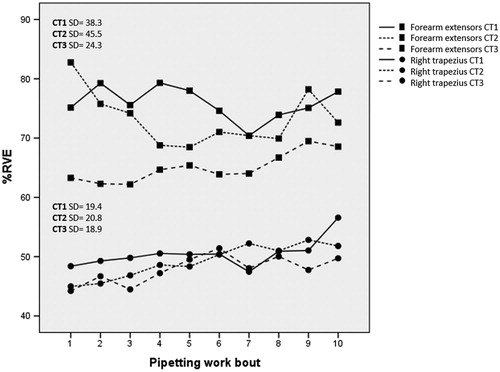
Table 2. Effect of cognitive task difficulty (CT1–CT3) on EMG activity, and perceived fatigue and pain during the work period. The columns show results of ANOVAs, with main effects Timewithin, Timebetween and CT, and their interactions (p < .05 marked in bold). Effect sizes are presented as partial eta squared (ηp2).
Ratings of perceived fatigue and pain in both right shoulder and in lower arm increased throughout the work period, with a significant timebetween effect and large effect sizes, all exceeding 0.40 (, ). Cognitive task difficulty (CT1–3) did not significantly influence ratings of fatigue and pain ().
Figure 5. Perceived fatigue and pain in the right shoulder and lower arm during the last minute of each pipetting bout and after each CT work bout. SD: pooled estimates of standard deviation between subjects within each measurement occasion. The sequence of pipetting and n-back bouts is illustrated at the bottom of the figure.
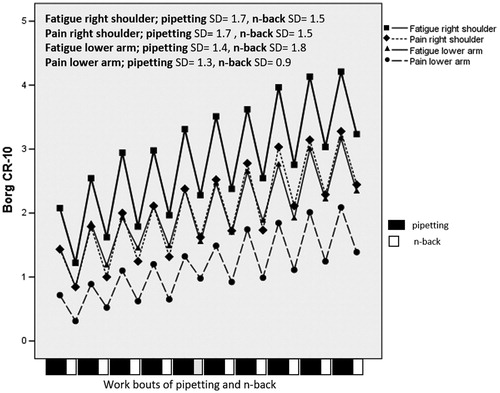
Differences in performance between the three CTs persisted throughout the one-hour work period, with a significant main effect of CT for correct positive as well as for false positive answers, and large effect sizes, i.e. 0.70 and 0.49, respectively (). Performance changed with time (significant main effect of time and large effect sizes; i.e. 0.22 and 0.18 for correct positive and false positive answers), and the change differed significantly between CT difficulties (significant interaction CT × time, with medium to large effect sizes; 0.19 and 0.11 for correct and false positive answers, respectively). Thus, performance declined slightly with time for CT1 and CT2, while it tended to improve for CT3.
Table 3. Cognitive task performance. Columns show means and standard deviations (between subjects) of correct positive and false positive answers for each CT difficulty level, and results of ANOVAs with main effects time and CT, and interaction time × CT. Effect sizes are presented as partial eta squared (partial η2).
Recovery immediately after each pipetting work bout
Recovery in EMG during the CT period between consecutive work bouts of pipetting (assessed as %RVE during the first minute of a work bout subtracted from the last minute in the preceding one) changed over time in the forearm extensors (; F = 4.67, p ≤ .001), but not in right trapezius (F = 0.88, p = .54). Cognitive task difficulty (CT1–3) did not affect recovery; the main effect of CT and the interaction CT × timebetween were both non-significant (right trapezius F = 1.46, p = .25; forearm extensors F = .34, p = .71).
Recovery of perceived fatigue and pain after each pipetting bout (defined as the rating following a CT work bout subtracted from the rating during the last minute of the preceding pipetting work bout) did not change over time (). The main effect of CT on recovery was not significant. However, perceived fatigue in right shoulder showed a significant CT × timebetween interaction effect (F = 2.56, p ≤ .001); during CT1, the recovery effect of the CT period decreased over time, while it increased for CT2 and CT3.
