Abstract
We examined the interactive effects of task load and music tempo on cognition, affect, cardiac response, and safety-relevant behaviour during simulated driving. Using a counterbalanced, within-subjects design, participants (N = 46) were exposed to fast-, slow-, and no-music conditions at high and low loads in a high-grade simulator. Task load had the most salient effect across a broad swath of variables. For core affect, the Load × Music Condition interaction showed that, under high load, affective arousal scores were higher in the fast-tempo condition vs. slow. A main effect of tempo emerged for the HRV index of SDNN, with fast-tempo music eliciting lower scores than both slow- and no-music conditions. Behavioural data showed a main effect of tempo for risk ratings, with fast-tempo music eliciting the highest scores for a traffic-light trigger. Our findings indicate that drivers in high-load, urban environments should exercise caution in their use of fast-tempo music.
Practitioner summary: We examined the interactive effects of task load and music tempo in simulated driving (urban and highway). Cognition, mood, cardiac response, and driving behaviour were assessed. Participants exhibited more risky behaviours in response to fast-tempo music. Drivers should exercise caution in their use of up-tempo music in urban settings.
Introduction
There is a growing acceptance of the importance of driver psychology in the causation of collisions on the road (Brodsky Citation2015; Parnell, Rand, and Plant Citation2020). The cognitions of drivers, their affective states, and behaviour are key determinants of road safety, and thus of high priority in the realm of crash prevention (DfT 2020). An expanding corpus of work has examined how the emotions and physiological states induced by music can affect driving behaviour (Navarro, Osiurak, and Reynaud Citation2018; Navarro et al. Citation2019). Although directly implicated in road collisions (Royal Citation2003; van der Zwaag et al. Citation2013), music also holds potential in terms of heightening drivers’ alertness or reducing their anxiety (Ünal et al. Citation2013a; Wen et al. Citation2019).
There is an imperative to reduce casualty rates, particularly among young adults, and so an enhanced understanding of the effects of various emotional factors that influence performance, such as vehicular music listening, is of high importance (Brodsky Citation2015; Brodsky Citation2018; van der Zwaag et al. Citation2013). It has been reported that ∼90% of drivers listen to music in-car (Brodsky and Kizner Citation2012) meaning that there is, possibly, untapped potential in this auditory stimulus to reduce casualty rates. Understanding the contingencies of music use will enable ergonomists to advise governments, road safety organisations, and motorists on when and how to apply it in a judicious manner (i.e. to optimise affective states and improve driver/road safety; see van der Zwaag et al. Citation2012).
Theoretical background
Fuller’s Driver Control Theory (2011) posits that drivers adjust their behaviour based on their perceived capability to match the demands of the driving situation. Such demands may, in turn, be influenced by perceptual input, personality, the behaviour of other drivers, perceived task difficulty, driving conditions, experience level, and affective/emotional information. Fuller’s theory served as a guiding framework for the present study.
There is substantial driver distraction literature detailing how different types of passive (e.g. music listening) and active (e.g. texting) distractions influence driver responses and decision making (see e.g. Karageorghis et al. Citation2021a, Citation2021b; Regan, Victor, and Lee Citation2013). Several informational properties of music may influence behaviour by consuming attentional resources: The complexity of rhythmical features (e.g. syncopation), excessive sound intensity (i.e. volume; Ünal et al. Citation2013a), fast tempi (Miao et al. Citation2021), and the presence of intelligible lyrics (Ünal, Steg, and Epstude Citation2012). Tempo is a particularly salient feature of music given that, when > ∼120 bpm, this intrinsic quality can elevate activation levels, sharpen attentional focus, and even lead to faster driving speeds (Brodsky Citation2001; Karageorghis et al. Citation2021b; Niu et al. Citation2020). Moreover, fast tempi are likely to upregulate psychomotor arousal (Brodsky Citation2001) and increase mental effort (Ünal, Steg, and Epstude Citation2012).
From an information processing perspective (e.g. Ho and Spence Citation2012), additional input, such as music, could negatively affect performance if the attentional demands exceed the driver’s capacity to respond. For example, in a high-load scenario, such as urban driving, with dense traffic, multiple junctions, and pedestrians, fast or complex music has the propensity to compromise the primary task (i.e. driving). Using a sample of novice drivers and a simulated hazard perception task, Miao et al. (Citation2021) found that the administration of fast-tempo music was associated with increased mental load and reduced hazard perception. If the music has exaggerated bass tones and/or aggressive lyrics, it can promote risky driving behaviours (e.g. undertaking and braking red lights), or even lead to road rage (Anderson, Carnagey, and Eubanks Citation2003; Gulian et al. Citation1989).
Notably, research has shown that driver workload increases with moderate-to-high levels of traffic flow (Teh et al. Citation2014). In a simulator-based study, Törnros and Bolling (Citation2006) demonstrated that urban driving makes greater demands on attentional processing than rural driving. Given that attention and arousal have a reciprocal relationship (see Vuilleumier, Armony, and Dolan Citation2003), slow/sedative music may have a beneficial effect on attentiveness for overloaded drivers (e.g. in densely populated, urban environments; van der Zwaag et al. Citation2013). Conversely, aspects of music, such as fast tempi and complex rhythmic structures were found to increase mental effort (Ünal, Steg, and Epstude Citation2012). Lyrics are particularly distracting, as they require the driver to engage in syntactic processing (see Chien and Chan Citation2015; Karageorghis et al. Citation2021b).
Brodsky and Kizner (Citation2012) designed a sedative music programme that was employed for an in vivo study. When compared to self-selected stimulative music, the sedative programme improved driving performance in terms of safety-relevant behaviours, and had a positive influence on feeling states. These findings were echoed, to a degree, by van der Zwaag et al. (Citation2012) who showed that abrupt changes, from stimulative to sedative music, engendered a mental state of calmness and attendant improvements in driving behaviour. The use of music to achieve a functional level of arousal in different settings is widely acknowledged in the psychomusicological literature (Chanda and Levitin Citation2013; North and Hargreaves Citation2008).
An implication of Fuller’s (Citation2011) model is that in-car music choices will moderate the effects of psychological state (emotion, attention, and arousal) on speed and risk perception, dependent on situational and personal factors. Drivers have the propensity to misperceive either the difficulty of the driving task and/or the distractive effect of music (see e.g. Karageorghis et al. Citation2021a). Empirical evidence suggests that drivers overestimate their driving skills and underestimate the risk of distractions (e.g. Amado et al. Citation2014); particularly so in the case of young, adult males (Fuller Citation2011). A passive form of distraction, such as music, could play an important part in unconscious decision-making processes (McMahon et al. Citation2011). Notably, perception of demands becomes distorted at the extremes of workload (e.g. Desmond, Hancock, and Monette Citation1998), causing drivers to mismatch their effort to the situation in a manner akin to Fuller’s prediction.
Individual differences
Although the primary focus of the present study concerned the interactive effects of music tempo and task load, three salient individual difference factors were included for exploratory purposes; namely, personality, sex, and age. It is probable that the effects of in-vehicle music will be contingent on both the task and driver (Fuller Citation2011). For example, music causes greaterdetriments on cognitive tests in introverts than in extroverts (Furnham and Strbac Citation2002). Individual differences in basal arousal among introverts and extroverts may lead to differential responses to fast/stimulative music (Mistry Citation2015). Matching the music to the driver and the task (i.e. the driving environment) could, conceivably, optimise psychomotor arousal, attentional focus, and driving performance (Brodsky Citation2015). Notably, from among the ‘Big Five’ personality traits, we expected the extroversion dimension to have the most salient effect on how participants responded to music with stimulative and sedative qualities (see e.g. Niu et al. Citation2020). For this reason, we did not wish to further complicate the present analyses by adding the other four traits (i.e. agreeableness, conscientiousness, neuroticism, and openness).
Under the rubric of individual differences come the demographical factors of sex and age—both relevant to road safety and musical responsiveness (Arnett Citation1991; Brodsky and Kizner Citation2012). In terms of the intersection of these factors, it is notable that young, male drivers are overrepresented in road collision statistics (DfT 2020). There are evolutionary antecedents to the reckless on-road behaviour sometimes displayed by this subgroup of the population. High levels of testosterone, sensation seeking, and a desire to gain approval from peers, cross with bass-heavy, aggressive music (e.g. heavy metal) with devastating consequences (Arnett Citation1991; Brodsky and Kizner Citation2012). Accordingly, the present study has an explicit focus on sex (females vs. males) and age group (young vs. middle-aged adults) to further understand the role of these factors. In addition, physiological signals, such as heart rate variability (HRV) have been employed as an index of the autonomic nervous system (ANS) activity in the investigation of affect, attention, and mental effort (Massaro and Pecchia Citation2019). HRV is a non-invasive tool, meaning that it is unlikely to detract from the appreciation of a musical stimulus and that it is suitable for a continuous motor task, such as driving (Riener, Ferscha, and Aly Citation2009). In the present study, we acquired a trio of cardiac measures (i.e. mean HR, SDNN, and RMSSD) to ascertain the association between the subjective ratings of perceived task load and core affect, and physiological responses during simulated driving.
