Abstract
The current study aimed to identity the optimal low-cost stroller cooling strategies for use in hot and moderately humid summer weather. A commercially available stroller was instrumented to assess the key parameters of the thermal environment. The cooling efficacy of eight different stroller configurations was examined in a counterbalanced order across 16 hot summer days (air temperature (Ta) = 33.3 ± 4.1 °C; relative humidity = 36.7 ± 15%; black globe temperature = 43.9 ± 4.6 °C). Compared with a standard-practice stroller configuration, combining a moist muslin draping with a battery-operated clip-on fan provided optimal in-stroller cooling, reducing the end-trial air temperature by 4.7 °C and the wet bulb globe temperature (WBGT) by 1.4 °C. In contrast, in-stroller temperatures were substantially increased by draping a dry muslin (Ta = +2.6 °C; WBGT = +0.9 °C) or flannelette (Ta = +3.7 °C; WBGT = +1.4 °C) cloth over the stroller carriage. These findings provide empirical evidence which may inform guidance aimed at protecting infants during hot weather.
Practitioner summary: This study examined the efficacy of traditional and novel stroller cooling strategies for use in hot and moderately humid weather. Covering the carriage with a dry muslin cloth substantially increased stroller temperatures and should be avoided. Evaporative cooling methods reduced in-stroller temperatures. A moist muslin cloth draping combined with a fan provided optimal stroller cooling.
Introduction
During periods of hot weather, the potential for infant hyperthermia is of particular concern as this can lead to adverse clinical symptoms including hypotension, dehydration, seizures and, in severe cases, infant fatality (Brand and Shippey Citation2021; Gardner and Cammack Citation2021). Heat stress has also been linked with sudden infant death syndrome (SIDS) (Fleming et al. Citation1990; Guntheroth and Spiers Citation2001) which is the leading cause of post-neonatal unexplained deaths worldwide (Elhaik Citation2019). These safety concerns are particularly salient given a limited thermoregulatory capacity during infancy, owing largely to unique morphological and physiological characteristics (e.g. higher body surface area to mass ratio, more limited sweating capacity) which may render infants liable to hyperthermia if exposed to high ambient temperatures for prolonged periods (Inoue, Kuwahara, and Araki Citation2004; Székely and Garai Citation2018). Furthermore, infants are highly dependent on their adult caregivers to ensure dangerous hyperthermia is avoided through appropriate behavioural intervention such as the removal of excess clothing, avoidance of radiant heat sources (e.g. solar radiation) and the seeking of cool shelter. Ensuring caregivers are provided with evidenced-based knowledge and guidance has been identified as being crucial to improving a wide range of health outcomes during childhood (CDC Citation2021).
On a day-to-day basis, exposure to environmental heat stress is not always avoidable during infancy, especially when caregivers engage in outdoor active travel or recreational activity. Indeed, outdoor physical activity is widely encouraged during the weeks and months after pregnancy due to both perceived and well-established benefits conferred to both mother and her young child (Bull et al. Citation2020; Currie and Develin Citation2002). During such activities, it is common practice for infants to be transported in strollers (pushchairs) (Birken et al. Citation2015) during which they can be exposed to significant levels of heat stress during hot summer weather. By design, strollers are typically semi-enclosed carriages built to facilitate both structural integrity and infant comfort (Sehat, Nirmal, and Jin Citation2017). However, such design features may result in compromised airflow and the creation of a space prone to heat absorption and retention—all of which exacerbate the risk for infant heat exposure.
Efforts to reduce the risk of stroller overheating have seen numerous techniques adopted by caregivers. Typically, these methods focus on reducing solar influx to the stroller carriage using material coverings e.g. muslin cloth (NSW Health, Citation2020) or sunshades/parasols (NHS (National Health Service) Citation2021) in an attempt to minimise ultraviolet (UV) radiation exposure within the stroller carriage, protecting against the long-term health consequences of UV-induced skin damage (Green, Wallingford, and McBride Citation2011). In addition, more sophisticated cooling methods such as battery-operated fans that attach to the stroller frame are also commercially available. However, despite the widespread adoption of such ‘cooling techniques’ their overall efficacy is not supported by empirical evidence and some anecdotal reports even suggest material stroller coverings may instead accelerate in-stroller heating (Burnie and Howe Citation2019; Holmberg Citation2014). In contrast, an understanding of the basic principles of heat exchange, raises the possibility of novel low-cost stroller interventions that harness the power of evaporation. Evaporative cooling methods have been utilised effectively in other settings to help adults manage heat stress severity (e.g. misting fans (Graham et al. Citation2021; Lynch et al. Citation2018, Mitchell, McFarlin, and Dugas Citation2003), water sprays (Morris et al. Citation2019), moistening of clothing (Cramer et al. Citation2020)) and may be well-suited for safe use in the context of infant stroller heat management.
