Abstract
This study quantified the physiological and biomechanical effects of the 20 lb (9.07 kg, males) and 14 lb (6.35 kg, females) weighted vest used in CrossFit, and whether they were predisposed to injury. Twenty subjects (10 males, 10 females) undertook walking (0%, 5% and 10% gradient) and running trials in two randomised study visits (weighted vest/no weighted vest). Physiological demand during walking was increased with the vest at 10% but not 5% or 0% with no change in gait variables. In the running trial, the weighted vest increased oxygen uptake (males; females) (+0.22L/min, p < 0.01; +0.07 L/min, p < 0.05), heart rate (+11bpm, p < 0.01; +11bpm, p < 0.05), carbohydrate oxidation (+0.6 g/min, p < 0.001; +0.2 g/min, p < 0.01), and energy expenditure (+3.8 kJ/min, p < 0.001; +1.5 kJ/min, p < 0.05) whilst blood lactate was increased only in males (+0.6 mmol/L, p < 0.05). There was no change in stride length or frequency. Weighted vest training increases physiological stress and carbohydrate oxidation without affecting measured gait parameters.
Practitioner summary: We examined the effect of weighted vest training prescribed in CrossFit (20 lb/9.07 kg, males and 14 lb/6.35 kg, females) in a randomised controlled trial. We found that physiological stress is increased in both sexes, although three-fold greater in males, but with no change in biomechanical gait that predisposes to lower-limb injury.
1. Introduction
CrossFit® (CrossFit, Inc., Washington, DC, USA) is a fitness regimen and sport, which is characterised by high-intensity functional movements captured in a ‘workout of the day’ or ‘WOD’ (Glassman Citation2007). It includes several high-intensity training activities such as running, weightlifting, and gymnastics, performed in a circuit format with little or no rest, aiming to improve aerobic and anaerobic endurance, speed, flexibility, agility, and motor coordination (Bellar et al. Citation2015; Glassman Citation2007). Indeed a recent systematic review suggests that CrossFit® improves VO2 max, strength, endurance, and lean body mass (Meyer, Morrison, and Zuniga Citation2017). The two hallmarks of CrossFit® are group training and high-intensity interval training, both of which are ranked in the top 3 worldwide fitness trends for 2020 (Thompson Citation2019), suggesting the popularity of CrossFit® will continue.
To augment the beneficial adaptations induced by CrossFit®, many workouts use modified plate carriers (weighted vests) to increase the intensity of the exercise stimulus. The musculoskeletal benefits of weighted resistance training are well documented (Ciolac and Rodrigues-da-Silva Citation2016) and the addition to aerobic exercise or body-weight resistance exercise would be expected to increase physiological demand (Rantalainen, Ruotsalainen, and Virmavirta Citation2012; Macadam, Cronin, and Feser Citation2019). Indeed, weighted vests are associated with an increased training load, evidenced through increased oxygen consumption, increased relative exercise intensity (Puthoff et al. Citation2006), and increased blood lactate accumulation during steady-state exercise (Rusko and Bosco Citation1987). Further, the use of a weighted vest as a training overload stimulus appears to have a beneficial effect on sprinting (Macadam, Cronin, and Feser Citation2019), although caution has been proposed regarding technical execution while wearing the vest (Cross et al., Citation2014). The vast majority of literature having utilised weighted activities has been based on body mass (e.g. 5–40% body mass; (Macadam, Cronin, and Feser Citation2019). The most prevalent weighted vest format for CrossFit® is 20 lb (9.07 kg) for males and 14 lb (6.35 kg) for females and is used for workouts including ‘Murph’, ‘Riley’, and ‘McCluskey’ in CrossFit® workouts (CrossFit Games Citation2019) and the CrossFit® Games (CrossFit Citation2018). All these workouts contain a significant running component with the weighted vest. Despite its popularity, there is no study to have examined the acute physiological stress induced by the 20 lb (males) and 14 lb (females) loads used prevalently in CrossFit® for athletic training and competition.
The use of a weighted vest, however, is likely to create an oxymoron situation. Whilst the physiological benefits of increased training load is desirable and support the use of weighted vests, the increased load carried could lead to increased risk of musculoskeletal injury (Wardle and Greeves Citation2017; Nindl et al. Citation2016). Increased load carriage results in higher ground reaction forces during walking (Gill et al. Citation2021) and running (Lobb et al. Citation2019), with the higher impact affecting most of the ankle (increased load to push at the push-off phase) and the knee (during rebound after foot contact) joints (Huang and Kuo Citation2014) and this impact increases injury risk (Walsh and Low Citation2021). As the knee has been reported as one of the areas commonly injured in CrossFit® training and the only lower-body site (Weisenthal et al. Citation2014; CitationFeito, Burrows, and Tabb 2018), the effects of load as a possible contributor to this statistic warrants investigation. Alteration in step length and cadence have been associated with injury risk during running, with decreased step length (Boyer and Derrick Citation2015) and associated increased cadence (Gerlach et al. Citation2005) reported reducing the risk of running injuries likely via moderation of the increased impact induced by the additional load (Knapik, Harman, and Reynolds Citation1996; Gerlach et al. Citation2005; Gill et al. Citation2021). On the other hand, increases in both step length and cadence parallel to increased load during walking have been reported (Majumdar, Pal, and Majumdar, Sudan Pal, and Majumdar Citation2010). Step length and cadence, thus, were recorded for providing fundamental gait parameters as well as an indication of the injury risk associated with the additional load carriage (Park et al. Citation2015; Schubert, Kempf, and Heiderscheit Citation2014).