Rationale, aims, and hypotheses
The present study will further understanding of the impact of music in terms of cognition, affective state, cardiac response, and safety-relevant behaviour using two simulated driving tasks. The findings will have implications for other life domains (e.g. surgical procedures, security tasks, and assembly line work) given our predominant focus on the interaction between task load (high vs. low) and the salient musical quality of tempo (fast vs. slow). There is a conspicuous dearth of work that has examined the interaction of task load and music tempo in the ergonomics literature (see Brodsky Citation2015 for a review). Recently, Miao et al. (Citation2021) examined this interaction using a hazard perception test delivered via a PC. They administered one non-lyrical track in each of three experimental conditions (fast-tempo, medium-tempo, and slow-tempo music). The present investigation extends this line of work to a high-grade simulator in which a programme of lyrical music was administered at two music tempi (fast and slow).
We included a range of individual difference factors (i.e. personality, sex, and age), known to be salient in theoretical (Fuller Citation2011) and empirical terms (e.g. Brodsky and Kizner Citation2012; Niu et al. Citation2020) to elucidate their influence on the music–driving relationship. The primary aim of the study was to examine the task load (high/low) by music condition (no music and slow/fast tempo) interaction across a range of cognitive, affective, cardiac, and behavioural variables. A secondary aim was to explore the influence of the individual difference factors of personality (introvert vs. extrovert), sex (females vs. males), and age group (young vs. middle-aged adults) in the aforementioned variables.
It was hypothesised that: a Load × Music Condition interaction for mental effort (measured by the NASA-TLX) would emerge with fast-tempo music demanding greater effort under high load (H1); a main effect of load would emerge, wherein under high load, there would be greater mental effort demanded (H2); the presence of music, regardless of tempo or task load, would elevate affective valence (H3); affective arousal would be optimised by slow-tempo music in the high-load condition and by fast tempo in the low-load condition (H4); there would be a greater amount of risk-taking behaviour (assessed via video and pedal data) with fast-tempo music under the high-load condition (H5). We used the cardiac data (i.e. mean HR, SDNN, and RMSSD) for exploratory and comparatory purposes and, thus tested the null hypothesis (H6). Similarly, we explored three between-subject factors (personality, sex, and age group) across the range of dependent variables under the null hypothesis (H7).
Method
Power analysis
To calculate an appropriate sample size, a power analysis was undertaken using G*Power3 (Faul et al. Citation2009) and the ‘Cohen (Citation1988)’ effect size option. This analysis was predicated on the study’s primary purpose, which was to examine the task load by music condition interaction. Given that an ordinal interaction was hypothesised, wherein the load factor would drive changes in the dependent variables but tempo manipulation would not, the effect size was based on estimation in relation to the effects of task load on driving performance during simulated driving (Ünal, Steg, and Epstude Citation2012). Accordingly, with an effect size of ηp2 = .33 at an alpha level of 0.05, and power at .95, the analysis indicated that 42 participants would be required. Four additional participants were recruited to account for experimental dropout and deletions due to outliers. Note that given the exploratory nature of the analyses pertaining to the aforementioned individual difference factors, these were not predicated on a priori power analyses.
Participants
With written informed consent, a convenience sample of 46 adult volunteers was recruited (23 females and 23 males; Mage = 29.8 years, SD = 2.0 years). Recruitment was conducted through word-of-mouth, promotional flyers, posters, social media posts, and e-mail circulars at Coventry University, UK, and in the surrounding area (i.e. the city of Coventry, UK). Ethical approval was granted by the ethics committees of Brunel University London, UK, and Coventry University, UK. Participants met five inclusion criteria: (a) in the age range 18–55 years; (b) in apparently good health; (c) did not have a tendency to feel sick when playing immersive video games or suffer from motion sickness as a road passenger; (d) no hearing deficiency and/or visual impairment; and (e) held a UK driver’s licence. As mentioned earlier, the between-subjects factors of personality, sex, and age group were explored. The details for each subgroup (n and Mage with SD) are as follows: Personality—nintroverts = 25, nextroverts = 21, Mage introverts = 29.0 years, SD = 8.3 years, Mage extroverts = 30.8 years, SD = 10.0 years; Sex—nfemales = 23, nmales = 23, Mage females = 29.0 years, SD = 9.0 years, Mage males = 30.5 years, SD = 9.2 years; Age Group—nyoung = 24, nmiddle aged = 22, Mage young = 21.7 years, SD = 2.2 years, Mage middle aged = 38.6 years, SD = 3.7 years. In terms of annual mileage and years of driving experience for the two age groups (Myears with SD), the data are as follows: Annual mileage—Myoung = 6,189.5 miles, SD = 5,712.9 miles, Mmiddle aged = 8,700.0 miles, SD = 4,143.5 miles; driving experience—Myoung = 3.8 years, SD = 2.5 years, Mmiddle aged = 17.1 years, SD = 6.4 years.
Music selection procedure
We used a panel representative of the experimental participants to select the music programmes. Previously validated music programmes were not used, as most of the work in the driving–music nexus has been completed outside of the UK (e.g. Beh and Hirst Citation1999; van der Zwaag et al. Citation2013) and thus the music selections would not be entirely appropriate for participants from the Coventry area in the UK. To ensure that the musical stimuli were suitable for each of the two groups of participants (i.e. young and middle-aged adults), a four-stage procedure was used.
First, the research team established participants’ preferred radio stations during driving using an online survey that was administered in advance of their visit to the simulator room. This was to ensure that the music presented would be familiar to participants and liked. Second, the research team listened to published playlists from participants’ preferred radio stations and established 6–7 tracks from each radio station that fell in the tempo range 130–140 bpm (for the fast-tempo condition) and 60–70 bpm (for the slow-tempo condition) that were not highly syncopated, and devoid of explicit lyrics.
Third, the team played 90-s excerpts of the tracks (inc. a verse and chorus) to a music-rating panel that was representative of the intended demographics of the experimental participants (six females and six males, Mage = 41.3 years, SD = 16.1 years), who rated each track using a modified (for urban driving) version of the Brunel Music Rating Inventory-3 (BMRI-3; Karageorghis and Terry Citation2011) and the Affect Grid (Russell, Weiss, and Mendelsohn Citation1989). Fourth, the team evaluated participants’ responses and selected four tracks for each radio station that scored moderately-to-high on the BMRI-3 and placed either in the top-right quadrant of the Affect Grid (i.e. pleasurable and arousing) or the bottom-right quadrant (i.e. pleasurable and relaxing; see in Supplemental Material 1). Three music tracks of the specified tempo and with an unchanging tempo (i.e. without accelerandos or ritardandos) were administered in each experimental condition.
Figure 1. The 2 (Load) × 3 (Music Condition) interaction for core affect (p = .067), which was significant in the affective arousal dimension (p = .013).
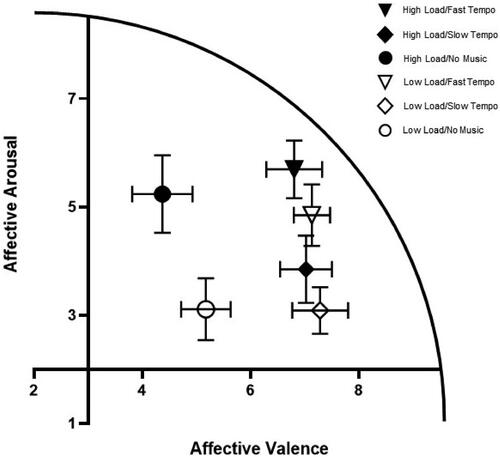
Psychological measures
A range of psychological measures was administered for which we provide a brief outline herein and full details can be found in Supplemental Material 2. To measure personality, we used the 60-item International Personality Item Pool-NEO (IPIP-NEO-60; Maples-Keller et al. Citation2019). To measure core affect immediately after each experimental trial, we administered Russell, Weiss, and Mendelsohn's (Citation1989) Affect Grid (AG). This is a single-item measure used to assess affect along the dimensions of pleasure–displeasure, and arousal–sleepiness. For the assessment of subjective mental workload, we used Hart and Staveland's (Citation1988) NASA Task Load Index (NASA-TLX), which is a 6-item instrument.
Experimental procedures
A few days before visiting the simulator room, participants completed a set of questionnaires online (inc. informed consent, demographic items, idiographic items). Each participant visited the simulator room on one occasion (∼2 h) during which some initial/baseline measures were taken. They were also habituated to the driving simulator for 5 min.