Whilst thermal manikins have been used to assess infant heat stress in a range of different settings (e.g. Danielsson Citation2004; Lahana et al. Citation2018), to the best of our knowledge no previous study has systematically examined the cooling efficacy of stroller cooling strategies, and moreover, in ecologically valid settings. Accordingly, the current study aimed to empirically examine the cooling efficacy of eight different stroller configurations; namely the stroller with: (a) the canopy closed (which served as the standard-practice control intervention); (b) a commercially available battery operated clip-on fan; (c) a white muslin cloth draped over the front of the carriage; (d) a flannelette cloth (made of polyester) draped over the front of the carriage; (e) a combination of muslin cloth and clip-on fan; (f) a combination of flannelette cloth and clip-on fan; g) a completely moistened muslin cloth draping; and (h) a combination of a completely moistened muslin cloth draping and clip-on fan. It was hypothesised that the stroller configurations that incorporated evaporative cooling techniques would reduce the heat stress severity experienced inside the stroller carriage, whereas those that instead utilised a dry material covering would exacerbate the level of in-stroller heat stress.
Methods
All experimental trials were conducted at the University of Sydney (Cumberland campus, Lidcombe, NSW) between November 2019 and February 2020, during the Australian summer. No human participants were involved in this study and, therefore, human ethical approval was not required. Experimental trials were planned by consulting the Bureau of Meteorology (BOM) weather forecast based on the requirement that: (1) the peak ambient temperature was forecasted to be ≥30 °C for at least 1 h; (2) with minimal to no cloud cover; (3) and no rainfall.
A total of 16 experimental trials were conducted, each on different days. Each experimental trial consisted of the separate 20-min testing of each stroller configuration () in a counterbalanced order. Including the set-up time and equilibration period required between each stroller configuration, each trial lasted approximately 4.5 h. All trials commenced at 1100 h (±30 min) and were completed by 1530 h (±30 min).
Table 1. Summary description of cooling interventions (see for images).
A standard infant stroller (Inglesina Trilogy stroller, Italy) made with a lightweight and waterproof polyester canvas material with full-recline backrest, full-pivoting canopy and a removable bumper bar was used for all trials. The stroller is its unfolded position presented with the dimensions of 56 cm (width), 105.5 cm (height) and 82 cm (depth), with a weight of 9.6 kg. This stroller design was selected for testing as it was commercially available and deemed to be broadly ‘typical’ in dimension and material composition. Future research is warranted to examine if the current findings are generalisable to other stroller models with different design features. It should be noted that the cooling interventions tested in the current study were selected as they could be feasibly used in conjunction with most standard stroller designs. During all trials, the stroller was pushed by the same researcher at a comfortable walking speed (∼1.4 m/s) along a pre-determined 72.5 m outdoor route (see ). The set-up of the stroller between configurations was conducted in an adjacent air-conditioned room (temperature of 23 ± 1 °C; relative humidity of 70 ± 5%) over the course of 10-min to ensure the baseline in-stroller temperature conditions were consistent between configurations.
Figure 1. Schematic diagram of pre-determined 72.5 m route along which the stroller was pushed during each 20-min intervention. The red dashed lines indicate the stroller route, the red arrows indicate the direction, the hatched circle indicates the location of the environmental measuring unit, placed on the tarmac of the nearby parking lot unobstructed.
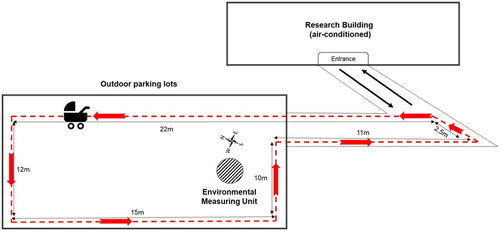
Instrumentation
Temperature sensors
A purpose-built wire frame was fitted into the internal stroller carriage to which six temperature sensors (YSI409B with 9.5 mm sensor, range of operation: 0–60 °C; accuracy: ±0.2 °C from 0 °C to 60 °C, ±0.1 °C from 25 °C to 45 °C) were suspended to measure air temperature. These temperature sensors were shielded from direct solar radiation by the stroller canopy (which was closed) or the stroller canopy and the cloth covering in combination. Four thermistors were positioned to simulate the location of body segments of an infant (i.e. head, chest, abdomen, and lower limbs); and two thermistors were suspended 15 cm from the top of the canopy to provide an indication of the thermal conditions in the upper portion of the stroller carriage space (). All thermometric measurements were sampled at 5-s intervals via a portable data acquisition box and displayed in real-time via a laptop using LabView software (v7.0. National Instruments, Austin, TX), for a total duration of 20 min per intervention.
Figure 2. In-stroller thermistors and Kestrel 5400-unit placement within the purpose-built wire frame. Numbers 1–4 indicate individual thermistor placement relative to a simulated infant’s body segments (i.e. head, chest, abdomen, and legs). Numbers 5 and 6 indicate the thermistor placement measuring the temperature in the upper portion of the stroller carriage space, close to the canopy. The orange icon indicates the location of the Kestrel 5400 unit, placed near the centre of the wire frame, which was suspended 15 cm above the stroller seat surface.