Response after work
All ratings of perceived fatigue (Borg CR10 and SOFI dimensions, except breathing heavily) were significantly higher during the post- than during the pre-test battery (). CT difficulty had no significant effect on differences between pre- and post-test battery results for any of the measured variables except the SOFI-dimension aching, which showed a significant CT × time interaction (). Pairwise comparisons revealed that values were significantly lower after CT2 than after CT3 (F = 4.85, p = .05). MVC increased pre-to-post in the forearm extensors, while no significant effect was found for the right shoulder (see ). Changes in MVC were not significantly influenced by CT difficulty. PPT did not change pre-to-post, and CT difficulty showed no significant effect ().
Discussion
The aim of this experimental study was to determine the extent to which the difficulty of a cognitive task interspersed in between bouts of a repetitive physical task influences recovery from fatigue in women. Based on previous literature, we hypothesised that recovery would be accelerated, i.e. fatigue would increase less over time, with a more difficult cognitive task (CT). In general, however, CT difficulty did not appear to have any strong influence on neither perceived fatigability nor performance fatigability, besides the observation that forearm extensor EMG amplitude was slightly lower, and increased more with time during CT3 than during CT1 and CT2.
Participants in the present study experienced increasing fatigue and pain in right shoulder and right forearm across the entire work period. Both objectively measured performance and perceived effort in the cognitive task differed significantly between the three CTs, indicating that participants did not ‘give in’ during the harder cognitive task (CT3). While perceived fatigability increased clearly with time, indicators of performance fatigability showed ambiguous results. The right trapezius EMG amplitude increased significantly both within and between pipetting work bouts as a sign of performance fatigability (Hägg, Luttmann, and Jäger Citation2000) while MVC, which is a very common metric of performance fatigability, had not decreased significantly after the experiment. However, MVC may not change much after a prolonged, low-force contraction, and may, thus, not be a conclusive measure of performance fatigability in this case (Taylor and Gandevia Citation2008). The observed effect sizes accompanying our results of statistical significance would be classified as medium and large according to Cohen’s benchmarks for evaluating partial eta squared (Cohen Citation1988). While this would corroborate that results are, indeed, of note, we emphasise that estimates of ηp2 should be interpreted with caution, in particular in repeated-measures designs as the present study (Lakens Citation2013).
Thus, we managed to create a physical work task leading to limited performance fatigability, yet definite perceived fatigability, if only to an extent still allowing participants to complete the entire work period with only minor changes in cognitive performance. The observed increase in trapezius EMG amplitude within and between the pipetting bouts corresponded to the development usually interpreted as a sign of performance fatigability, since it suggests an increased central drive to the muscle as a response to reduced force-generating capacity of involved motor units (Hägg, Luttmann, and Jäger Citation2000). Forearm extensor EMG, in contrast, was initially high and decreased thereafter, especially within work bouts. While we have no obvious explanation for this, it may be that the participants adjusted towards a more ‘smooth’ work technique during the course of the bout, or that they re-distributed activity to muscles in the lower arm outside the reach of our EMG recordings. The apparent absence of performance fatigability in the lower arm may be considered a weakness of the pipetting task in the context of the aim of the present study to determine the influence of a cognitive task on development and recovery of fatigue. We recorded surface EMG from single sites on the upper trapezius and forearm extensors and could therefore not assess possible changes in the spatiotemporal distribution of activity, which could also reflect performance fatigability. Such changes have been reported both within and between muscles during sustained activities (Samani et al. Citation2016; Falla and Farina Citation2007). Changes in the EMG frequency spectrum is often used as a sign of changes in muscle properties accompanying performance fatigability (Hägg, Luttmann, and Jäger Citation2000), but we chose not to do any spectral analyses, as guided by studies suggesting that frequency data are difficult to interpret, at least in low-intensity tasks (Hägg Citation1992; Mathiassen Citation1993).