On arrival to the simulator room, participants were offered ginger confectionary (nut free), as this is known to be a mild antidote to motion sickness (Lien et al. Citation2003). Thereafter, the participant was led on a 10-min walk around the campus of Coventry University to ensure they were suitably refreshed before the experimental phase. They were then administered six experimental conditions in a counterbalanced order. Full technical details of the high-grade simulator used can be found in Supplemental Material 3. Participants completed an 8-min urban driving simulation (high load [under three music conditions]) and an 8-min highway driving simulation (low load [under three music conditions]) and each condition was separated by a 5-min break. During the break, a 2-min filler task (a word search) was administered to negate any potential carryover effects. The high-load simulation included four triggers or events, details of which can be found in Supplemental Material 4. By comparison, the low-load condition was characterised by few on-road vehicles, a requirement for relatively gentle manoeuvres (e.g. in overtaking), and long roads with only slight cambers.
Participants were instructed to have adequate sleep the night before testing, avoid alcohol consumption, and refrain from ingesting caffeine on the day of their visit. A within-subjects factorial design was adopted. The first experimental factor measured the effect of mental load (high and low). The second was the music condition, with fast-tempo music (130–140 bpm), slow-tempo music (60–70 bpm), and no music, which served as a control condition in each level of load. Thus, six experimental conditions (high load/fast tempo, high load/slow tempo, high load/no music, low load/fast tempo, low load/slow tempo, low load/no music) were administered. Note that to increase the cognitive demand of the high-load condition (urban simulation) in an ecologically valid way, participants were played a singular traffic announcement in each of the corresponding three trials, which they were required to repeat aloud. Moreover, it is important to highlight that in each experimental condition, only one music tempo was administered.
Conditions were administered in counterbalanced order with each experimental trial lasting ∼8 min. Experimental manipulations coincided with the 8-min driving phase under high and low loads. Participants were asked to maintain a speed of 30 mph in the urban simulation and 70 mph in the highway simulation. Both simulations were populated with traffic in both directions to maintain ecological validity. Moreover, participants were instructed to observe all traffic signals/road signs in accord with the UK Highway Code. The driving simulator was equipped with an automatic transmission system. Accordingly, participants were told not to use the gear lever; only the steering wheel, accelerator pedal, and brake pedal. Participants were also asked to use the rear-view and side-view mirrors, as they would normally.
Heart rate variability
HRV data were recorded throughout each trial. Data were imported into Kubios HRV Standard software (https://www.kubios.com/) and the signal was broken down into four samples, each lasting 5 min. Two time-domain indices were extracted from the cardiac electrical signal. The standard deviation of normal-to-normal RR intervals (SDNN) was used as an index of global activity of the sympathetic–parasympathetic system and the root mean square of successive RR interval differences (RMSSD) was used as an index of parasympathetic activity (Acharya et al. Citation2006). These time-domain indices provide useful objective information regarding the relationship between environmental manipulations and human emotion, which is why they have been used in several related studies (e.g. Ito et al. Citation2017; Karageorghis et al. Citation2021b).
Data analysis
Data were checked for univariate outliers using standardised scores (z > ± 3.29) and for multivariate outliers using the Mahalanobis distance test (p < .001; Tabachnick and Fidell Citation2019). Data were also examined for the parametric assumptions that underlie within-subjects ANOVA (Tabachnick and Fidell Citation2019), other than the behavioural data from video analysis, which were derived from frequency counts. Where the assumption of sphericity was violated, Greenhouse–Geisser-adjusted F tests were used.
Initial analyses, which focussed on the independent variables of primary interest from a theoretical standpoint, employed repeated-measures (RM) 2 (Load) × 3 (Music Condition) (M)ANOVAs for the two psychological measures (i.e. NASA-TLX and Affect Grid) and cardiac measures (HR and HRV indices). Additionally, exploratory analyses were conducted using a mixed-model approach, adopting the between-subject factors of personality (introvert vs. extrovert), sex (females vs. males), and age group (young adults vs. middle-aged adults). Significant F tests were followed up with Bonferroni-adjusted pairwise/multiple comparisons, or in the case of interaction effects, examination of 95% confidence intervals (95% CIs) to identify where differences lay.
Behavioural data were collated for the urban environment (high load) simulation under the following categories: (a) Video data pertaining to risk-related behaviour in response to four triggers (pedestrian, garbage truck, traffic lights, and vehicle cutting); (b) and simulator-derived data from the accelerator and brake pedal positions (i.e. 0 = no pressure applied, 1 = maximum braking); (c) mean speed (mph), and (d) course completion time (min). For the highway environment (low load), simulator data were collated for: (a) accelerator and brake pedal positions; (b) mean speed; and (c) completion time. From among these data, where parametric assumptions were not met and transformations would not serve to normalise the distribution, non-parametric analyses were adopted using rank-based, non-parametric tests. Specifically, the Wald-type statistic and the ANOVA-type statistic were computed within the nparLD package (Noguchi et al. Citation2012) of data analysis software R. In the exploratory analyses, a factorial approach was used, with a series of mixed-model ANOVAs 3 ([Music Condition] × 2 [Personality], 3 [Music Condition] × 2 [Sex], and 3 [Music Condition] × 2 [Age Group]). The main effect of music condition for trigger and pedal data is relevant to the main analysis but in the interests of parsimony, is incorporated within the exploratory factorial analyses.
Results
One univariate outlier was identified and modified to be one unit larger than the next most extreme score in the distribution until the corresponding z-score was < 3.29 (Tabachnick and Fidell Citation2019). No multivariate outliers were identified. Q–Q plots used to examine the distributional properties of interval and ratio data in each cell of each analysis indicated that the data were suitable for parametric analysis.
NASA Task Load Index (NASA-TLX)
The 2 (Load) × 3 (Music Condition) ANOVAs across the six items of NASA-TLX did not show any significant interactions (see ). A main effect of load, associated with large effect sizes, was significant for Mental Demand (ηp2 = .65), Physical Demand (ηp2 = .45), Temporal Demand (ηp2 = .64), Performance (ηp2 = .58), Mental Effort (ηp2 = .58), and Frustration (ηp2 = .52; see ). Higher scores were found for high load compared to low load in all six items (ps < .001). There were also significant main effects of music condition, associated with a large effect for Mental Demand (ηp2 = .16), and a medium effect for Effort (ηp2 = .09; see ).
Table 1. Inferential statistics from within-subjects (M)ANCOVAs.
Core affect
A 2 (Load) × 3 (Music Condition) MANOVA for affective valence and affective arousal showed a marginally non-significant interaction, associated with a large effect (ηp2 = .19; see ). There were significant main effects of load (ηp2 = .45) and music condition (ηp2 = .80), both with a large effect (see ). Step-down F tests were conducted for affective valence and arousal. For the former, there was a main effect of load associated with a medium effect (ηp2 = .12; see ). Scores in the high-load were lower than those in the low-load condition (p = .019). There was also the main effect of music condition with a large effect (ηp2 = .66; see ). Albeit there was no difference in valence scores between fast- and slow-tempo music conditions, there were differences for fast- and slow-tempo music when compared to the no-music control (ps < .001). For arousal, there was a significant two-way interaction associated with a medium effect (ηp2 = .09). For high load, scores were higher in the fast-tempo condition compared to slow music, but there was no difference between fast tempo and no music. For low load, scores for fast-tempo music were higher compared to both slow-tempo and no-music conditions (see ; ).
Table 2. Descriptive statistics across conditions for selected variables.
Cardiac variables
One participant was excluded from the analyses due to equipment failure. Mean HR met the parametric assumptions, however HRV variables (i.e. SDNN and RMSSD) did not, and so non-parametric factorial rank-tests were used (Noguchi et al. Citation2012). The ANOVA-Type Statistic (ATS) is provided in the interests of brevity; suffice to say that in no instance did differing results emerge in the less conservative Wald-Type Statistic.
Mean HR
A 2 (Load) × 3 (Music Condition) ANOVA did not show a significant interaction or main effect of tempo, but a significant main effect of load did emerge, associated with a large effect (ηp2 = .42). Examination of 95% CIs indicated that participants exhibited higher mean HR under high load compared to low load.
Heart rate variability (HRV)
For SDNN, a 2 (Load) × 3 (Music Condition) rank-based factorial analysis showed that there was no interaction, although there was a significant main effect of tempo, associated with a large effect size (ηp2 = .16). Examination of 95% CIs indicated that, for both loads, SDNN in response to fast-tempo music (0.51–0.60) was lower when compared to both slow-tempo (0.43–0.51) and no music (0.43–0.52). For RMSSD, a 2 (Load) × 3 (Music Condition) rank-based factorial analysis did not show an interaction or any main effects (ps > .05).
Exploratory analyses
The aforementioned variables were also investigated with inclusion of the between-subject factors of personality (introvert vs. extrovert), sex (females vs. males) and age group (young vs. middle-aged adults). For the NASA-TLX items, there were significant three-way interactions of Load × Music Condition × Personality associated with large effects for Temporal Demand [F(2, 88) = 3.94, p = .023, ηp2 = .16] and Performance [F(2, 88) = 6.19, p = .003, ηp2 = .24]. There was also a significant two-way interaction of Load × Age Group for Effort, associated with a large effect [F(1, 44) = 8.06, p = .007, ηp2 = .16].