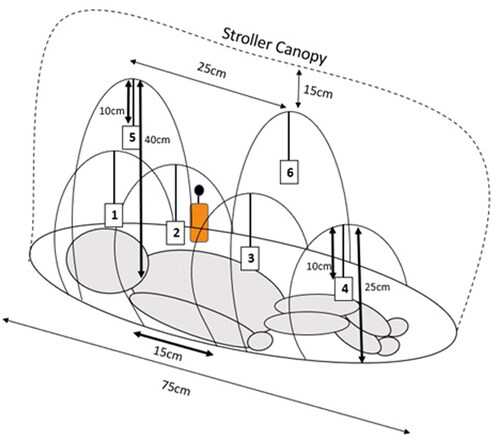
Other in-stroller environmental parameters
A Kestrel 5400 series (Nielsen-Kellerman, Pennsylvania, PA) portable unit was also positioned in the stroller to measure in-stroller air temperature (Ta), relative humidity (RH), natural wet bulb temperature (Tnwb), black globe temperature (Tglobe), wet bulb globe temperature (WBGT) and air velocity. WBGT was calculated using the following equation: WBGT= 0.7Twb + 0.2 Tglobe + 0.1 Tdb. The unit was placed in the centre of the stroller carriage in an upright position () and was secured 15 cm above the stroller seat surface by the purpose-built wire frame. All measurements were sampled at 5-s intervals.
External environmental parameters
Outdoor air temperature, relative humidity, wind speed, and black globe temperature were measured by an environmental measuring unit (EMU) designed and built by the University of Sydney. Wind speed and direction were measured using an ultrasonic anemometer (Gill Instruments, UK). Black globe temperature was measured using a 100 mm platinum resistance thermometer (Temperature Controls, Australia) placed inside a 150 mm diameter copper globe (Irrigation Direct, Australia) painted matt black. Air temperature and wet bulb temperature were measured using two 100 mm platinum resistance thermometers (Temperature Controls, Australia). Both thermometers were mechanically aspirated with one covered with a cotton shroud and saturated with distilled water. A temperature data logger unit (Pico Technology PT-104, RS Components, Australia) with an accompanying PicoLog 6 application programming software was used to record temperature measurements. The instruments were mounted on a modified tripod stand (Jaycar, Australia) at a height of 1.2 m. During all trials, the EMU was placed at a standardised, unobstructed location along the pre-determined route (see ). All environmental measurements were sampled and recorded at 5-s intervals via a tablet (Microsoft Surface Go) using customised software with the data subsequently exported for analysis.
Stroller configurations
The eight stroller configurations tested in the study were selected due to their conventional day-to-day use, using inexpensive and readily available resources (see , ). In the Closed Stroller configuration, the stroller canopy was put down, shading the stroller carriage from solar radiation whilst not impeding the airflow through the carriage (). We opted for this control configuration as we deemed it likely that caregivers would opt to close the canopy during hot weather to help avoid excessive UV exposure.
Figure 3. The tested stroller cooling interventions. All fabric drapings were secured using plastic clothes pegs, ensuring that there were no gaps present. For illustrative purposes, the drapings of interventions E and F were raised on one side to display the position of the battery-operated fan.
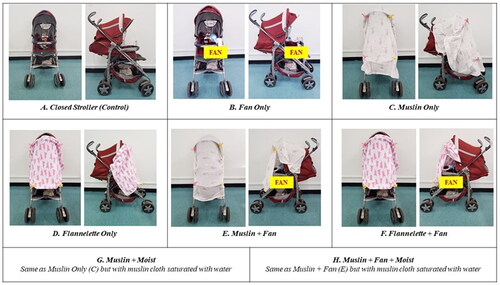
During the configurations involving the clip-on battery-operated fan (Tee-Zed Products, Guangdong, China), new batteries were used for each trial to avoid decrements in maximal fan speed, which was confirmed before each trial to be ∼1 m/s. Muslin coverings (112 cm by 112 cm) made from 100% cotton weave were used due to its high capacity to absorb moisture. One dry muslin covering was used for both the Muslin Only and Muslin + Fan trials. Two separate muslin coverings, which were saturated with tap water, were used for the Muslin + Moist intervention, and the Muslin + Fan + Moist intervention. The flannelette covering (112 cm by 112 cm), made from 100% polyester, was not moistened as it was a non-absorbent material. Both the muslin and flannelette coverings were draped over the stroller carriage as single layer (i.e. unfolded).
Experimental protocol
Before the start of experimentation, three muslin covering were prepared for use. Two of these muslin coverings were weighed with a digital scale (±0.1 g, Mettler Toledo, MS12001L) before and after being soaked in room temperature tap water and wrung-out to ensure complete saturation but to avoid dripping. These wet muslin coverings were then stored in separate sealed plastic bags until use to prevent water loss via evaporation.