Interestingly, the benefits of CrossFit® training appear to be somewhat different between the sexes. Testosterone response was higher in male CrossFit® participants while cortisol and immunological responses were similar between sexes when participants were followed over six months (Poderoso et al. Citation2019). Similarly, the risk of injury is not homogenous between sexes (Weisenthal et al. Citation2014), with females having a greater prevalence of lower-body injury (Sugimoto et al. Citation2020).
Despite the popularity of CrossFit® and the use of the weighted vest in its workouts, to our knowledge, the physiological and biomechanical impact of wearing these specific loads has not been quantified for either sex. It is important that this is investigated in order to inform its use with the potential benefits and risks. The aim of this study, therefore, was to quantify the physiological and biomechanical effects of using the 20 lb weighted vest in males and 14 lb weighted vest in females, prescribed for popular CrossFit® workouts. It was hypothesised that these loads would increase physiological demand, promoting a change in biomechanical gait, which may predispose to injury.
2. Methods
2.1. Subjects
A convenience sample of twenty university-level athletes (10 males, 10 females), aged 19–25 years old were recruited from University sports teams including CrossFit, Athletics, Rugby, and Rowing and gave written informed consent to participate. All participants undertook a minimum of three exercise training sessions per week for at least two years before the start of the study. Subject characteristics are detailed in . Subjects were informed of the benefits and risks of the investigation prior to signing the institutionally approved informed consent document to participate in the study and the study conformed to the seventh revision of the Declaration of Helsinki and ethics approval was granted by the Biomedical & Life Sciences (Lancaster University) Research Ethics Committee.
Table 1. Subject characteristics of male and female participants.
2.2. Procedures
2.2.1. Medical screening and VO2 max test
Subjects attended the Human Performance Laboratory having refrained from exercise or caffeine for 24 h. The initial medical screening was aligned to the American College of Sports Medicine (ACSM) safety to exercise recommendations previously described (American College of Sports Medicine Citation2017). In addition, subjects were excluded if they had a recent history of musculoskeletal injury and if they presented with atrial fibrillation, determined using a personal ECG monitor (KardiaMobile, AliveCor, California, USA). Subjects’ anthropometry was then determined by measuring height (to the nearest 1 cm; 217 Stadiometer, Seca, Hamburg, Germany), body mass (to the nearest 0.1 kg; 799 Seca, Hamburg, Germany) and body composition (Tanita DC-430P, Tanita, Tokyo, Japan) ().
Having successfully passed medical screening, subjects underwent a maximum oxygen uptake (VO2 max) test to volitional exhaustion as previously described (Noakes, Myburgh, and Schall Citation1990). In brief, subjects started running at 10 km h−1 and the speed was increased by 1 km h−1 each minute until exhaustion. The treadmill remained at a 0% gradient throughout the VO2 max test. The VO2 max was defined through obtaining a respiratory exchange ratio (RER) >1.0, a rating of perceived exertion (RPE) of >19, a heart rate within 10 beats of age-predicted max, a blood lactate value of > 8 mmol/L, and a plateau of VO2 uptake (Edvardsen, Hem, and Anderssen Citation2014). All participants met 4/5 of these criteria (RER 1.32 ± 0.02; RPE 19.8 ± 0.1; heart rate 190 ± 2 bpm; blood lactate 10.8 ± 0.7 mmol/L; and plateau of VO2 17 of 20 participants) prior to ending the test.
2.2.2. Experimental visits
Two hours before arriving at the laboratory, subjects consumed a high carbohydrate control meal (306 kcal: 56 g carbohydrates; 6.2 g fat; and 5.2 g protein) typical for pre-competition or training (Rapoport Citation1985). Participants arrived at the laboratory to complete either a weighted vest or no weighted vest condition, with the order randomised (Research randomiser, www.randomizer.org) and participants were unaware of which condition they would be completing before arriving at the lab. Experimental visits were separated by a minimum of 48 h. Participants were fitted with a chest-strap heart-rate monitor (Polar H10, Kempele, Finland) for both experimental conditions. For the weighted vest condition, the weighted vest (5.11 TacTec plate carrier vest, Rogue Fitness, Finland) was also fitted, with the weight plates of 2 × 8.75 lb for males or 2 × 5.75 lb for females (Rogue Cast Weight Vest Plates, Rogue Fitness, Finland) added to the vest to give a total mass of 20 lb for males and 14 lb for females (9.07 kg and 6.35 kg, respectively). The load was distributed equally anteriorly and posteriorly, as plates were fitted in pockets in the front and back of the vest. Participants were then fitted with a mouthpiece (7450 Series V2 Mask and headgear, Hans Rudolph, Shawnee, USA) connected to an online gas analysis system (Metalyzer 3B-R3 Metabolic System, Cortex Biophysik GmbH, Leipzig, Germany).
Using an HP Cosmos treadmill (Pulsar, HP Cosmos, Traunstein, Germany), participants then underwent 20 minutes of walking at 4.9 km h−1; the averaged self-paced walking speed of a healthy person for the age of the participants (Himann et al. Citation1988). The first 10 min were at 0% gradient, with an increase to 5% incline at 10 mins and a further increase to 10% at 15 mins. The increase in gradient was utilised as an additional stressor to enable comparisons and understanding of the effects seen, as gradient can have a larger impact on physiological parameters (Hinde et al. Citation2017), allowing us to distinguish between sex and increased demands effect. Capillary blood samples were taken after each change in inclination gradient and after 20 mins. Participants then undertook 15 mins of passive relaxation where plain water was permitted ad libitum, to be followed by 30 mins running. Immediately before the running trial stage, another further blood sample was taken. Participants then returned to the treadmill and completed 30 mins running at 55% VO2 max at 0% gradient. Pilot work had shown that intensities >60% VO2 max resulted in high subject dropout rates and <45% was deemed not sufficiently (physically) challenging. Blood samples were taken at 15 mins and immediately after the run had finished. RPE (Borg Citation1973) was taken at 5 mins intervals throughout. After the 30 mins run was complete, subjects completed a 10 mins cool down at 4.9 km h−1, before a final blood sample was taken. A schematic of the experimental protocol and the study design is shown in .