For Temporal Demand, introverted drivers showed higher scores in the slow-music condition under low load, with no differences among music conditions under high load. Conversely, for extroverted drivers, scores were higher in the no-music condition under high load, whereas there was no difference across music conditions under low load (see ). With regard to Performance, under high load, introverted drivers exhibited higher scores in the fast-tempo condition compared to slow tempo and no music (see ). For extroverted drivers, no difference emerged across the three music conditions, under either load. For effort, both young and middle-aged adults exhibited higher scores under high vs. low load. Nonetheless, this effect was stronger for middle-aged adults (see ).
Figure 2. The 2 (Load) × 3 (Music Condition) × 2 (Personality) interaction for the NASA Task Load Index Temporal Demand (a; p = .023) and Performance items (b; p = .003).
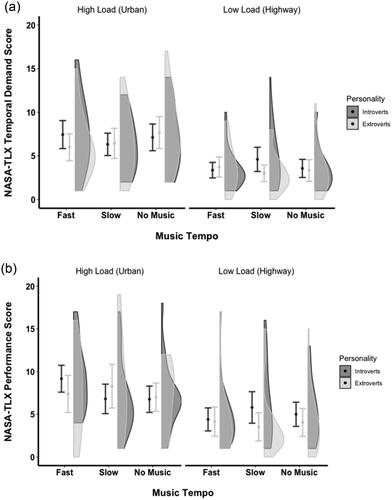
Figure 3. The 2 (Load) × 2 (Age Group) interaction for the NASA Task Load Index Effort item (p = .007).
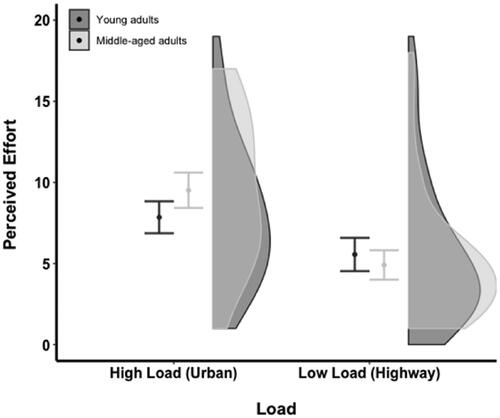
A significant higher-order interaction of Load × Music Condition × Age Group emerged for core affect [Pillai’s Trace = .177, F(4, 176) = 4.28, p = .002, ηp2 = .09], associated with a medium effect size. Step-down F tests show differences both for affective valence and arousal, albeit the pattern of interactive effects differed between these variables. Examination of 95% CIs for valence showed that in the absence of music (i.e. in the control condition) scores were higher under low load vs. high load for young adults, but there was no such difference in middle-aged adults. For arousal, young adults exhibited higher scores in the no-music condition vs. slow-tempo music, but no such difference emerged in the middle-aged group. Middle-aged adults exhibited higher arousal in response to fast-tempo music compared to both slow tempo and no music, whereas no difference emerged between fast tempo and no music in young adults (see Supplemental Material 5; ).
Behavioural data
Risk ratings
The ratings for risk-related behaviour in relation to the four simulator triggers were examined by use of non-parametric Music Condition × Personality, Music Condition × Sex, and Music Condition × Age Group ANOVAs (Noguchi et al. Citation2012). For the pedestrian trigger, there were no significant interactions (p > .05). There were, however, main effects of tempo and sex. For the former, the two music conditions yielded higher risk ratings than control, and for the latter, the males attracted higher risk ratings than females.
For the garbage-truck trigger, the interaction and main effects were non-significant (ps > .05). For the traffic-light trigger, there were only main effects of music condition [F(2, 45) = 3.69, p = .028, ηp2 = .14] and sex [F(1, 45) = 8.19, p = .028, ηp2 = .15]. For the former, the fast-tempo music yielded the highest risk rating when compared to slow tempo and control, and for the latter, the males attracted higher risk ratings than females. For the final trigger, the vehicle cutting, no interactions or main effects emerged (ps > .05).
Pedal data
For the pedestrian trigger, a significant main effect of music condition emerged for mean speed (see ), with higher scores in the control condition compared to the two experimental conditions. A Music Condition × Personality interaction emerged for mean speed in relation to the garbage-truck trigger. Examination of 95% CIs indicated that extroverts recorded higher mean speeds in the fast-tempo condition when compared to introverts.
Cardiac data
Mean HR
A significant Load × Age Group interaction emerged, associated with a medium effect size (ηp2 = .11). Examination of 95% CIs indicated that for young adults, there was an increase of mean HR when driving under high load (0.75–0.78) compared to low load (0.71–0.73), while for middle-aged adults, there was no difference between the two load conditions.
Heart rate variability
A significant Load × Personality interaction emerged for SDNN and was associated with a large effect size (ηp2 = .22). Examination of 95% CIs indicated that for extroverts, there was a decrease in SDNN under the low-load (0.39–0.56) compared to the high-load condition (0.45–0.59). In contrast, for introverts, there was no such difference. There was a significant higher-order Load × Music Condition × Personality interaction for RMSSD, associated with a medium effect (ηp2 = .07). Examination of 95% CIs indicated that for extroverts, under high load, fast-tempo music (0.28–0.50) elicited a decrease in RMSSD compared to slow-tempo music (0.39–0.60) and no music (0.40–0.62). For introverts, no changes emerged in RMSSD, regardless of load or tempo manipulation.
Discussion
The primary purpose of the present study was to investigate the effects of music tempo across high- and low-load driving conditions. A secondary purpose concerned an exploration of the between-subject factors of personality, sex, and age group. Fuller’s Driver Control Theory (2011) served as a guiding framework. The expected Load × Music Condition interaction for the NASA-TLX, with fast-tempo music demanding greater effort under high load, did not emerge, meaning that H1 is not supported (see ). Nonetheless, given the main effect of load, H2 is strongly supported by the present findings.
The hypothesis that the presence of music, regardless of tempo or task load, would elevate affective valence relative to a no-music control (H3) is strongly supported by the present data (see ). The related hypothesis, that affective arousal would be optimised by slow music in the high-load condition and by fast music in the low-load condition (H4), is only partially supported; in the high-load condition, no difference emerged between fast-tempo music and the no-music control (see ).
The hypothesis that more risk-taking behaviour would be evident in the fast-tempo condition under high load (H5) is not accepted. In the case of the Load × Music Condition interaction for cardiac variables, the null hypothesis (H6) was tested and not rejected. For the between-subjects factors of personality, sex, and age group, we also tested the null hypothesis (H7) across the swath of dependent variables and this was not rejected, albeit some minor effects did emerge (e.g. a Load × Music Condition × Age Group interaction for core affect) that will be considered herein. On the whole, the factors of personality and sex had little bearing on the present findings, whereas age group did yield some meaningful findings (see ).
Mental load and music tempo
The two-way interaction of Load × Music Condition that we expected for the NASA-TLX items did not emerge (see ). The strong main effect of load indicated how the driving setting (urban vs. highway) represented a far more salient factor in terms of the requirement for mental effort than did music tempo (Ünal, Steg, and Epstude Citation2012). Ostensibly, participants found that the high load, urban simulation was mentally more demanding and that this demand was not assuaged by the presence of slow-tempo music (see ). When we compare the present findings with those of Miao et al. (Citation2021), it is clear that there are some notable differences. Miao et al. reported higher mental load with fast-tempo music using a hazard perception test but the present findings, using simulated driving, showed no differences between fast- and slow-tempo music conditions (see ).
From the perspective of Fuller’s (Citation2011) Driver Control Theory, the urban environment absorbed attentional capacity, to a degree, and so the soothing (slow-tempo) playlist had no bearing on perceptions of task demand. Examining this interaction from a converse perspective, it is somewhat surprising that the fast-tempo music did not engender greater mental demand under high load; nonetheless, it should be noted that excessive sound intensities, which have higher attentional demands, were not employed in the present study (cf. Brodsky and Slor Citation2013; Mizoguchi and Tsugawa Citation2012).
The six items of the NASA-TLX exhibited a rather similar pattern of findings and, in each case, large effect sizes emerged (see ). The main effect of music condition in the Mental Demand and Effort items illustrated how the no-music control was associated with greater mental demand, whereas the slow-tempo music required the least effort. The finding for Mental Demand is somewhat unexpected and possibly hints at the degree to which the habitual behaviour of listening to music while driving could have impacted the present results (i.e. driving without music is perceived to be somewhat ‘harder’; Dibben and Williamson Citation2007). ‘Habitual behaviour’ can be understood with reference to Fuller’s (Citation2011) notion of ‘poorly calibrated drivers’ (p. 17); such drivers either overestimate their capability or underestimate the task demand. They will typically operate with less spare capacity (e.g. through the use of high-intensity music) and thus visit the boundary at which task demand meets capability more often.