Mathiassen et al. (Citation2014) also found that CT difficulty had a vague influence on recovery; EMG changes in the upper trapezius muscle were similar in protocols with n-back 1, 2, and 3, while recovery of perceived fatigue 1 h post work was slightly more pronounced for n-back 3. Also, heart rate and heart rate variability recovered more with the hardest CT compared to the very easy CT. In contrast to these ambiguous effects of a cognitive task on recovery, both Stock, Beck, and DeFreitas (Citation2011) and Asmussen and Mazin (Citation1978b) showed a substantial acceleration of recovery when mental tasks were performed between repeated bouts of a physical task, compared to inactive rest. Mathiassen et al. (Citation2014) studied a repetitive physical task (repeated movements of a 300 g hand-held manipulandum), which was somewhat more strenuous than the pipetting task in the present study, as indicated by higher ratings of perceived fatigue. Stock, Beck, and DeFreitas (Citation2011) and Asmussen and Mazin (Citation1978b) studied the effects of a mental or diverting physical task interspersed between a set of repeated maximal knee extensions (Stock, Beck, and DeFreitas Citation2011), and between finger flexions performed to exhaustion (Asmussen and Mazin Citation1978b). Both peripheral mechanisms in the exercising muscles and central nervous mechanisms at the supraspinal and spinal level contribute to fatigability (Gandevia Citation2001; Hunter, Duchateau, and Enoka Citation2004). Studies suggest that even though fatigue mechanisms in the central nervous system, CNS, are likely at play during low-intensity, submaximal efforts, their contribution is harder to determine under these conditions than if the subject performs maximally, due to a more complex motor unit recruitment pattern (Taylor and Gandevia Citation2008). Given that the physical tasks used by Asmussen and Mazin (Citation1978b) and Stock, Beck, and DeFreitas (Citation2011) were, indeed, maximal, either in terms of force or endurance, the recovery effect of a mental task might have been larger than in the present study, in that it would elicit a more influential CNS response. We thus argue that the differences in physical and psychophysiological responses between our study and those discussed above are mainly explained by differences in the intensity of the physical task, and thus that the results reported by Asmussen and Mazin (Citation1978b) and Stock, Beck, and DeFreitas (Citation2011) may not extend to repetitive tasks of a comparatively low intensity. Moreover, the duration of the task may have played a role, since the relative involvement of peripheral and central mechanisms of fatigue appears to differ between brief and prolonged submaximal efforts (Taylor and Gandevia Citation2008).
In an occupational perspective, strength of using pipetting as opposed to the tasks used by Asmussen and Mazin (Citation1978b) and Stock, Beck, and DeFreitas (Citation2011) is better ecological validity. Pipetting serves as a valid model of submaximal, repetitive work involving the upper extremity, such as occurring, e.g. in industrial settings (Ferguson et al. Citation2013; Bernard Citation1997; Madeleine, Voigt, and Mathiassen Citation2008). Pipetting has also been used in previous studies of motor variability during repetitive work (Srinivasan, Samani, et al. Citation2015; Sandlund et al. Citation2017).
We wished to use a cognitive task for which performance was easy to track and the difficulty could be controlled at clearly differing levels. Since no deterioration in performance occurred across the ten work bouts for any of the three CT, we suggest that at these levels of difficulty, a cognitive task in between physical work bouts can be carried out with maintained productivity. Unlike simple span tasks, such as recalling a list of numbers or letters, the n-back test requires maintaining information while new information is presented in parallel; thus it reflects both storage and control processes in the working memory (Baddeley Citation1986; Bailey, Dunlosky, and Kane Citation2008). In working life, cognitive tasks may vary greatly in difficulty, and they often engage both storage and processing, such as when performing administrative work. We, therefore, propose the n-back task to be more occupationally relevant than, for instance, a simple span task. The inclusion of n-back 1 as a very easy cognitive condition, with close to perfect performance allowed us to control for attention effects while inducing minimal cognitive load. We, therefore, regard n-back 1 as a control condition in the present experimental design, as an attractive alternative to a simple rest with uncontrolled attention, such as used by Asmussen and Mazin (Citation1978b) and Stock, Beck, and DeFreitas (Citation2011).
The temporal patterns of alternations in this study were designed to allow for a controlled examination of fatigue and recovery. In occupational life, temporal patterns may differ from and be less regular than the 7 + 3 min alternation we used (Jahncke et al. Citation2017). Since the temporal pattern of work with the upper extremity has a profound effect on physiologic and cognitive responses (Mathiassen Citation1993) we recommend further studies to determine the extent to which the temporal pattern of alternations influence fatigue and recovery. Moreover, our experiment was limited to about 2 h of work, and can therefore only inform about short-term effects on fatigue and pain. Inferences regarding the possible effects of alternations between physical and cognitive tasks continuing for whole workdays, and across longer time-spans may be justified only with great caution. In occupational life, additional factors beyond the mere tasks will influence fatigue and recovery during a workday, such as the experience of external stimuli, leadership behaviour and perceived control (Bakker and Demerouti Citation2007). A recent field study describing patterns of alternations between physical and mental tasks in blue-collar occupations reported that workers generally preferred a mental activity after a physical one, and that that they, in general, wished for more alternations than they currently had (Jahncke et al. Citation2017). This point to the need for further research into alternations, using both controlled and observational designs.