Figure 1. Schematic of the study design.
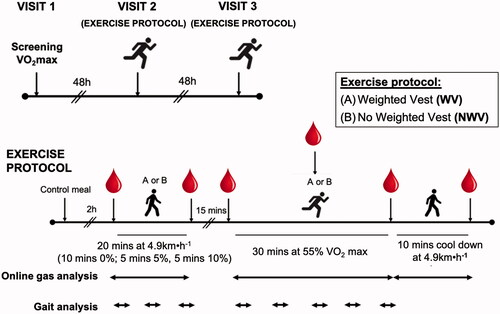
2.2.3. Online gas analysis
Online gas analysis was completed using a Metalyzer 3B-R3 Metabolic System (Cortex Biophysik GmbH, Leipzig, Germany). The online gas analysis system was calibrated with 5% CO2 and 15% O2 (Cranlea, Birmingham, UK). The Metalyzer was connected to the heart rate monitor (Polar H10, Kempele, Finland), to measure heart rate throughout. Data collected included the volumes of oxygen inhaled (VO2) and CO2 exhaled (VCO2), RER, minute ventilation (VE) and breathing frequency. Breath-by-breath data were collected and averaged every 30 s.
2.2.4. Measurement of blood glucose and blood lactate
Capillary blood samples were taken into a 20 ml capillary tube, which was then inserted into a 1,000 ml glucose/lactate hemolyzing solution where it was mixed thoroughly (EKF Diagnostics, Cardiff, UK). The blood was then analysed for glucose and lactate (simultaneously) using a Biosen C-Line Glucose and Lactate analyser (EKF Diagnostics, Cardiff, UK).
2.2.5. Gait analysis
Gait data were collected for 30 s every 5 mins throughout both the walking and running protocols using OptoJump (Microgate SRL, Bolzano, Italy). The OptoJump system is an optical measurement system consisting of two, 1 m-long infra-red photocell bars (with 1 cm intra-photocell spacing) that can derive contact time based on each footfall from the breaking of the transmitted beam. The bars were secured to either side of the treadmill and data were collected at 1000 Hz. Step length (defined as the anteroposterior distance between two opposite heel strikes) and cadence (steps min−1) were recorded as the basic kinematic components of gait (Mercer et al. Citation2002).
2.3. Statistical analysis
All data were checked for normality using a Shapiro-Wilk test and normality was confirmed. Subsequently, physiological variables (including VO2, VE, heart rate, RER, CHO oxidation, energy expenditure, and blood lactate) were analysed by a two-way mixed-model analysis of variance (ANOVA) (2 (no/weight vest condition) x 4-9 (sampling time points during the run)) or (2 (sex) × 4–9 (sampling time points during the run)). When a significant main effect was observed, a Sidak post-hoc test was used to locate differences and correct for multiple comparisons. A third independent variable of time was added with the gait analysis as some evidence suggests that spatiotemporal patterns change during steady-state walking (Simpson, Munro, and Steele Citation2012) or running (Hanley and Mohan Citation2014) whereas the physiological data were not expected to change (Holewijn Citation1990). Gait parameters were missing data points (<20% per group) due to technical failures. To avoid excluding pairwise comparisons and reducing statistical power, the Markov Chain Monte-Carlo method was used (with 20 imputations performed) to replace the missing values. Subsequently, both gait variables were analysed with a 2 (sex) × 2 (no/weight vest condition) × 10 (walk and run time points), followed by univariate analysis and independent (for between-group comparisons) and dependent (for within-group comparisons) t-tests, with a Sidak post-hoc test to correct for multiple comparisons. Data were analysed using GraphPad Prism (GraphPad Prism 8.0, GraphPad Software, Inc.). Data are presented as means ± SEM, and statistical significance was set at p < 0.05.
3. Results
3.1. The weighted vest promoted an increase in physiological demand during walking but only at 10% inclination
There was no significant increase in any physiological measures when wearing a weighted vest and walking at 4.9 km h−1 at 0% incline, except for VO2 in males (). When the incline was increased to 5%, there was no longer a significant increase in VO2 versus no weighted vest, but there was a significant increase in heart rate in males and breathing frequency in females in the weighted vest condition (). Despite these observations of increased exertion, neither 0% nor 5% was associated with any significant increase in blood lactate concentration in either sex. Interestingly, when the incline was increased to 10%, there was an increase in VO2, heart rate, RER, VE, CHO oxidation, and energy expenditure in both sexes. Furthermore, there was an increase in breathing frequency in females but not males (p < 0.05). Collectively, these data show that there is a significant increase in physiological demand with a weighted vest at 10% incline but not at 0%.
Table 2. Comparison of physiological variables across the walking condition.
The between-sex differences in physiological measures largely reflected between-sex differences in normal physiology with no interaction effect of the weighted vest during walking. Indeed, at all inclines, there were significant differences in VO2, VE, and energy expenditure between males and females in both weighted vest and no vest conditions (). At 10% incline, there was a further sex difference in heart rate in the no weighted vest condition (p < 0.05).