Core affect
The present findings fully support what has long been reported in the psychological literature that, regardless of its tempo, music can enhance affective valence during driving (FakhrHosseini and Jeon Citation2019; Karageorghis et al. Citation2021a; van der Zwaag et al. Citation2013). The affective valence results are among the most compelling of the present study, and confirm how music can modulate this aspect of emotionality. It is worth acknowledging that, regardless of task load, music that is deemed to be enjoyable is likely to absorb greater attentional capacity (e.g. through the driver singing along, tapping on the steering wheel, or with associated imagery; Brodsky Citation2018). The music used in the present study was broadly similar to that used in other experimental studies in terms of genres (i.e. pop, rock, hip-hop; see e.g. Brodsky and Slor Citation2013). It was also broadly comparable to what individuals might choose to listen to while driving (see e.g. Ünal, Steg, and Epstude Citation2012).
In terms of affective arousal, the results did not follow the expected direction, as fast-tempo music did not differ from the no-music control under high load (see ). This finding suggests that slow music is beneficial in terms of optimising arousal under high load, but that under low load, slow music and no music lead to similar affective outcomes. In both high- and low-load conditions, fast-tempo music appears to elicit high levels of affective arousal (see ). Nonetheless, in real-life driving scenarios, such upregulation might be conducive to the maintenance of attention and, by extension, safety-relevant driving behaviour (Ünal et al. Citation2013a, Citation2013b). Notably, the present simulator protocol was only of an 8-min duration (in each condition), thus the demands on drivers for extended maintenance of attention were not as great as those imposed by typically longer real-world drives.
Cardiac variables
Among the cardiac variables, a main effect of load emerged in mean HR. Essentially, the high-load condition elicited higher mean HR, indicating a physiological corollary to the heightened task load (Solhjoo et al. Citation2019). The slow-tempo music did not, however, assuage this elevation in HR during the urban simulation, as might be expected (see ). This may be attributable to the use of a driving simulator, which presents an unfamiliar and possibly anxiety-inducing environment for participants (Zöller, Abendroth, and Bruder Citation2019). The main effect of music condition for SDNN also emerged, showing that slow-tempo music and the no-music control were more effective than fast-tempo music in maintaining greater variability.
Variability is associated with a more positive affective state (Riener, Ferscha, and Aly Citation2009), albeit such an association did not emerge in the present findings. Nonetheless, it is plausible that slow-tempo music might engender an optimal physiological state for driving in a high-load environment (Brodsky Citation2001). In line with Fuller’s (Citation2011) prediction, it is entirely plausible that slow-tempo music would more likely engender an optimal cognitive state to match perceived capability with task demand. If we consider this in light of young drivers, and in particular young male drivers, the observed consequence of a mismatch is an unduly high frequency of collisions and near misses on the road (DfT 2020). It is conceivable that the promotion of more calming music among young males would likely reduce the frequency of such incidents (Brodsky and Kizner Citation2012).
Exploratory analyses
We tested the null hypothesis across a series of exploratory analyses that included the between-subject factors of personality, sex, and age group. For the most part, these independent variables did not yield significant effects, in support of our hypothesis, but there were some notable exceptions. For example, a higher-order Load × Music Condition × Age Group interaction was evident for core affect (see Supplemental Figure 2S). The finding that young adults reported higher arousal scores under high load with no music, is indicative of how germane music listening is to their regular driving experiences (Brodsky and Kizner Citation2012). It would seem that slow-tempo music has a mentally calming effect for young and middle-aged adults alike, but the implications for road safety could be more profound for the young (DfT 2020; Fuller Citation2011). For short-duration urban drives, in particular, music that is calming in nature and of a slow-to-medium tempo (i.e. < 110 bpm) is likely to engender benefits for young drivers in terms of road safety (Brodsky Citation2001; Brodsky and Kizner Citation2012).
Task load
A higher-order Load × Music Condition × Personality interaction emerged for the NASA-TLX items of Temporal Demand and Performance. It is interesting that extroverts, who purportedly have lower visceral stimulation than introverts (Eysenck Citation1994), reported higher Temporal Demand in the slow-tempo condition under low load (see ). This would suggest that when driving on the open road, extroverts might well derive benefit from the presence of mildly stimulating music (Furnham and Strbac Citation2002). Extroverts also benefit from the presence of music in high load, urban environments, albeit the tempo does not bear influence on their perception of Temporal Demand (see ).
For introverts, there was a difference between the fast-tempo condition and remaining conditions in the NASA-TLX Performance item, but no such difference emerged for extroverts (see ). This difference was evident only in the high-load condition. A plausible explanation for this seemingly anomalous finding concerns the fact that introverts are known to express a preference for music that might compensate for aspects of their personality (North and Hargreaves Citation2008). In this instance, the fast-tempo music—with its greater rhythmic accentuation—may have held greater arousal potential when compared to the rhythmically simpler, slow-tempo condition. This effect is unlikely to be random given its magnitude (ηp2 = .24).
The two-way interaction of Load × Age Group for the effort perception item of the NASA-TLX, suggests greater discrimination in terms of effort perception under high and low loads for middle-aged adults when compared to their younger counterparts. The interaction is driven by a clear dip in perceived effort in the slow-tempo condition for young adults (see ). It is plausible that the effort ratings of middle-aged adults were subject to a ceiling effect under high load but not so for young adults. This left greater scope for the experimental manipulation to influence the effort ratings of young adults.
In concert with Fuller’s (Citation2011) Driver Control Theory, due to their relative inexperience, young drivers exhibit a tendency to devote insufficient attention to the demands of the road. They perceive, however, that their attention is adequate. Indeed, it may be adequate until the occurrence of an unexpected event. For example, a pedestrian walks in front of them and they have a reduced ability to avoid the collision. Accordingly, although drivers can cope with even loud and aggressive music during normal driving, it is the response to a sudden peak in attentional demand that is affected by music (cf. Young et al. Citation2008).
Cardiac data
Analysis of the Load × Age Group interaction showed that, in young adults, there was an increase of mean HR under high load compared to low load. There was no difference in middle-aged adults. These results may be explained by a possible ceiling effect as the middle-aged had higher mean HR in both load conditions (see ). The Load × Personality interaction showed a decrease in SDNN under the low-load compared to the high-load condition for extroverts, while for introverts there was no SDNN difference across the two loads. This could be attributed to the extroverts not receiving sufficient mental stimulation from the low-load condition (Depue Citation2006). Although there is limited literature that links personality traits with HRV, it has been found that personality traits are related to autonomic balance (Zohar, Cloninger, and McCraty Citation2013). This is clearly an area that warrants further investigation.
As for the Load × Music Condition × Personality interaction, extroverts exhibited lower RMSSD for fast-tempo music under the high-load condition. There was no difference for introverts regardless of load or tempo. RMSSD is an index of parasympathetic activity and there is evidence to suggest that stimulating music decreases activation of the parasympathetic nervous system (i.e. there is an increase in respiration and heart rate; Nakahara et al. Citation2009). Accordingly, it appears that the combination of high load with fast-tempo music influenced parasympathetic activity only in extroverts, who apparently have greater sensitivity in their dopamine reward network than introverts (Depue and Fu Citation2013). The dopamine network of introverts is less sensitive to stimulating music, which accounts for their lack of psychophysiological response under high load. Based on this finding, a clear implication for extroverted drivers is that they might seek to avoid highly stimulating music in demanding urban environments. This would facilitate the regulation of their physiological arousal in a manner that is conducive to road safety.
Behavioural data
Analyses of the four simulation triggers showed that where differences emerged, fast-tempo music elicited greater risk-taking behaviour, and males exhibited greater risk-taking behaviour than females. Both of these findings are consistent with those of past empirical studies that have examined tempo and sex effects during driving (Brodsky Citation2001; Konz and McDougal Citation1968; Li, Chen, and Zhang Citation2019), although the measures of driving behaviour were different (i.e. longitudinal control rather than the brake pedal use of the present study).
It appeared throughout the present findings that personality bore no influence on risk-taking behaviour, although extroverted females and males tended to be more similar than their introverted counterparts. This result is not congruent with a recent finding showing that introverts drove at a slower speed and attracted lower risk ratings under slow-tempo music when compared to extroverts (Karageorghis et al. Citation2021b). The personality difference in risk-taking emerged when participants self-selected music, rather than when administered a predetermined playlist, as in the present study. This suggests that personality effects might be sensitive to the agency over music selection, which might account for the difference between the two studies.