The present study was performed on healthy and relatively young women (mean age 26.5, SD 4.7). It is likely that the effects of task difficulty on fatigue depend on various individual factors, and our results might not be readily generalisable to other populations. As one example, both cognitive (Deary et al. Citation2009) and physical (Aoyagi and Shephard Citation1992; Yarasheski Citation2003) performance decrease with age, and participants of older age than those in the present study might have reacted differently to both the physical and the cognitive task. Also, the working memory capacity of an individual will likely influence the cognitive load associated with n-back (Williams, Suchy, and Rau Citation2009), and thus, in theory, the effect that the n-back task could have on central nervous components of fatigue. We, therefore, recommend future studies to address individual factors with a possible influence on the response to alternations.
As mentioned previously, a major rationale for performing this experimental study was to give support for recommendations regarding alternating physical and cognitive tasks in job rotation. Our findings suggest that cognitive tasks with even high demands can be performed successfully between bouts of physical repetitive work without compromising recovery from fatigue or work performance. Thus, with the reservations outlined above as to the portability of these results into occupational practice, we propose that a job rotation including alternations between physical and cognitive tasks appears feasible and that the difficulty of the cognitive task may not be a factor of any major concern.
Conclusion and recommendations
Overall, the results from this controlled experiment showed that both perceived and performance fatigability caused by a repetitive physical task recovered during interspersed periods comprising a cognitive task, but that the difficulty of that task did not have any major influence on the extent of recovery. Thus, in designing a job rotation scheme with alternating mental and physical work tasks, the difficulty level of the cognitive task appears to be of minor importance.
Ethical approval
The study was approved by the Ethical Review Board in Uppsala, Sweden (Dnr. 2014/002).
Abbreviations | ||
EMG | = | Electromyography |
CT | = | Cognitive task |
MVC | = | Maximal Voluntary Contraction |
PPT | = | Pressure Pain Threshold |
PT | = | Physical task |
RMS | = | Root mean square |
RVC | = | Reference Voluntary Contraction |
RVE | = | Reference Voluntary Electrical Activation |
SOFI | = | Swedish Occupational Fatigue Inventory |
Acknowledgements
We are grateful to Nisse Larson and Per Gandal for technical support.
Disclosure statement
No potential conflict of interest was reported by the authors.
Additional information
Funding
References
- Åhsberg, E. 2000. “Dimensions of Fatigue in Different Working Populations.” Scandinavian Journal of Psychology 41 (3): 231–241. doi:10.1111/1467-9450.00192.
- Aoyagi, Y., and R. J. Shephard. 1992. “Aging and Muscle Function.” Sports Medicine 14 (6): 376–396. doi:10.2165/00007256-199214060-00005.
- Asmussen, E., and B. Mazin. 1978a. “A Central Nervous Component In Local Muscular Fatigue.” European Journal of Applied Physiology and Occupational Physiology 38 (1):9–15. doi:10.1007/BF00436748.
- Asmussen, E., and B. Mazin. 1978b. “Recuperation after Muscular Fatigue by ‘Diverting Activities’.” European Journal of Applied Physiology and Occupational Physiology 38 (1): 1–7. doi:10.1007/BF00436747.
- Baddeley, A. 1986. Working Memory. 1st ed. Oxford, UK: Oxford University Press.
- Bailey, H., J. Dunlosky, and M. J. Kane. 2008. “Why Does Working Memory Span Predict Complex Cognition? Testing the Strategy Affordance Hypothesis.” Memory & Cognition 36 (8): 1383–1390. doi:10.3758/MC.36.8.1383.
- Bakker, A., and E. Demerouti. 2007. “The Job Demands-Resources Model: State of the Art.” Journal of Managerial Psychology 22 (3): 309–328. doi:10.1108/02683940710733115.