3.2. The weighted vest promoted an increase in physiological exertion during the 30-min run
In contrast to the walking data, the addition of a weighted vest promoted both a large and significant shift in physiological demand during running (). Both sexes responded to the addition of the weighted vest with an increase in VO2 consumption (+0.22 L/min in males, p < 0.01; +0.07 L/min in females, p < 0.05) (), an increase in VE/VO2 (7.2% males, p < 0.01; 5.7% females, p < 0.05), and an increase in heart rate (7% males, p < 0.01; 7% females, p < 0.05). There was only a significant increase in VE/VCO2 in males (3.2%, p < 0.05).
Figure 2. Cardiorespiratory responses to weighted vest running. There was an increase in VO2 in the weighted vest condition in males (A, p < 0.001), and females (B, p < 0.01). This was associated with an increase in ventilation in males (C, p < 0.001) and females (D, p < 0.05) and an elevation in heart rate in males (E, p < 0.001) and females (F, p < 0.001). *p < 0.05; **p < 0.01 WV vs. NWV.
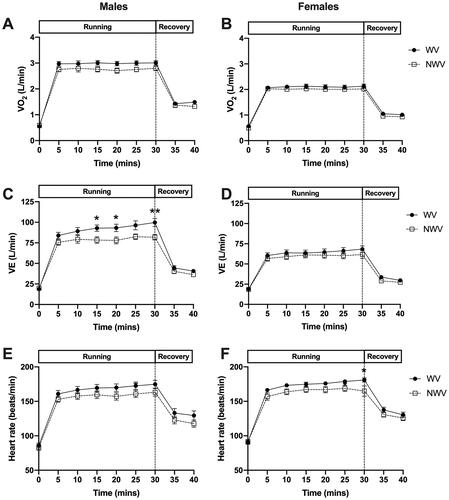
Table 3. Comparison of physiological variables across the 30-minute run.
3.3. The weighted vest promoted a shift in substrate utilisation during the 30-min run that was greater in males than females
These increases in cardiorespiratory demand across sexes were accompanied by a greater shift in substrate utilisation in males () (RER +0.04 in males, p < 0.001 and +0.02 in females, p < 0.05). Indeed, carbohydrate oxidation increased +0.6 g/min (p < 0.001) and fat oxidation was suppressed (–0.18 g/min, p < 0.001) in males whereas in females, carbohydrate oxidation was increased +0.2 g/min (p < 0.01) and fat oxidation was suppressed −0.06 g/min (p < 0.05); only 33% of the shift in substrate utilisation observed in males. This shift towards greater carbohydrate utilisation in males was further accompanied by a significant increase in blood lactate accumulation (+0.6 mmol/L, p < 0.05) that was not observed in females (+0.1 mmol/L, p > 0.05) (). Lastly, the addition of the weighted vest promoted an increase in the rating of perceived exertion in males and females (+3.1 males, p < 0.01; +2.2 females, p < 0.001).
Figure 3. Energy expenditure and substrate utilisation in males and females during weighted vest running. The addition of a weighted vest promoted a significant increase in RER in males (A, p < 0.001) but not females (B, p > 0.05). This was associated with a shift towards greater CHO oxidation in males (C, p < 0.001) and females (D, p < 0.01) and an increase in energy expenditure in males (E, p < 0.001) and females (F, p < 0.05). *p < 0.05; **p < 0.01; ***p < 0.001 WV vs. NWV.
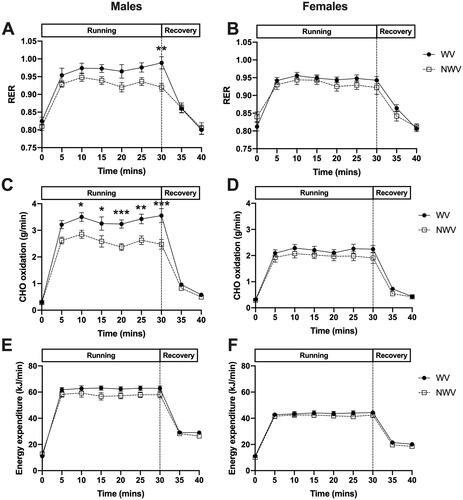
Figure 4. Increased blood lactate levels in the weighted vest condition in males (p < 0.05) but not females (p > 0.05). **p < 0.01 WV vs. NWV.
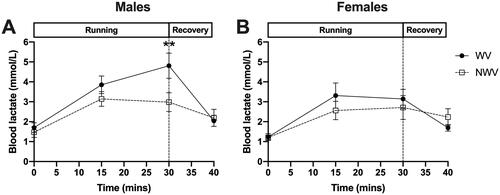
3.4. The addition of a weighted vest did not alter spatiotemporal gait parameters for either sex or locomotion mode
There was no interaction between any of the three factors (sex, load or timepoint – F9,153 = 0.731, p = 0.680, η2 = 0.041), suggesting no statistical significant difference in responses between sexes, and no statistically significant effect on stride length with the addition of the weighted vest. Predictably, there was a statistically significant difference within the time points (F9,153 = 55.0, p = 0.001, η2 = 0.764, with the stride length at all walking time points (no difference between the three walking time points, average 150.3 ± 1.7 cm) smaller than any of the time points during running (no difference between the seven running time points, average 182.4 ± 2.8). The exact same pattern was revealed for cadence, with no interactions (F9,153 = 0.312, p = 0.970, η2 = 0.018) and the only statistically significant difference being within the time points (F9,153 = 535.1, p = 0.001, η2 = 0.969). Cadence at all walking time points (no difference between the three walking time points, average 110.3 ± 1.4 steps/min) was lower than any of the time points during running (no difference between the seven running time points, average 160.8 ± 1.3 steps/min). The results per group and condition can be found in .
Table 4. Comparison of biomechanical variables across the walking and running conditions.