A Music Condition × Personality interaction for mean speed (see ) suggests that extroverts listening to fast-tempo music drove faster when negotiating the garbage-truck trigger. Accordingly, the stimulation from their environment that extroverts are known to seek (Depue Citation2006) can lead to more risky behaviour when fast music is combined with driving. It should be emphasised, however, that among the present findings, the influence of personality on driving behaviour is far from compelling (Oltedal and Rundmo Citation2006). This aspect of our findings does not serve to support Fuller’s (Citation2011) model, given that it highlights personality as an important individual difference variable in the interplay between risk feeling and task difficulty. There is clearly scope for further empirical research to address the interplay of music exposure and personality type in terms of safety-relevant driving behaviour.
An anomalous finding to emerge that warrants brief mention is that of the main effect of tempo in relation to mean speed and the pedestrian trigger (see ). The speed was highest in the no-music control, suggesting that the two music conditions led to greater attention being apportioned to the safe passage of the pedestrian. We might speculate that because participants could not be blinded to the presence of the music, they took extra care in its presence in some instances. This would not, however, be generalisable to real-world driving settings, where there is ample evidence to suggest that the presence of fast music can lead to speeding (Brodsky Citation2001; Cassidy and MacDonald Citation2010).
Strengths and limitations
The present simulation study centred on an examination of the task demand of attentional load and the musical quality of tempo. Albeit load and tempo were the variables of primary interest, we ran additional analyses to explore potentially salient variables of personality, sex, and age group. With the ecological validity of the simulation task in mind, we included four random triggers (see Supplemental Material 4) and the environment was populated with traffic in both directions. A robust design was adopted with six conditions administered over a 2-h period with breaks and a filler task (i.e. the word search). The analyses were extensive and prefaced by a thorough data diagnostic process (see Tabachnick and Fidell Citation2019). Notably, we used factorial non-parametric statistics (rank-based ANOVAs) for data that did not meet the relevant parametric assumptions, so as not to inflate alpha.
Before testing, a rigorous procedure involving four stages was employed to render the two music conditions suitable for participants. In particular, we carefully considered differences in musical predilections between young and middle-aged adults (Bonneville-Roussy and Rust Citation2018). Accordingly, the present study exhibited considerable sensitivity to the cultural milieu and music preferences, without undermining internal validity through requesting participants to engage in the simulation tasks with self-selected music (e.g. Cassidy and MacDonald Citation2010; Ünal, Steg, and Epstude Citation2012). The use of self-selected music carries the danger of introducing a broad range of psycho-acoustic properties in each music programme (see Karageorghis et al. Citation2021a). Moreover, the study combined a range of subjective and objective outcome measures to facilitate an understanding of how driving load and music tempo might impact safety-relevant cognitions and behaviours.
The study was not, however, without some limitations. Perhaps, most notably, given that only two tempo conditions were administered, with these being fairly disparate (i.e. fast and slow), it might not represent how music is typically used on the road (i.e. shuffled within a broad range of tempi; Brodsky Citation2001; Ünal, Steg, and Epstude Citation2012). The external validity of the high- and low-load stimulations was not formally established in the present study but rather based on logical assumptions regarding the tasks/stimuli that would differentiate load. Differences in key outcome measures (e.g. core affect and cardiac variables) were not assessed between simulated and real-life environments to gauge how using a simulation might have threatened ecological validity (Zöller, Abendroth, and Bruder Citation2019).
Only one sound intensity was used (70 dBA), as the tempo was not crossed with volume (see e.g. Dalton, Behm, and Kibele Citation2008); thus, we could not examine the interactive effects of tempo and volume in addition to the interactive effects of tempo and load. It seems from the human factors literature that the combination of high tempo with high volume could have deleterious consequences for driver and road-user safety (Mizoguchi and Tsugawa Citation2012). Nonetheless, the present design was already rather complex and so a programmatic approach might be taken in further examining the various independent variables that were explored.
Conclusions
The present results reinforce previous findings in elucidating the potential benefits of music for drivers’ affective state, regardless of its tempo and the mental load imposed by driving conditions. It appears that under high-load conditions, particularly when a driver feels stressed or under the pressure of time, slow-tempo music is likely to engender an affective state that is most conducive to safety-relevant behaviour. This is in accord with the predictions of Fuller’s (Citation2011) Driver Control Theory. Interestingly, a Load × Music Condition interaction did not emerge for mental load-related outcomes, suggesting that the strong effect of load overrode any possible interaction. Perhaps more subtle manipulations of load in future studies might serve to bring the effects of music tempo (i.e. auditory stimulation) to the fore.
A series of exploratory analyses involving the between-subjects factors of personality, sex, and age group were computed. There was also a three-way interaction of Load × Music Condition × Personality for two of the NASA-TLX items. This showed that extroverts reported higher scores for Temporal Demand in the slow-tempo condition under low load and that they benefitted from the presence of music under high load. For introverts, there was a difference in perceived performance between fast-tempo music and both other conditions under high load. A three-way interaction of Load × Music Condition × Age Group showed that middle-aged adults exhibited higher arousal in response to fast-tempo music compared to both slow tempo and no music. No such difference emerged between fast-tempo music and no music in young adults, suggesting middle-aged adults’ greater sensitivity to the music.
The originality in this study stems from the simultaneous examination of relevant factors in the music–driving nexus that further our understanding of how auditory stimuli can influence safety-relevant cognitions and behaviours under two contrasting driving conditions (urban and highway). There are practical implications that stem from the present findings in terms of extroverts appearing to derive some benefit from the stimulation afforded by fast-tempo music and middle-aged adults requiring greater mental effort to process music—regardless of its tempo—while driving in both simulation scenarios. Extroverts need to be wary, however, of predisposing themselves to excessive levels of physiological arousal when listening to fast-tempo music in urban driving environments. It would seem that there is further need in this literature for studies to examine the interactive effects of music tempo and intensity (see e.g. Navarro, Osiurak, and Reynaud Citation2018; Niu et al. Citation2020), particularly under contrasting mental loads. Such work would shine a light on the potential dangers of combining high levels of music tempo and intensity in a driving context.
Abbreviations | ||
HRV | = | heart rate variability |
SDNN | = | standard deviation of normal-to-normal RR intervals |
DfT | = | Department for Transport |
ANS | = | autonomic nervous system |
RMSSD | = | root mean square of successive RR interval differences |
PC | = | personal computer |
HR | = | heart rate |
ηp2 | = | partial eta squared effect size |
M | = | mean |
SD | = | standard deviation |
UK | = | United Kingdom |
bpm | = | beats per minute |
h | = | hours |
NASA-TLX | = | NASA Task Load Index |
min | = | minutes |
mph | = | miles per hour |
ATS | = | ANOVA-type statistic |
CI | = | confidence interval |
TERG-2021-0195-File007.docx
Download MS Word (12.3 KB)TERG-2021-0195-File006.docx
Download MS Word (64.5 KB)TERG-2021-0195-File005.docx
Download MS Word (15.4 KB)TERG-2021-0195-File004.docx
Download MS Word (471 KB)TERG-2021-0195-File003.docx
Download MS Word (22.1 KB)TERG-2021-0195-File002.docx
Download MS Word (78.8 KB)Disclosure statement
No potential conflict of interest was reported by the authors.
Data availability statement
The data that support the findings of this study are openly available on Figshare at https://figshare.com/s/eeaee0f0decf48d90ce6.
Additional information
Funding
References
- Acharya, U. R., K. P. Joseph, N. Kannathal, C. M. Lim, and J. S. Suri. 2006. “Heart Rate Variability: A Review.” Medical & Biological Engineering & Computing 44 (12): 1031–1051. doi:https://doi.org/10.1007/s11517-006-0119-0.
- Amado, Sonia, Elvan Arıkan, Gülin Kaça, Mehmet Koyuncu, and B. Nilay Turkan. 2014. “How Accurately Do Drivers Evaluate Their Own Driving behavior? An On-Road Observational Study.” Accident; Analysis and Prevention 63: 65–73. doi:https://doi.org/10.1016/j.aap.2013.10.022.
- Anderson, C. A., N. L. Carnagey, and J. Eubanks. 2003. “Exposure to Violent Media: The Effects of Songs with Violent Lyrics on Aggressive Thoughts and Feelings.” Journal of Personality and Social Psychology 84 (5): 960–971. doi:https://doi.org/10.1037/0022-3514.84.5.960.
- Arnett, J. 1991. “Heavy Metal Music and Reckless Behavior among Adolescents.” Journal of Youth and Adolescence 20 (6): 573–592. doi:https://doi.org/10.1007/BF01537363.
- Beh, H. C., and R. Hirst. 1999. “Performance on Driving-Related Tasks during Music.” Ergonomics 42 (8): 1087–1098. doi:https://doi.org/10.1080/001401399185153.
- Bonneville-Roussy, A., and J. Rust. 2018. “Age Trends in Musical Preferences in Adulthood: 2. Sources of Social Influences as Determinants of Preferences.” Musicae Scientiae 22 (2): 175–195. doi:https://doi.org/10.1177/1029864917704016.
- Brodsky, W. 2001. “The Effects of Music Tempo on Simulated Driving Performance and Vehicular Control.” Transportation Research Part F: Traffic Psychology and Behaviour 4 (4): 219–241. doi:https://doi.org/10.1016/S1369-8478(01)00025-0.