- Bernard, B. P. 1997. “Musculoskeletal Disorders and Workplace Factors.” NIOSH 97–141, July 1997. https://www.cdc.gov/niosh/docs/97-141/pdfs/97-141.pdf
- Borg, G. 1990. “Psychophysical Scaling with Applications in Physical Work and the Perception of Exertion.” Scandinavian Journal of Work, Environment & Health 16 (1): 55–58. doi:10.5271/sjweh.1815.
- Borg, G. 1998. Borg’s Percieved Extertion and Pain Scales. 1st ed. Champaign, IL: Human Kinetics.
- Christmansson, M., J. Fridén, and C. Sollerman. 1999. “Task Design, Psycho-Social Work Climate and Upper Extremity Pain Disorders - Effects of an Organisational Redesign on Manual Repetitive Assembly Jobs.” Applied Ergonomics 30 (5): 463–472. doi:10.1016/S0003-6870(98)00060-X.
- Cohen, J. 1988. Statistical Power Analysis for the Behavioral Sciences. Second ed. New York, NY: Lawrence Erlbaum Associates.
- Deary, I. J., J. Corley, A. J. Gow, S. E. Harris, L. M. Houlihan, R. E. Marioni, L. Penke, S. B. Rafnsson, and J. M. Starr. 2009. “Age-Associated Cognitive Decline.” British Medical Bulletin 92 (1): 135–152. doi:10.1093/bmb/ldp033.
- Enoka, R. M. 2012. “Muscle Fatigue-from Motor Units to Clinical Symptoms.” Journal of Biomechanics 45 (3): 427–433. doi:10.1016/j.jbiomech.2011.11.047.
- Enoka, R. M., and J. Duchateau. 2016. “Translating Fatigue to Human Performance.” Medicine and Science in Sports and Exercise 48 (11): 2228–2238. doi:10.1249/MSS.0000000000000929.
- Falla, D., and D. Farina. 2007. “Periodic Increases in Force during Sustained Contraction Reduce Fatigue and Facilitate Spatial Redistribution of Trapezius Muscle Activity.” Experimental Brain Research 182 (1): 99–107. doi:10.1007/s00221-007-0974-4.
- Ferguson, S. A., W. G. Allread, P. Le, J. Rose, and W. S. Marras. 2013. “Shoulder Muscle Fatigue During Repetitive Tasks as Measured by Electromyography and Near-Infrared Spectroscopy.” Human Factors: The Journal of the Human Factors and Ergonomics Society 55 (6): 1077–1087. doi:10.1177/0018720813482328.
- Gandevia, S. C. 2001. “Spinal and Supraspinal Factors in Human Muscle Fatigue.” Physiological Reviews 81 (4): 1725–1789. doi:10.1152/physrev.2001.81.4.1725.
- Hägg, G. M. 1992. “Interpretation of EMG Spectral Alterations and Alteration Indexes at Sustained Contraction.” Journal of Applied Physiology (Bethesda, Md.: 1985) 73 (4): 1211–1217. doi:10.1152/jappl.1992.73.4.1211.
- Hägg, G. M., A. Luttmann, and M. Jäger. 2000. “Methodologies for Evaluating Electromyographic Field Data in Ergonomics.” Journal of Electromyography and Kinesiology 10 (5): 301–312. doi:10.1016/S1050-6411(00)00022-5.
- Hunter, S. K., J. Duchateau, and R. M. Enoka. 2004. “Muscle Fatigue and the Mechanisms of Task Failure.” Exercise and Sport Science Reviews 32 (2): 44–49. doi:10.1097/00003677-200404000-00002.
- Jahncke, H., S. Hygge, S. E. Mathiassen, D. Hallman, S. Mixter, and E. Lyskov. 2017. “Variation at Work: Alternations between Physically and Mentally Demanding Tasks in Blue-Collar Occupations.” Ergonomics 0139: 1–10. doi:10.1080/00140139.2017.1282630.
- Johansen, T. I., A. Samani, D. M. Antle, J. N. Côté, and P. Madeleine. 2013. “Gender Effects on the Coordination of Subdivisions of the Trapezius Muscle during a Repetitive Box-Folding Task.” European Journal of Applied Physiology 113 (1): 175–182. doi:10.1007/s00421-012-2425-6.