The set-up of each stroller configuration was conducted in an air-conditioned room set to 23 °C. The stroller backrest was placed in a full recline (supine) position to simulate a young infant’s (from birth to 6 months) typical positioning.
Based on the counterbalanced order, one of the eight stroller configurations was assigned as the first to be tested and the stroller was prepared accordingly. Before the prepared stroller was taken out of the air-conditioned room, a five-minute baseline period was observed to ensure that all stroller temperature sensors had equilibrated to room temperature (within 1 °C), across all temperature sensors. The stroller was then pushed continually outdoors along the pre-determined (see ) at a comfortable walking speed of ∼1.4 m/s. Each stroller configuration was tested for 20 min, at which point the stroller was pushed back to the air-conditioned room and placed in front of a pedestal fan to cool the interior carriage of the stroller to baseline room temperature. Once cooled, the stroller was prepared for the next experimental intervention. This process was repeated eight times each experimental day until all stroller configurations had been tested ().
Statistical analysis
All data are reported as means with standard deviation, unless otherwise stated. The GraphPad Prism (version 8.3.0, GraphPad Software, La Jolla, CA) software was used for all data analyses. Environmental parameter data for each stroller configuration were averaged over the 16 days of experimental testing. Separate one-way repeated measures ANOVA with the independent variable of stroller configuration (eight levels: Stroller Closed; Fan Only; Muslin Only; Flannelette Only; Muslin + Fan; Flannelette + Fan; Muslin + Moist; Muslin + Fan + Moist) were used to analyse the differences in dependent variables (mean and end-of-trial environmental parameters). Paired T-tests were used to compare the volume of water evaporated, rate of water evaporation and the heat liberated from the evaporation of water from the muslin cloths. An alpha of 0.05 was used to determine statistical significance, hence, the probability of making type 1 error in all tests was maintained at 5%. When a significant effect was found, planned pairwise comparisons were made against the Closed Stroller Control configuration using two-tailed paired-samples t-tests, with Bonferroni adjustments made for multiple comparisons.
Results
Outdoor environmental conditions
The average outdoor air temperature (p = 0.257), relative humidity (p = 0.224), black globe temperature (p = 0.388), air velocity (0.89 ± 0.33 ms−1, p = 0.135), and WBGT (p = 0.598) did not differ across the testing of the eight stroller configurations. The end-trial outdoor environmental parameters for each stroller configuration are presented in .
Table 2. The mean (mean of 16 separate experimental observations) end of trial in-stroller and outside ambient environmental conditions and the mean differences observed between in-stroller vs outside for each stroller configuration.
In-stroller environmental conditions
The average temporal (minute averaged) in-stroller environmental conditions for each stroller configuration are displayed in .
Figure 4. The mean (minute averaged data) in-stroller environmental conditions for each stroller cooling intervention. Due to the number of interventions presented, error bars have been omitted for clarity.
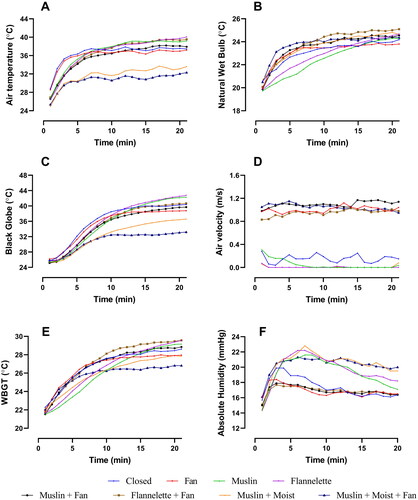
End-trial absolute stroller temperatures
An effect of stroller configuration was observed for absolute in-stroller air temperature (p < 0.001), relative humidity (p < 0.001), black globe temperature (p < 0.001) and WBGT (p < 0.001). These data are presented in . In summary, the lowest absolute in-stroller air temperature was observed in Muslin + Moist + Fan (32.3 ± 4.2 °C) followed by Muslin + Moist (33.6 ± 5.1 °C), both of which were significantly lower than Closed Stroller Control (37.5 ± 4.7 °C) (p < 0.009). In contrast, the highest absolute in-stroller air temperature was observed in Flannelette Only (40.0 ± 4.8 °C), followed by the Flannelette + Fan (39.5 ± 4.2 °C) and Muslin Only (39.2 ± 4.5 °C) configurations, all of which were higher than the Closed Stroller Control (p < 0.026). No differences in absolute in-stroller air temperature were observed in the Fan Only (37.2 ± 4.8 °C) or the Muslin + Fan (37.9 ± 3.3 °C) configurations compared with the reference control configuration (P > 0.995).