4. Discussion
To the best of the author’s knowledge, this is the first study examining the sex differences in the physiological and biomechanical responses to running in the 20 lb/14 lb weighted vests prescribed in CrossFit. This study shows that the weighted vest does increase physiological demand in both sexes. The increase in energy expenditure with the weighted vest across sexes was similar to that previously shown with approximately 10% body weight vests, although the suppression of fat oxidation was greater in the present study (Purdom et al. Citation2021). The increase in physiological demand with a weighted vest was far greater in males than females. Indeed, the increase in VO2 uptake, heart rate, and energy expenditure was all two to three times greater with the weighted vest in males than females. Furthermore, these changes promoted a shift towards greater carbohydrate oxidation in males and a greater increase in blood lactate, only observed in males. Whilst evidence suggests that lactate is not a cause of fatigue (Robergs, Ghiasvand, and Parker Citation2004), it is nonetheless a good indicator of increased glycolytic metabolism and increased physiological and metabolic stress in males.
Data suggest that physiological and metabolic stress including elevations in VO2, carbohydrate oxidation and energy expenditure were greater in males than females. Whilst both our cohorts were lighter than “elite” CrossFit athletes (77 vs. 84 kg, −8% males, (Bellar et al. Citation2015)) and (64 vs. 68 kg; −6% females (Mangine et al. Citation2020)), they were well-matched for anthropometric and physiological variables including VO2 max previously reported in CrossFit athletes (Kramer et al. Citation2016). In our cohort, the vest was 12 ± 0.5% of male body weight (range: 9.2 to 14.4%) and 10.0 ± 0.3% of female body weight (range: 9.2 to 12.4%) and this may partly explain the increased physiological stress in males. However, even if the vest was worn by an elite male CrossFit athlete of the heaviest body mass found in the literature (approximately 91.5 kg (Mangine et al. Citation2020)), this would still represent only 9.9% of body mass, notably only 0.7% more than in our heaviest participant (9.2%). Moreover, there did not appear a pattern where heavier participants showed a smaller increase in physiological stress and vice-versa, suggesting the interaction between the weighted vest and increase in physiological stress is multi-factorial and not solely based on a linear association between the two. Collectively, these data suggest that the 20 lb weighted vest is a greater physiological challenge for males than the 14 lb vest in females. CrossFit might consider changing the vest weight to make loads more comparable between the sexes.
The authors sought to determine whether this increase in physiological stress was accompanied by a change in gait parameters associated with increased injury risk for either sex.
The lack of any sex effect in the present study agrees with findings from Krupenevich et al. (Citation2015) who reported no differences in stride length between sexes whether loaded or unloaded in a sample with very similar stature to ours (males = 1.79 m, females = 1.71 m), albeit a heavier load (22 kg). When the height difference between the two sexes was increased to 10.5 cm, the step length and cadence were significantly different between sexes for unloaded as well as loaded (9, 17, 29 and 36 kg) trials (Martin and Nelson Citation1986). It appears that a considerable height difference is required before differences in such parameters are presented.
The results in the present study showed no change in step length or cadence with the weighted vest, thus refuting our hypothesis. In a study of carrying different loads (an additional 0% − 27.2% of body mass), neither stride length nor cadence was found to be significantly different between loads (Majumdar, Pal, and Majumdar, Sudan Pal, and Majumdar Citation2010). Our results agree with those findings, as the load in the present study (approximately 12% and 10% of body mass for males and females, respectively) falls within the range examined by Majumdar, Sudan Pal, and Majumdar (Citation2010). A review by Haisman (Citation1988) reported that a ‘safe’ load is one that is less than 1/3 of the body mass. It is, therefore, plausible that the loads above were within ‘tolerance levels’ and, thus, insufficient to cause gait to change, despite the additional physiological stress imposed. In contrast, our results appear to disagree with Gerlach et al. (Citation2005), who reported increased cadence and decreased step length, following a fatiguing protocol to voluntary exhaustion. The protocol in the present study, however, is unlikely to have fatigued the participants to the same level as Gerlach et al. study, as indicated by the physiological data. Indeed, a study by Bampouras and Dewhurst (Citation2016) found that carrying approximately 9.0% of body mass did not alter gait parameters in either older (71 years) or younger (26-year-old) healthy females; it is, thus, plausible that the load used was insufficient to be challenging. Overall, our data suggest that the additional load imposed by the weight vest on CrossFit participants does not alter gait patterns, indicating sufficient ability to absorb the increased ground reaction forces. These findings suggest that walking or running with weighted vests are unlikely to contribute to leg injuries frequently reported in CrossFit (da Costa et al. Citation2019), the cause of which remains elusive.
Although the gait parameters investigated did not change, adaptations to the increased load can not be excluded. Foot strike patterns have been shown to impact selected running kinematics associated with injury (Boyer and Derrick Citation2015). It is possible that individuals utilised a greater ankle plantarflexion motion at contact during the weight vest conditions, landing with a fore/midfoot strike, allowing greater ankle rotation and thus better force absorption (Futrell et al. Citation2020). Although such a change would likely result in altered step length, based on the equipment’s step length calculation, the absence of video analysis to confirm this aspect, is a limitation of the study. In addition, the lack of ground reaction forces or 3D motion analysis to enrich the kinetic and kinematic parameters explored (for example, assessing dynamic stability), prevents us from robust inferences on mechanisms of injury or indeed more mechanistic conclusions about the vest’s effect. Subjects were athletically trained and therefore the physiological and biomechanical responses to wearing a weighted vest may be specific to this population. Moreover, whilst all subjects were familiarised with the weighted vest, some participants used this as a training aid more frequently than others, potentially bringing some bias to the results. Finally, the results must be considered within the context of the methods used. Both walking and running were done on a treadmill with a fixed, determined speed, which will have invariably impacted to some extent cadence and stride length. Given the difference in stride length and cadence between running on a treadmill and running overground at the same velocity (Bailey, Mata, and Mercer Citation2017), running overground could have produced somewhat different results.