- Brodsky, W. 2015. Human Factors in Road and Rail Transport. Driving with Music: Cognitive-Behavioural Implications. London: Ashgate Publishing.
- Brodsky, W. 2018. “A Performance Analysis of in-Car Music Engagement as an Indication of Driver Distraction and Risk.” Transportation Research Part F: Traffic Psychology and Behaviour 55: 210–218. doi:https://doi.org/10.1016/j.trf.2018.02.034.
- Brodsky, W., and M. Kizner. 2012. “Exploring an Alternative in-Car Music Background Designed for Driver Safety.” Transportation Research Part F: Traffic Psychology and Behaviour 15 (2): 162–173. doi:https://doi.org/10.1016/j.trf.2011.12.001.
- Brodsky, W., and Z. Slor. 2013. “Background Music as a Risk Factor for Distraction among Young-Novice Drivers.” Accident; Analysis and Prevention 59: 382–393. doi:https://doi.org/10.1016/j.aap.2013.06.022.
- Cassidy, G. G., and R. A. MacDonald. 2010. “The Effects of Music on Time Perception and Performance of a Driving Game.” Scandinavian Journal of Psychology 51 (6): 455–464. doi:https://doi.org/10.1111/j.1467-9450.2010.00830.x.
- Cassidy, G., and R. MacDonald. 2009. “The Effects of Music Choice on Task Performance: A Study of the Impact of Self-Selected and Experimenter-Selected Music on Driving Game Performance and Experience.” Musicae Scientiae 13 (2): 357–386. doi:https://doi.org/10.1177/102986490901300207.
- Chanda, M. L., and D. J. Levitin. 2013. “The Neurochemistry of Music.” Trends in Cognitive Sciences 17 (4): 179–193. doi:https://doi.org/10.1016/j.tics.2013.02.007.
- Chien, P. J., and S. H. Chan. 2015. “Old Songs Can Be as Fresh as New: An ERP Study on Lyrics Processing.” Journal of Neurolinguistics 35: 55–67. doi:https://doi.org/10.1016/j.jneuroling.2015.02.002.
- Cohen, J. 1988. Statistical Power Analysis for the Behavioral Sciences. Cambridge, MA: Academic Press.
- Dalton, B. H., D. G. Behm, and A. Kibele. 2008. “Effects of Sound Types and Volumes on Simulated Driving, Vigilance Tasks and Heart Rate.” Occupational Ergonomics 7 (3): 153–168. doi:https://doi.org/10.3233/OER-2007-7302.
- Department for Transport 2020. Reported road casualties in Great Britain: provisional results 2019. https://assets.publishing.service.gov.uk/government/uploads/system/uploads/attachment_data/file/904698/rrcgb-provisional-results-2019.pdf
- Depue, R. A. 2006. “Interpersonal Behavior and the Structure of Personality: Neurobehavioral Foundation of Agentic Extraversion and Affiliation.” In Biology of Personality and Individual Differences, edited by T. Canli, 60–92. Lausanne: The Guilford Press.
- Depue, R. A., and Y. Fu. 2013. “On the Nature of Extraversion: Variation in Conditioned Contextual Activation of Dopamine-Facilitated Affective, Cognitive, and Motor Processes.” Frontiers in Human Neuroscience 7: 288. doi:https://doi.org/10.3389/fnhum.2013.00288.
- Desmond, P. A., P. A. Hancock, and J. L. Monette. 1998. “Fatigue and Automation-Induced Impairments in Simulated Driving Performance.” Transportation Research Record: Journal of the Transportation Research Board 1628 (1): 8–14. doi:https://doi.org/10.3141/1628-02.
- Dibben, N., and V. J. Williamson. 2007. “An Exploratory Survey of in-Vehicle Music Listening.” Psychology of Music 35 (4): 571–589. doi:https://doi.org/10.1177/0305735607079725.
- Eysenck, H. J. 1994. “Personality: Biological Foundations.” In The Neuropsychology of Individual Differences, edited by P. A. Vernon, 151–207. Cambridge, MA: Academic Press. doi:https://doi.org/10.1016/B978-0-12-718670-2.50011-6.
- FakhrHosseini, S. M., and M. Jeon. 2019. “How Do Angry Drivers Respond to Emotional Music? A Comprehensive Perspective on Assessing Emotion.” Journal on Multimodal User Interfaces 13 (2): 137–150. doi:https://doi.org/10.1007/s12193-019-00300-3.
- Faul, F., E. Erdfelder, A. Buchner, and A. Lang. 2009. “Statistical Power Analyses Using G*Power 3.1: Tests for Correlation and Regression analyses.” Behavior Research Methods 41 (4): 1149–1160. doi:https://doi.org/10.3758/BRM.41.4.1149.
- Fuller, R. 2011. “Driver Control Theory: From Task Difficulty Homeostasis to Risk Allostasis.” In Handbook of the 4th International Conference on Traffic and Transport Psychology, edited by B. Porter. Cambridge, MA: Academic Press. doi:https://doi.org/10.1016/B978-0-12-381984-1.0002-5.
- Furnham, A., and L. Strbac. 2002. “Music is as Distracting as Noise: The Differential Distraction of Background Music and Noise on the Cognitive Test Performance of Introverts and Extraverts.” Ergonomics 45 (3): 203–217. doi:https://doi.org/10.1080/00140130210121932.
- Gulian, E., L. M. Debney, A. I. Glendon, D. R. Davies, and G. Matthews. 1989. “Coping with Driver Stress.” In Stress and Tension Control, 3rd ed., edited by F. McGuigan, W. E. Sime, and J. M. Wallace, 173–186. Amsterdam: Springer.
- Hart, S. G., and L. E. Staveland. 1988. “Development of NASA-TLX (Task Load Index): Results of Empirical and Theoretical Research.” In Human Mental Workload, edited by P. A. Hancock and N. Meshkati. Amsterdam: North Holland Press.
- Ho, C., and C. Spence. 2012. The Multisensory Driver: Implications for Ergonomic Car Interface Design. Champaign, IL: Ashgate Publishing.
- Ito, K., Y. Harada, T. Tani, Y. Hasegawa, H. Nakatsuji, Y. Tate, H. Seto, T. Aikawa, N. Nakayama, and M. Ohkura. 2017. “Evaluation of Feelings of Excitement Caused by Auditory Stimulus in Driving Simulator Using Biosignals.” In Advances in Affective and Pleasurable Design, edited by W. Chung and C. S. Shin, 231–240. Berlin: Springer.
- Karageorghis, C. I., and P. C. Terry. 2011. Inside Sport Psychology. Champaign, IL: Human Kinetics.
- Karageorghis, C. I., G. Kuan, W. Payre, E. Mouchlianitis, L. W. Howard, N. Reed, and A. M. Parkes. 2021a. “Psychological and Psychophysiological Effects of Music Intensity and Lyrics on Simulated Urban Driving.” Transportation Research Part F: Traffic Psychology and Behaviour 81: 329–341. doi:https://doi.org/10.1016/j.trf.2021.05.022.
- Karageorghis, C. I., E. Mouchlianitis, W. Payre, G. Kuan, L. W. Howard, N. Reed, and A. M. Parkes. 2021b. “Psychological, Psychophysiological and Behavioural Effects of Participant-Selected vs. Researcher-Selected Music in Simulated Urban Driving.” Applied Ergonomics 96: 103436. doi:https://doi.org/10.1016/j.apergo.2021.103436.
- Konz, S., and D. McDougal. 1968. “The Effect of Background Music on the Control Activity of an Automobile Driver.” Human Factors 10 (3): 233–243. doi:https://doi.org/10.1177/001872086801000305.
- Li, R., Y. V. Chen, and L. Zhang. 2019. “ Effect of Music Tempo on Long-Distance Driving: Which Tempo Is the Most Effective at Reducing Fatigue?” i-Perception 10 (4): 2041669519861982. doi:https://doi.org/10.1177/2041669519861982.
- Lien, H. C., W. M. Sun, Y. H. Chen, H. Kim, W. Hasler, and C. Owyang. 2003. “Effects of Ginger on Motion Sickness and Gastric Slow-Wave Dysrhythmias Induced by Circular Vection.” American Journal of Physiology: Gastrointestinal and Liver Physiology 284 (3): 481–489. doi:https://doi.org/10.1152/ajpgi.00164.2002.
- Maples-Keller, J. L., R. L. Williamson, C. E. Sleep, N. T. Carter, W. K. Campbell, and J. D. Miller. 2019. “Using Item Response Theory to Develop a 60-Item Representation of the NEO PI-R Using the International Personality Item Pool: Development of the IPIP-NEO-60.” Journal of Personality Assessment 101 (1): 4–15. doi:https://doi.org/10.1080/00223891.2017.1381968.