The lowest absolute in-stroller WBGT was also observed in Muslin + Moist + Fan (26.7 ± 3.2 °C) which was lower than the Closed Stroller Control (28.3 ± 3.4 °C) (p = 0.009). In contrast, the highest absolute WBGT was observed in Flannelette Only (29.3 ± 3.5 °C) which was higher than the Closed Stroller Control configuration (p = 0.024). The in-stroller absolute WBGT did not differ in any other stroller configuration when compared with Closed Stroller Control (p > 0.072).
In-stroller vs outside temperatures
The differences between the end-trial internal stroller temperatures and the corresponding outside temperatures are presented in , with an effect of stroller configuration observed for all environmental parameters (p < 0.001). In Closed Stroller Control the internal stroller air temperature was 3.5 °C higher than outside (p < 0.0001), whilst WBGT was 0.7 °C higher (p = 0.0016). In comparison, during Muslin + Moist + Fan the in-stroller air temperature did not differ to outside (p = 0.124), however, the internal WBGT was lower than outside (p < 0.0001). During Muslin + Moist neither the in-stroller air temperature (p = 0.445) or WBGT (p = 0.446) differed to outside. During Fan Only, the air temperature was higher than outside (p < 0.0001), but the in-stroller WBGT did not differ (p = 0.796). All other stroller configurations resulted in a significantly higher in-stroller air temperature and WBGT compared with outside (p < 0.001)
Difference between in-stroller vs outside temperatures relative to Closed-Stroller control
When the differences in in-stroller vs. outside temperatures observed in the Closed Stroller Control (i.e. air temperature: +3.5 °C, WBGT: +0.7 °C) are used as anchors for comparison, the relative efficacy of the other stroller configurations in lowering the internal stroller temperatures are presented in .
Figure 5. The mean end-trial difference between the in-stroller vs outside air temperature (Ta) and WBGT, expressed relative to the difference observed during Closed Stroller Control. T1–T6 represent the mean relative ΔTa for each of the six thermistors located in the stroller chamber.* Significantly lower than Closed Stroller Control (p < 0.05). †Significantly higher than Closed Stroller Control (p < 0.05).
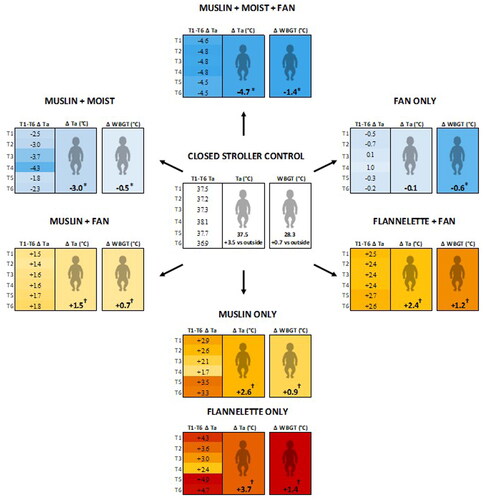
Relative to Closed Stroller Control, the greatest reduction in air temperature (p < 0.0001) and WBGT (p = 0.0004) was observed in Muslin + Moist + Fan. Smaller—but still significant—reductions in air temperature (p = 0.009) and WBGT (p = 0.015) were observed in Muslin + Moist, compared with the Closed Stroller Control reference condition. During the Fan Only configuration the in-stroller vs outside difference did not differ for air temperature relative to the Closed Stroller Control (p = 0.999) but a larger reduction in WBGT was observed (p = 0.033).
All other stroller configurations resulted in a larger elevation of in-stroller air temperature and WBGT compared with the Closed Stroller Control (p < 0.032). The most severe heating effect was observed in Flannelette Only followed by Muslin Only. Smaller—but still substantial—increases in in-stroller temperatures were observed in the Muslin + Fan and Flannelette + Fan configurations.
Water evaporation from moistened cloths
The mass of the muslin and flannelette cloths before and after water saturation and pre and post each experimental trial are presented in . The values were used to derive the amount of water evaporated from the cloth and the resultant heat liberation during each trial (i.e. water evaporation (g) = wet mass muslin Pre (g) – wet mass muslin Post (g)). The heat liberated by the evaporation of water from the wet muslin cloths was calculated assuming a latent heat of water evaporation of 2430 J/g at 30 °C.
Table 3. Muslin characteristics pre- and post-trial and the evaporative cooling yielded.
Discussion
The present study examined the efficacy of eight low-cost stroller configurations commonly adopted during hot and moderately humid summer weather to mitigate the development of in-stroller carriage heat stress. The main findings of the study are: (1) draping a light-coloured moist muslin cloth over the stroller carriage reduced both the air temperature and the WBGT inside the carriage; (2) this stroller configuration was further optimised when coupled with the use of a clip-on battery operated fan; (3) the use of clip-on fan in isolation yielded a marginal reduction in WBGT, but had no effect on air temperature; (4) all other configurations—including the draping of dry muslin and flannelette cloths over the stroller carriage—worsened the prevailing level of heat stress inside the stroller.