- Kane, M. J., A. R. Conway, T. K. Miura, and G. J. H. Colflesh. 2007. “Working Memory, Attention Control, and the N-Back Task: A Question of Construct Validity.” Journal of Experimental Psychology. Learning, Memory, and Cognition 33 (3): 615–622. doi:10.1037/0278-7393.33.3.615.
- Konz, S. 1998. “Work/Rest: Part II - The Scientific Basis (Knowledge Base) for the Guide.” Industrial Ergonomics 22 (1–2): 73–99. doi:10.1016/S0169-8141(97)00069-3.
- Lakens, D. 2013. “Calculating and Reporting Effect Sizes to Facilitate Cumulative Science: A Practical Primer for t-Tests and ANOVAs.” Frontiers in Psychology 4: 1–12. doi:10.3389/fpsyg.2013.00863.
- Leider, P. C., J. S. Boschman, M. H. W. Frings-Dresen, and H. F. van der Molen. 2015. “Effects of Job Rotation on Musculoskeletal Complaints and Related Work Exposures: A Systematic Literature Review.” Ergonomics 58 (1): 18–32. doi:10.1080/00140139.2014.961566.
- Luger, T., T. Bosch, D. Veeger, and M. de Looze. 2014. “The Influence of Task Variation on Manifestation of Fatigue Is Ambiguous - A Literature Review.” Ergonomics 57 (2): 162–174. doi:10.1080/00140139.2014.885088.
- Madeleine, P., M. Voigt, and S. E. Mathiassen. 2008. “The Size of Cycle-to-Cycle Variability in Biomechanical Exposure among Butchers Performing a Standardised Cutting Task.” Ergonomics 51 (7): 1078–1095. doi:10.1080/00140130801958659.
- Mandrick, K., G. Derosiere, G. D. Ray, D. Coulon, J. P. Micallef, and S. Perrey. 2013. “Prefrontal Cortex Activity during Motor Tasks with Additional Mental Load Requiring Attentional Demand: A Near-Infrared Spectroscopy Study.” Neuroscience Research 76 (3): 156–162. doi:10.1016/j.neures.2013.04.006.
- Manyard, H., G. Stegemerten, and J. Schwab. 1948. Method-Time-Measurements (MTM). Pennsylvania, USA: Mc Graw- Hill.
- Mathiassen, S. E. 1993. “The Influence of Exercise/Rest Schedule on the Physiological and Psychophysical Response to Isometric Shoulder-Neck Exercise.” European Journal of Applied Physiology and Occupational Physiology 67 (6): 528–539. doi:10.1007/BF00241650.
- Mathiassen, S. E. 2006. “Diversity and Variation in Biomechanical Exposure: What Is It, and Why Would We like to Know?” Applied Ergonomics 37 (4): 419–427. doi:10.1016/j.apergo.2006.04.006.
- Mathiassen, S. E., D. M. Hallman, E. Lyskov, and S. Hygge. 2014. “Can Cognitive Activities during Breaks in Repetitive Manual Work Accelerate Recovery from Fatigue? A Controlled Experiment.” PLoS One 9 (11): e112090. doi:10.1371/journal.pone.0112090.
- Mathiassen, S. E., J. Winkel, and G. M. Hägg. 1995. “Normalization of Surface EMG Amplitude from the Upper Trapezius Muscle in Ergonomic Studies - A Review.” Journal of Electromyography and Kinesiology: Official journal of the International Society of Electrophysiological Kinesiology 5 (4): 197–226. doi:1050-6411(94)00014-X.
- Nordander, C., I. Balogh, S. E. Mathiassen, K. Ohlsson, J. Unge, S. Skerfving, and G.-A. Hansson. 2004. “Precision of Measurements of Physical Workload during Standardised Manual Handling. Part I: Surface Electromyography of m. Trapezius, m. Infraspinatus and the Forearm Extensors.” Journal of Electromyography and Kinesiology 14 (4): 443–454. doi:10.1016/j.jelekin.2003.12.003.