Practical applications
This investigation has shown that the addition of weighted vests can increase physiological demand in both sexes without changes in spatiotemporal gait parameters. These data suggest that weighted vest training prescribed in CrossFit (20 lb/9.07 kg, males and 14 lb/6.35 kg, females) can act as an effective training aid to increase overload, without changing biomechanical gait to a pattern associated with a heightened risk of lower-limb injury. This study further suggests that physiological demand is greater in males than females with these training loads. CrossFit training programmes should therefore be tailored to each sex considering the different demands imparted by weighted vests.
Abbreviations | ||
CHO | = | carbohydrate |
ECG | = | electrocardiogram |
NWV | = | no weighted vest |
RER | = | respiratory exchange ratio |
RPE | = | rating of perceived exertion |
VE | = | pulmonary ventilation |
VO2 | = | oxygen consumption |
VCO2 | = | carbon dioxide production VO2 max: maximal oxygen uptake |
VT | = | tidal volume |
WOD | = | workout of the day |
WV | = | weighted vest |
Acknowledgements
The authors would like to thank the study participants, without whom this work would not have been possible.
Disclosure statement
No potential conflict of interest was reported by the author(s).
Additional information
Funding
References
- American College of Sports Medicine. 2017. ACSM’s Guidelines for Exercise Testing and Prescription. Wolters Kluwer, Philadelphia, United States.
- Bailey, Joshua, Tiffany Mata, and John. A. Mercer. 2017. “Is the Relationship between Stride Length, Frequency, and Velocity Influenced by Running on a Treadmill or Overground?” International Journal of Exercise Science 10 (7): 1067–1075.
- Bampouras, Theodoros. M., and Susan Dewhurst. 2016. “Carrying Shopping Bags Does Not Alter Static Postural Stability and Gait Parameters in Healthy Older Females.” Gait & Posture 46: 81–85. doi:https://doi.org/10.1016/j.gaitpost.2016.02.017.
- Bellar, David, Andrew Hatchett, Lawrence. W. Judge, M.E. Breaux, and Lena Marcus. 2015. “The Relationship of Aerobic Capacity, Anaerobic Peak Power and Experience to Performance in CrossFit Exercise.” Biology of Sport 32 (4): 315–320. doi:https://doi.org/10.5604/20831862.1174771.
- Borg, Gunnar. A. 1973. “Perceived Exertion: A Note on ‘History’ and Methods.” Medicine and Science in Sports 5 (2): 90–93.
- Boyer, Elizabeth R., and Timothy. R. Derrick. 2015. “Select Injury-Related Variables Are Affected by Stride Length and Foot Strike Style during Running.” The American Journal of Sports Medicine 43 (9): 2310–2317. doi:https://doi.org/10.1177/0363546515592837.
- Ciolac, Emmanuel G., and Jose M. Rodrigues-da-Silva. 2016. “Resistance Training as a Tool for Preventing and Treating Musculoskeletal Disorders.” Sports Medicine 46 (9): 1239–1248. doi:https://doi.org/10.1007/s40279-016-0507-z.
- Cross, Matt R Matt E Brughelli, and John B Cronin. 2014. “Effects of Vest Loading on Sprint Kinetics and Kinematics.” Journal of Strength and Conditioning Research 28 (7): 1867–1874. doi:https://doi.org/10.1519/JSC.0000000000000354.
- CrossFit. 2018. “CrossFit Games 2017 Regional and Qualifier Events.” Crossfit. Available at: https://games.crossfit.com/workouts/regionals/2017
- CrossFit Games. 2019. “Hero and Tribute Workouts.” CrossFit. Available at: https://www.crossfit.com/heroes
- da Costa, Taline S., Clayder T. N. Louzada, Gustavo K. Miyashita, Paulo H. J. da Silva, Heloisa Y. F. Sungaila, Paulo H. S. Lara, Alberto l. Pochini, Benno Ejnisman, Moises Cohen, and Gustavo G. Arliani. 2019. “CrossFit®: Injury Prevalence and Main Risk Factors.” Clinics 74 (November): e1402. doi:https://doi.org/10.6061/clinics/2019/e1402.
- Edvardsen, Elisabeth, Erlend Hem, and Sigmund A. Anderssen. 2014. “End Criteria for Reaching Maximal Oxygen Uptake Must Be Strict and Adjusted to Sex and Age: A Cross-Sectional Study.” PLoS One. 9 (1): e85276. doi:https://doi.org/10.1371/journal.pone.0085276.
- Feito, Yuri, Evanette K. Burrows, and Loni Philip Tabb. 2018. “A 4-Year Analysis of the Incidence of Injuries among CrossFit-Trained Participants.” Orthopaedic Journal of Sports Medicine 6 (10): 2325967118803100. doi:https://doi.org/10.1177/2325967118803100.
- Futrell, Erin E., K. Douglas Gross, Darcy Reisman, David R. Mullineaux, and Irene S. Davis. 2020. “Transition to Forefoot Strike Reduces Load Rates More Effectively than Altered Cadence.” Journal of Sport and Health Science 9 (3): 248–257. doi:https://doi.org/10.1016/J.JSHS.2019.07.006.
- Gerlach, Kristen E., Scott C. White, Harold W. Burton, Joan M. Dorn, John J. Leddy, and Peter J. Horvath. 2005. “Kinetic Changes with Fatigue and Relationship to Injury in Female Runners.” Medicine and Science in Sports and Exercise 37 (4): 657–663. doi:https://doi.org/10.1249/01.mss.0000158994.29358.71.