Stroller cooling: harnessing evaporation
The largest cooling effects were observed when a moist muslin cloth was draped over front of the stroller. This cooling effect can be attributed to the evaporation of moisture from the cloth resulting in the extraction of latent heat energy from the stroller carriage. When a moist muslin draping was used in isolation (Muslin + Moist) in-stroller air temperature was reduced by 3.0 °C compared with the standard-practice Closed Stroller control configuration (). Additionally, a 0.5 °C reduction in WBGT—a unified heat index which integrates the key parameters of the thermal environment to determine the overall heat stress severity—was also observed inside the stroller when covered with a moist muslin cloth.
The moist muslin draping provided an even larger cooling effect when combined with a battery-operated fan attached to the front of the stroller which circulated air around the stroller carriage at approximately 1 m/s (Muslin + Moist + Fan). The additional forced convection contributed to increased water evaporation from the cloth (∼22 g, ), further augmenting heat loss from the stroller, whilst also reducing the vertical temperature gradient evident throughout the carriage (). This combined intervention, reduced in-stroller air temperature by 4.7 °C and the WBGT by 1.4 °C, compared with the Closed Stroller Control. It should, however, be noted that whilst air temperature and WBGT were lowered by the wet drapings, the water vapour pressure (absolute humidity) inside the stroller was increased in the stroller carriage (, ), which may have implications for in-stroller thermal comfort.
Evaporative cooling techniques have been reported previously to be efficacious in an array of different contexts for adults exposed to heat stress, including via misting fans (Graham et al. Citation2021; Lynch et al. Citation2018; Mitchell, McFarlin, and Dugas Citation2003), water dousing (Morris et al. Citation2019) and the moistening of clothing garments (Cramer et al. Citation2020). Whilst it is important to acknowledge that the evaporative cooling techniques examined in the current study may be less effective in hot and very humid conditions owing to a lower evaporative potential of the environment, the current findings do highlight the potential utility of evaporative cooling techniques to help safeguard infants during hot and moderately humid weather. Future research is warranted to examine the efficacy of stroller cooling techniques under different climatic conditions.
Increasing convection via a battery-operated fan
When a battery-operated fan was used in isolation (Fan Only), the in-stroller air temperature did not differ to the Closed Stroller control. Thermodynamic principles explain this observation as increasing convection via a fan provides no physical means of heat extraction and merely facilitates additional circulation of warm ambient air which is, at best, equal to the outdoor shaded air temperature. However, the higher internal air velocity did result in a in a small (0.6 °C) reduction in WBGT, compared with the closed stroller control configuration. Critical environmental threshold limits for electric fan efficacy have been defined previously (Ravanelli et al. Citation2015; Hospers et al. Citation2020; Foster et al. Citation2022; Morris et al. Citation2021) and indicate that fans are always beneficial when air temperature is lower than human skin temperature, but can also confer thermoregulatory advantage at temperatures at least as high as 40 °C. However, the efficacy of fan use at these higher temperatures is reliant on their facilitation of additional sweat evaporation from the skin surface. Whilst most babies >37 weeks (gestational age) can secrete sweat (Harpin, Chellappah, and Rutter Citation1983), their maximal sudomotor capacity is thought to be up to three times lower than that observed in older children and adults (Foster, Hey, and Katz Citation1969). Additionally, the stroller setting is unique in that only half of the infant’s body surface area is available for convective and/or evaporative heat exchange as the other half is obscured by the seat which may in turn alter the efficacy of fan-based cooling interventions. Given these considerations, in-stroller fans (without accompanying evaporative cooling methods) should be used with caution, and future research is required to determine the upper environmental limits for safe and effective fan use during infancy.
In-stroller thermal environment: Beware of potential heating effects
It was noteworthy that other stroller covering techniques substantially exacerbated the in-stroller heat stress compared with the Closed Stroller control. The draping of both dry muslin and dry flannelette cloths over the front of the stroller carriage increased the in-stroller air temperature (Muslin Only = +2.6 °C; Flannelette Only = +3.7 °C) and WBGT (Muslin Only = +0.9 °C; Flannelette Only = +1.4 °C). The covering of the stroller entrance with either a dry muslin or flannelette cloth reduced natural ventilation through the stroller—as evidenced by lower air velocity observed in the covered stroller configurations ()—resulting in a net heating of the carriage as heat was gradually absorbed and retained.
To date, only anecdotal evidence of elevated in-stroller temperatures during hot weather has been reported (Holmberg Citation2014). However, more robust evidence derived from vehicle research indicates that even in moderately hot ambient conditions (e.g. 32 °C), the internal temperature of an enclosed vehicle (e.g. a car) can be up to 25 °C higher, leading to very dangerous levels of heat stress (McLaren et al. Citation2005; Vanos et al. Citation2018). Our data highlight that a similar phenomenon could manifest when the stroller carriage is encapsulated with a dry material covering. For example, during the final 15-min of Muslin Only configuration the stroller carriage heated at a rate of +0.20 °C/min, whist a similar heating rate of +0.26 °C/min was observed during the Flannelette Only strategy. It therefore stands to reason that substantial—and potentially hazardous—elevations in carriage temperature could develop over longer heat stress exposures (e.g. 1 h).