- Oldfield, R. C. 1971. “The Assesment and Analysis of Handedness: The Edinburgh Inventory.” Neuropsychologica 9 (1): 97–113. doi:10.1016/0028-3932(71)90067-4.
- Owen, A. M., K. M. McMillan, A. R. Laird, and E. Bullmore. 2005. “N-Back Working Memory Paradigm: A Meta-Analysis of Normative Functional Neuroimaging Studies.” Human Brain Mapping 25 (1): 46–59. doi:10.1002/hbm.20131.
- Padula, R. S., M. L. C. Comper, E. H. Sparer, and J. T. Dennerlein. 2017. “Job Rotation Designed to Prevent Musculoskeletal Disorders and Control Risk in Manufacturing Industries: A Systematic Review.” Applied Ergonomics 58: 386–397. doi:10.1016/j.apergo.2016.07.018.
- Samani, A., D. Srinivasan, S. E. Mathiassen, and P. Madeleine. 2016. “Variability in Spatio-Temporal Pattern of Trapezius Activity and Coordination of Hand-Arm Muscles during a Sustained Repetitive Dynamic Task.” Experimental Brain Research 235 (2): 1–12. doi:10.1007/s00221-016-4798-y.
- Sandlund, J., D. Srinivasan, M. Heiden, and S. E. Mathiassen. 2017. “Differences in Motor Variability among Individuals Performing a Standardized Short-Cycle Manual Task.” Human Movement Science 51: 17. doi:10.1016/j.humov.2016.10.009.
- Srinivasan, D., S. E. Mathiassen, A. Samani, and P. Madeleine. 2015. “The Combined Influence of Task Accuracy and Pace on Motor Variability in a Standardised Repetitive Precision Task.” Ergonomics 58: 1388–1397. doi:10.1080/00140139.2015.1005174.
- Srinivasan, D., A. Samani, S. E. Mathiassen, and P. Madeleine. 2015. “The Size and Structure of Arm Movement Variability Decreased with Work Pace in a Standardised Repetitive Precision Task.” Ergonomics 58 (1): 128–139. 2014.957736. doi:10.1080/00140139.
- Srinivasan, D., K. E. Sinden, S. E. Mathiassen, and J. N. Cote. 2016. “Gender Differences in Fatigability and Muscle Activity Responses to a Short-Cycle Repetitive Task.” European Journal of Applied Physiology 116 (11–12): 1–9. doi:10.1007/s00421-016-3487-7.
- Stock, M. S., T. W. Beck, and J. M. DeFreitas. 2011. “The Effects of Diverting Activities on Recovery from Fatiguing Concentric Isokinetic Muscle Actions.” Journal of Strength and Conditioning Research 25 (7): 1911–1917. doi:10.1519/JSC.0b013e318220d8dc.
- Taylor, J. L., and S. C. Gandevia. 2008. “A Comparison of Central Aspects of Fatigue in Submaximal and Maximal Voluntary Contractions.” Journal of Applied Physiology 104 (2): 542–550. doi:10.1152/japplphysiol.01053.2007.
- The Swedish Work Environment Authority. 2012. Ergonomics for the Prevention of Musculoskeletal Disorders AFS 2012:2. Stockholm, Sweden: The Swedish Work Environment Authority.
- Tomporowski, P. D. 2003. “Effects of Acute Bouts of Exercise on Cognition.” Acta Psychologica 112 (3): 297–324. doi:10.1016/S0001-6918(02)00134-8.
- Williams, P. G., Y. Suchy, and H. K. Rau. 2009. “Individual Differences in Executive Functioning: Implications for Stress Regulation.” Annals of Behavioral Medicine 37 (2): 126–140. doi:10.1007/s12160-009-9100-0.
- Yarasheski, K. E. 2003. “Review Article: Exercise, Aging, and Muscle Protein Metabolism.” The Journals of Gerontology Series A: Biological Sciences and Medical Sciences 58 (10): 918–922. doi:10.1093/gerona/58.10.M918.
- Yassierli, M. A., N. H. Iridiastadi, and L. A. Wojcik. 2007. “The Influence of Age on Isometric Endurance and Fatigue Is Muscle Dependent: A Study of Shoulder Abduction and Torso Extension.” Ergonomics 50 (1): 26–45. doi:10.1080/00140130600967323.