- Gill, Niamh, Andrew Roberts, Thomas J. O’Leary, Anmin Liu, Kristen Hollands, Dale Walker, Julie P. Greeves, and Richard Jones. 2021. “Role of Sex and Stature on the Biomechanics of Normal and Loaded Walking: Implications for Injury Risk in the Military.” BMJ Military Health. doi:https://doi.org/10.1136/bmjmilitary-2020-001645.
- Glassman, Greg. 2007. “Understanding CrossFit.” CrossfitJ 56: 1–2.
- Haisman, M.F. 1988. “Determinants of Load Carrying Ability.” Applied Ergonomics 19 (2): 111–121. Elsevier: doi:https://doi.org/10.1016/0003-6870(88)90004-X.
- Hanley, Brian, and Anna K. Mohan. 2014. “Changes in Gait during Constant Pace Treadmill Running.” Journal of Strength and Conditioning Research 28 (5): 1219–1225. doi:https://doi.org/10.1519/JSC.0b013e3182a38796.
- Himann, Joan E., David A. Cunningham, Peter A. Rechnitzer, and Donald H. Paterson. 1988. “Age-Related Changes in Speed of Walking.” Medicine and Science in Sports and Exercise 20 (2): 161–166. doi:https://doi.org/10.1249/00005768-198820020-00010.
- Hinde, Katrina, Ray Lloyd, Chris Low, and Carlton Cooke. 2017. “The Effect of Temperature, Gradient, and Load Carriage on Oxygen Consumption, Posture, and Gait Characteristics.” European Journal of Applied Physiology 117 (3): 417–430. doi:https://doi.org/10.1007/s00421-016-3531-7.
- Holewijn, Michael. 1990. “Physiological Strain Due to Load Carrying.” European Journal of Applied Physiology and Occupational Physiology 61 (3–4): 237–245. doi:https://doi.org/10.1007/BF00357606.
- Huang, Tzu-Wei P., and Arthur D. Kuo. 2014. “Mechanics and Energetics of Load Carriage during Human Walking.” The Journal of Experimental Biology 217 (Pt 4): 605–613. doi:https://doi.org/10.1242/jeb.091587.
- Knapik, Joseph, Everett Harman, and Katy Reynolds. 1996. “Load Carriage Using Packs: A Review of Physiological.” Applied Ergonomics 27 (3): 207–216. doi:https://doi.org/10.1016/0003-6870(96)00013-0.
- Kramer, Samuel J., Daniel A. Baur, Maria T. Spicer, Matthew D. Vukovich, and Michael J. Ormsbee. 2016. “The Effect of Six Days of Dietary Nitrate Supplementation on Performance in Trained CrossFit Athletes.” Journal of the International Society of Sports Nutrition 13 (1): 39. doi:https://doi.org/10.1186/s12970-016-0150-y.
- Krupenevich, Rebecca, Patrick Rider, Zachary Domire, and Paul DeVita. 2015. “Males and Females Respond Similarly to Walking With a Standardized, Heavy Load.” Military Medicine 180 (9): 994–1000. doi:https://doi.org/10.7205/MILMED-D-14-00499.
- Lobb, Nicholas J., AuraLea C. Fain, Kayla D. Seymore, and Tyler N. Brown. 2019. “Sex and Stride Length Impact Leg Stiffness and Ground Reaction Forces When Running with Body Borne Load.” Journal of Biomechanics 86: 96–101. doi:https://doi.org/10.1016/j.jbiomech.2019.01.048.
- Macadam, Paul, John B. Cronin, and Erin H. Feser. 2019. “Acute and Longitudinal Effects of Weighted Vest Training on Sprint-Running Performance: A Systematic Review.” Sports Biomech, 1–16. doi:https://doi.org/10.1080/14763141.2019.1607542.
- Majumdar, Deepti, Madhu Sudan Pal, and Dhurjati Majumdar. 2010. “Effects of Military Load Carriage on Kinematics of Gait.” Ergonomics 53 (6): 782–791. doi:https://doi.org/10.1080/00140131003672015.
- Mangine, Gerald T., Matthew T. Stratton, Christian G. Almeda, Michael D. Roberts, Tiffany A. Esmat, Trisha A. VanDusseldorp, and Yuri Feito. 2020. “Physiological Differences between Advanced CrossFit Athletes, Recreational CrossFit Participants, and Physically-Active Adults.” PLoS One 15 (4): e0223548. doi:https://doi.org/10.1371/journal.pone.0223548.
- Martin, Philip E., and Richard C. Nelson. 1986. “The Effect of Carried Loads on the Walking Patterns of Men and Women.” Ergonomics 29 (10): 1191–1202. doi:https://doi.org/10.1080/00140138608967234.
- Mercer, John A., Jason Vance, Alan Hreljac, and Joseph Hamill. 2002. “Relationship between Shock Attenuation and Stride Length during Running at Different Velocities.” European Journal of Applied Physiology 87 (4–5): 403–408. doi:https://doi.org/10.1007/s00421-002-0646-9.
- Meyer, Jena, Janet Morrison, and Julie Zuniga. 2017. “The Benefits and Risks of CrossFit: A Systematic Review.” Workplace Health & Safety 65 (12): 612–618. doi:https://doi.org/10.1177/2165079916685568.