The addition of a battery-operated fan to the dry cloth configurations did attenuate the in-stroller heating effect yet failed to provide benefit relative to the Closed Stroller Control. In terms of material selection, the dry flannelette draping resulted in higher end-trial stroller temperatures, compared to the dry muslin covering; an observation likely explained by the additional thickness and polyester composition of the flannelette which is associated higher thermal resistance (O’Callaghan and Probert Citation1976; Alay, Alkan, and Göde Citation2012) which promotes additional heat retention. It should be acknowledged that the muslin and flannelette cloths were not matched exactly for colour which may have impacted the absorption, reflection, and transmission of radiant heat (Scipioni et al. Citation2020), however, both were both predominantly white in colour with a printed pattern (which was however slightly darker on the flannelette). However, regardless of material type, our data provide compelling evidence that dry material coverings that excessively impede airflow through the carriage should not be used to cover the stroller during periods of hot weather. One important caveat here is that whilst refraining from fully covering the stroller carriage with a material draping, it is important that efforts are taken to ensure the carriage is not directly exposed to solar radiation. For example, this may be achieved by closing the stroller canopy—as was the case in the Closed Stroller control configuration—or by other means such as the use of a parasol attached to the stroller frame which may serve to provide shade without impeding airflow through the stroller carriage.
Physiological relevance
Although the physiological consequences of the differing stroller configurations were not assessed, the thermo-physiological relevance of our findings is clear. Whilst wide variations in sweating responses are observed among infants depending on their age and maturation status (Rutter and Hull Citation1979), their absolute sweating capacity can be up to three-times lower than that of adults (Foster, Hey, and Katz Citation1969; Sulyok, Jéquier, and Prod’hom Citation1973). Although this impairment may be negated to a certain extent by a greater sweat output per unit of body mass due to a higher surface area-to-body mass ratio, it is generally accepted that infants exhibit a lower capacity to dissipate body heat via evaporative pathways compared with older children and adults (Gardner and Cammack Citation2021; Inoue, Kuwahara, and Araki Citation2004). Additionally, their surface area-to-mass ratio may also promote dry heat gain when ambient temperature exceeds skin temperature, further increasing the risk for infant hyperthermia. This is particularly saliant as babies/infants typically exhibit lower skin temperatures compared with older children and adults (Garcia-Souto and Dabnichki Citation2016) meaning that the skin-to-ambient temperature gradient is reversed in favour of heat storage (skin temperature < ambient temperature) at lower ambient temperatures. It is also desirable to limit the severity of heat stress experienced inside the stroller to avoid the need for infants to sweat excessively to help prevent the insidious development of hypohydration which may further exacerbate the risk for morbidity (Brand and Shippey Citation2021). Beside the risk of heat-related illness, infant heat stress may also result in thermal discomfort and disturbance to sleep patterns (Wailoo, Petersen, and WhitakerCitation1990; Filingeri et al. Citation2020). Although the upper thermal limits for the avoidance of infant thermal discomfort are poorly understood, the maximum tolerable air temperature is thought to be in the region of 35 °C for a naked baby and slightly lower when dressed (WHO (World Health Organisation) Citation1997)—only the Muslin + Moist and Muslin + Moist + Moist + Fan configurations succeeded in preventing the mean in-stroller temperature exceeding this threshold under the conditions assessed (see ).
Translational importance
The current findings may inform evidence-based guidance regarding stroller heat safety management which may be disseminated to caregivers through healthcare services (e.g. antenatal classes, online health advice, etc). Evaporative cooling techniques may be advocated as efficacious stroller cooling strategies for use in hot weather. In contrast, caregivers should be advised to refrain from using dry material coverings that limit natural ventilation through the stroller as these exacerbate the heat stress within the carriage. The relevance of these findings may also translate to other settings in which infant thermal comfort is of concern. Evaporative cooling may also be suitable for use in indoor settings during hot weather when air conditioning is not readily available. For example, the use of a moist muslin cloth in conjunction with a fan may be feasibly employed to help cool the internal chamber of a bassinet/cot in which infants typically sleep. However, it acknowledged that future research is required to better understand the interaction between temperature, humidity, and outcomes such as infant comfort and sleep quality and to ultimately determine the efficacy of the tested cooling interventions in free living settings. Such cooling methods are simple and affordable and may therefore be employed as an accessible heat stress mitigation strategy when energy air-conditioning is inaccessible and/or prohibitively expensive, for example, in low- and middle-income countries (Lighting Global, GOGLA & ESMAP, Citation2020).