- Massaro, S., and L. Pecchia. 2019. “Heart Rate Variability (HTV) Analysis: A Methodology for Organizational Neuroscience.” Organizational Research Methods 22 (1): 354–393. doi:https://doi.org/10.1177/1094428116681072.
- McMahon, K., B. Sparrow, L. Chatman, and T. Riddle. 2011. “Driven to Distraction: The Impact of Distracter Type on Unconscious Decision Making.” Social Cognition 29 (6): 683–698. doi:https://doi.org/10.1521/soco.2011.29.6.683.
- Miao, L., Y. Gu, L. He, H. Wang, D. C. Schwebel, and Y. Shen. 2021. “The Influence of Music Tempo on Mental Load and Hazard Perception of Novice Drivers.” Accident; Analysis and Prevention 157: 106168. doi:https://doi.org/10.1016/j.aap.2021.106168.
- Mistry, H. 2015. “Music While You Work: The Effects of Background Music on Test Performance Amongst Extroverts and Introverts.” Journal of Applied Psychology and Social Science 1 (1): 1–14.
- Mizoguchi, K., and S. Tsugawa. 2012. “Influence of in-Vehicle Music on Driving: Experimental Results with a Driving Simulator.” 2012 IEEE International Conference on Vehicular Electronics and Safety (ICVES 2012), 117–121. IEEE. doi:https://doi.org/10.1109/ICVES.2012.6294296.
- Nakahara, H., S. Furuya, S. Obata, T. Masuko, and H. Kinoshita. 2009. “Emotion-Related Changes in Heart Rate and Its Variability during Performance and Perception of Music.” Annals of the New York Academy of Sciences 1169 (1): 359–362. doi:https://doi.org/10.1111/j.1749-6632.2009.04788.x.
- Navarro, J., F. Osiurak, and E. Reynaud. 2018. “Does the Tempo of Music Impact Human Behavior behind the Wheel?” Human Factors 60 (4): 556–574. doi:https://doi.org/10.1177/0018720818760901.
- Navarro, J., F. Osiurak, V. Gaujoux, M. C. Ouimet, and E. Reynaud. 2019. “Driving under the Influence: How Music Listening Affects Driving Behaviors.” Journal of Visualized Experiments 145 (145): 58342. doi:https://doi.org/10.3791/58342.
- Niu, J., C. Ma, J. Liu, L. Li, T. Hu, and L. Ran. 2020. “Is Music a Mediator Impacting Car following When Driver's Personalities Are Considered.” Accident; Analysis and Prevention 147: 105774. doi:https://doi.org/10.1016/j.aap.2020.105774.
- Noguchi, K., Y. R. Gel, E. Brunner, and F. Konietschke. 2012. “nparLD: An R Software Package for the Nonparametric Analysis of Longitudinal Data in Factorial Experiments.” Journal of Statistical Software 50 (12). doi:https://doi.org/10.18637/jss.v050.i12.
- North, A. C., and D. J. Hargreaves. 2008. The Social and Applied Psychology of Music. Oxford: Oxford University Press.
- Oltedal, S., and T. Rundmo. 2006. “The Effect of Personality and Gender on Risky Driving Behaviour and Accident Involvement.” Safety Science 44 (7): 621–628. doi:https://doi.org/10.1016/j.ssci.2005.12.003.
- Parnell, K. J., J. Rand, and K. L. Plant. 2020. “A Diary Study of Distracted Driving Behaviours.” Transportation Research Part F: Traffic Psychology and Behaviour 74: 1–14. doi:https://doi.org/10.1016/j.trf.2020.08.003.
- Regan, M., T. Victor, and J. Lee. 2013. Driver Distraction and Inattention: Advances in Research and Countermeasures. Boca Raton, FL: SAE.
- Riener, A., A. Ferscha, and M. Aly. 2009. “Heart on the Road: HRV Analysis for Monitoring a Driver's Affective State.” Proceedings of the 1st International Conference on Automotive User Interfaces and Interactive Vehicular Applications, 99–106. doi:https://doi.org/10.1145/1620509.1620529.
- Royal, D. 2003. National Survey of Distracted and Drowsy Driving Attitudes and Behaviors: 2002. National Highway Traffic Safety Administration. http://www.nhtsa.gov/people/injury/drowsy_driving1/survey-distractive03/Technical_Page1.htm
- Russell, J. A., A. Weiss, and G. A. Mendelsohn. 1989. “Affect Grid: A Single-Item Scale of Pleasure and Arousal.” Journal of Personality and Social Psychology 57 (3): 493–502. doi:https://doi.org/10.1037/0022-3514.57.3.493.
- Solhjoo, Soroosh, Mark C. Haigney, Elexis McBee, Jeroen J G. van Merrienboer, Lambert Schuwirth, Anthony R. Artino, Alexis Battista, Temple A. Ratcliffe, Howard D. Lee, and Steven J. Durning. 2019. “Heart Rate and Heart Rate Variability Correlate with Clinical Reasoning Performance and Self-Reported Measures of Cognitive Load.” Scientific Reports 9 (1): 14668. doi:https://doi.org/10.1038/s41598-019-50280-3.
- Tabachnick, B. G., and L. S. Fidell. 2019. Using Multivariate Statistics. 7th ed. New York, NY: Pearson.
- Teh, E., S. Jamson, O. Carsten, and H. Jamson. 2014. “Temporal Fluctuations in Driving Demand: The Effect of Traffic Complexity on Subjective Measures of Workload and Driving Performance.” Transportation Research Part F: Traffic Psychology and Behaviour 22: 207–217. doi:https://doi.org/10.1016/j.trf.2013.12.005.
- Törnros, J., and A. Bolling. 2006. “Mobile Phone Use–Effects of Conversation on Mental Workload and Driving Speed in Rural and Urban Environments.” Transportation Research Part F: Traffic Psychology and Behaviour 9 (4): 298–306. doi:https://doi.org/10.1016/j.trf.2006.01.008.
- Ünal, A. B., D. de Waard, K. Epstude, and L. Steg. 2013a. “Driving with Music: Effects on Arousal and Performance.” Transportation Research Part F: Traffic Psychology and Behaviour 21: 52–65. doi:https://doi.org/10.1016/j.trf.2013.09.004.
- Ünal, A. B., S. Platteel, L. Steg, and K. Epstude. 2013b. “Blocking-Out Auditory Distracters While Driving: A Cognitive Strategy to Reduce Task-Demands on the Road.” Accident; Analysis and Prevention 50: 934–942. doi:https://doi.org/10.1016/j.aap.2012.07.020.
- Ünal, A. B., L. Steg, and K. Epstude. 2012. “The Influence of Music on Mental Effort and Driving Performance.” Accident; Analysis and Prevention 48: 271–278. doi:https://doi.org/10.1016/j.aap.2012.01.022.
- van der Zwaag, M. D., C. Dijksterhuis, D. De Waard, B. L. Mulder, J. H. Westerink, and K. A. Brookhuis. 2012. “The Influence of Music on Mood and Performance While Driving.” Ergonomics 55 (1): 12–22. doi:https://doi.org/10.1080/00140139.2011.638403.
- van der Zwaag, M. D., J. H. Janssen, C. Nass, J. H. Westerink, S. Chowdhury, and D. de Waard. 2013. “Using Music to Change Mood While Driving.” Ergonomics 56 (10): 1504–1514. doi:https://doi.org/10.1080/00140139.2013.825013.
- Vuilleumier, P., J. Armony, and R. Dolan. 2003. “Reciprocal Links between Emotion and Attention.” In Human Brain Function, 2nd ed., edited by R. S. J. Frackowiak, 419–444. Cambridge, MA: Academic Press.
- Wen, H., N. N. Sze, Q. Zeng, and S. Hu. 2019. “Effect of Music Listening on Physiological Condition, Mental Workload, and Driving Performance with Consideration of Driver Temperament.” International Journal of Environmental Research and Public Health 16 (15): 2766. doi:https://doi.org/10.3390/ijerph16152766.
- Young, M. S., J. M. Mahfoud, G. H. Walker, D. P. Jenkins, and N. A. Stanton. 2008. “Crash Dieting: The Effects of Eating and Drinking on Driving Performance.” Accident; Analysis and Prevention 40 (1): 142–148. doi:https://doi.org/10.1016/j.aap.2007.04.012.
- Zohar, A. H., C. R. Cloninger, and R. McCraty. 2013. “Personality and Heart Rate Variability: exploring Pathways from Personality to Cardiac Coherence and Health.” Open Journal of Social Sciences 1 (6): 32–39. doi:https://doi.org/10.4236/jss.2013.16007.
- Zöller, I., B. Abendroth, and R. Bruder. 2019. “Driver Behaviour Validity in Driving Simulators – Analysis of the Moment of Initiation of Braking at Urban Intersections.” Transportation Research Part F: Traffic Psychology and Behaviour 61: 120–130. doi:https://doi.org/10.1016/j.trf.2017.09.008.