- Nindl, Bradley C., Bruce H. Jones, Stephanie J. Van Arsdale, Karen Kelly, and William J. Kraemer. 2016. “Operational Physical Performance and Fitness in Military Women: Physiological, Musculoskeletal Injury, and Optimized Physical Training Considerations for Successfully Integrating Women into Combat-Centric Military Occupations.” Military Medicine 181 (1 Suppl): 50–62. Narnia:doi:https://doi.org/10.7205/MILMED-D-15-00382.
- Noakes, Tim. D., Kathryn. H. Myburgh, and Robert Schall. 1990. “Peak Treadmill Running Velocity during the VO2 Max Test Predicts Running Performance.” Journal of Sports Sciences 8 (1): 35–45. doi:https://doi.org/10.1080/02640419008732129.
- Park, Huiju, Seonyoung Kim, Kristen Morris, Melissa Moukperian, Youngjin Moon, and Jeffrey Stull. 2015. “Effect of firefighters’ personal protective equipment on gait.” Applied Ergonomics 48 (May): 42–48. doi:https://doi.org/10.1016/J.APERGO.2014.11.001.
- Poderoso, Rodrigo, Maria Cirilo-Sousa, Adenilson Júnior, Jefferson Novaes, Jeferson Vianna, Marcelo Dias, Luis Leitão, Victor Reis, Nacipe Neto, and José Vilaça-Alves. 2019. “Gender Differences in Chronic Hormonal and Immunological Responses to CrossFit®.” International Journal of Environmental Research and Public Health 16 (14): 2577. doi:https://doi.org/10.3390/ijerph16142577.
- Purdom, Troy M., Christine Mermier, Karol Dokladny, Terence Moriarty, Leigh Lunsford, Nathan Cole, Kelly Johnson, and Len Kravitz. 2021. “Predictors of Fat Oxidation and Caloric Expenditure with and without Weighted Vest Running.” Journal of Strength and Conditioning Research 35 (7): 1865–1872. doi:https://doi.org/10.1519/JSC.0000000000003049.
- Puthoff, Michael L., Benjamin J. Darter, David H. Nielsen, and H John Yack. 2006. “The Effect of Weighted Vest Walking on Metabolic Responses and Ground Reaction Forces.” Medicine and Science in Sports and Exercise 38 (4): 746–752. doi:https://doi.org/10.1249/01.mss.0000210198.79705.19.
- Rantalainen, Timo, Ilona Ruotsalainen, and Mikko Virmavirta. 2012. “Effect of Weighted Vest Suit Worn during Daily Activities on Running Speed, Jumping Power, and Agility in Young Men.” Journal of Strength and Conditioning Research 26 (11): 3030–3035. doi:https://doi.org/10.1519/JSC.0b013e318245c4c6.
- Rapoport, Susan. 1985. “Pre-Compeititon Meals.” National Strength & Conditioning Association Journal 7 (1): 36–37. doi:https://doi.org/10.1519/0744-0049(1985)007<0036:PCM>2.3.CO;2.
- Robergs, Robert A, Farzenah Ghiasvand, and Daryl Parker. 2004. “Biochemistry of Exercise-Induced Metabolic Acidosis.” American Journal of Physiology. Regulatory, Integrative and Comparative Physiology 287 (3): R502–R516. doi:https://doi.org/10.1152/AJPREGU.00114.2004.
- Rusko, Heikki, and Carmelo Bosco. 1987. “Metabolic Response of Endurance Athletes to Training with Added Load.” European Journal of Applied Physiology and Occupational Physiology 56 (4): 412–418. doi:https://doi.org/10.1007/BF00417768.
- Schubert, Amy G., Jenny Kempf, and Bryan C. Heiderscheit. 2014. “Influence of Stride Frequency and Length on Running Mechanics: A Systematic Review.” Sports Health 6 (3): 210–217. doi:https://doi.org/10.1177/1941738113508544.
- Simpson, Katrina M., Bridget J. Munro, and Julie R. Steele. 2012. “Effects of Prolonged Load Carriage on Ground Reaction Forces, Lower Limb Kinematics and Spatio-Temporal Parameters in Female Recreational Hikers.” Ergonomics 55 (3): 316–326. :doi:https://doi.org/10.1080/00140139.2011.642004.
- Sugimoto, Dai, Rebecca L. Zwicker, Bridget J. Quinn, Gregory D. Myer, and Andrea Stracciolini. 2020. “Part II: Comparison of Crossfit-Related Injury Presenting to Sports Medicine Clinic by Sex and Age.” Clinical Journal of Sport Medicine 30 (3): 251–256. doi:https://doi.org/10.1097/JSM.0000000000000812.
- Thompson, Walter R. 2019. “Worldwide Survey of Fitness Trends for 2020.” ACSM'S Health & Fitness Journal 23 (6): 10–18. doi:https://doi.org/10.1249/FIT.0000000000000526.
- Walsh, Gregory S., and Daniel C. Low. 2021. “Military Load Carriage Effects on the Gait of Military Personnel: A Systematic Review.” Applied Ergonomics 93: 103376. doi:https://doi.org/10.1016/J.APERGO.2021.103376.
- Wardle, Sophie L., and Julie P. Greeves. 2017. “Mitigating the Risk of Musculoskeletal Injury: A Systematic Review of the Most Effective Injury Prevention Strategies for Military Personnel.” Journal of Science and Medicine in Sport 20 (Suppl 4): S3–S10. doi:https://doi.org/10.1016/J.JSAMS.2017.09.014.
- Weisenthal, Benjamin M., Christopher A. Beck, Michael D. Maloney, Kenneth E. DeHaven, and Brian D. Giordano. 2014. “Injury Rate and Patterns among CrossFit Athletes.” Orthopaedic Journal of Sports Medicine 2 (4): 232596711453117. doi:https://doi.org/10.1177/2325967114531177.