Limitations
No human participants were involved in experimental testing and, therefore, it was not possible to assess the thermo-physiological consequences of the eight stroller configurations. However, the study design afforded well-controlled quantification of the thermal effects of each stroller configuration. Secondly, only one stroller type was tested, and it is therefore possible that the efficacy of the cooling techniques may vary if applied to a stroller of vastly different design and/or material composition. However, the current stroller was selected due to its generic design and similarity to other commercially available infant strollers. Additionally, we acknowledge that we did not exhaust all possible cooling strategies (e.g. incorporation of ice blocks/phase change cooling materials into the stroller configuration), and therefore alternative cooling methods may also be efficacious. The cooling techniques were also only assessed over a 20-min period, so their efficacy remains unknown over an extended duration. Future research is required to examine the efficacy of cooling interventions during prolonged heat-stress exposures and to determine the extent to which these may help mitigate the level of heat stress experienced in the stroller carriage over time. However, when the moist muslin draping was employed, the amount of water remaining in the cloth after 20-min well exceeded the amount of water evaporated (). It is therefore expected that the Muslin + Moist and Muslin + Moist + Fan configurations would have continued to confer cooling benefit for at least an additional 20 min (40-min in total, assuming an evaporation rate of ∼9 g/min). Moreover, the longevity of these simple cooling methods may be extended further by regular re-wetting the cloth using a hand-held water spray bottle. Further research is warranted to elucidate the critical time limits for these infant stroller evaporative cooling interventions—such information may be yielded using experimental methods like those used in the current study or via the use of thermal manikins which have been used previously to assess infant heat stress (Danielsson Citation2004; Lahana et al. Citation2018). However, our recommendation is that future research should prioritise examining the efficacy of stroller cooling interventions with human (infant) participants, enabling the physiological effects of in-stroller cooling to be examined.
As no infants were recruited to this study, the impact of metabolic heat production and insensible water losses (i.e. from the skin and respiratory system) was not assessed and, therefore, it is unknown to what extent this might have altered the in-stroller conditions had an infant been in situ. However, based on estimated resting infant metabolic rates and whole body sweat losses, in conjunction with the high air exchange within the stroller carriage, it is deemed highly unlikely that the in-stroller temperature and/or humidity would have been elevated meaningfully. Additionally, the surface temperatures of the various stroller components were not assessed. As such, it is not possible to determine how the potential for conductive heat transfer between an infant and the stroller might have been impacted by the different stroller configurations. However, we consider the likely role of conductive heat transfer between stroller and infant to be negligible as the stroller carriage was completely shaded from direct solar radiation (either by the canopy or the canopy and cloth covering in combination), thus limiting the heating of the internal stroller surfaces. Furthermore, when positioned within the stroller carriage, the infant is in contact with the seat (i.e. the posterior aspect of the legs and torso), further shielding this material surface from solar radiation. From a human heat balance perspective, it is likely that dry heat exchange would occur primarily via convection, thus, we opted to focus on the measurement of the in-stroller air temperature. The use of thermistors suspended inside the stroller carriage was deemed both appropriate and practical for use in the current experimental set-up due to the shading provided by the stroller canopy (and material coverings), the consistent airflow through the carriage and the relatively stable environmental conditions. Additionally, the use of small thermistors enabled multiple temperature measurements, providing insight into the spatial variation inside the stroller. However, it should be acknowledged that this measurement technique may not be suitable in other settings in which such conditions are not met. For example, when the temperature sensors are exposed to direct solar radiation or when airflow is restricted, the use of a shaded and aspirated dry bulb thermometer in conjunction with a black globe temperature sensor is likely to be a more appropriate measurement tool.
Finally, whilst we opted to use the WBGT index to integrate the key environmental parameters and provide an assessment of the overall level of heat stress, we acknowledge that WBGT is not typically applied to compartment settings—this should be considered when interpreting the differences observed inside the stroller carriage across the various configurations. However, it is important to note that WBGT was not used to inform decision making (i.e. to establish thresholds with which the efficacy of the cooling inventions was assessed) but, instead WBGT was simply used as a composite index to express the combined effects of multiple environmental parameters.
Conclusion
Our findings provide clear empirical evidence in support of the use of a moistened light-coloured muslin cloth draped over the entrance of the stroller carriage to reduce in-carriage infant stroller heat stress. The optimal cooling effect was achieved when a moist muslin draping was coupled with additional forced convection delivered by an inward facing battery-operated fan attached to the stroller frame. In contrast, other widely adopted stroller configurations exacerbated heat stress conditions inside the stroller. For example, the draping of a dry cloth (both muslin and flannelette) substantially worsened the heating of the stroller carriage and should, therefore, be advised against during periods of hot weather to help prevent dangerous levels of in-stroller heat stress.
Acknowledgements
We thank Associate Professor Leigh Wilson for initial insightful conversations regarding parental behaviour and the potential challenges infants face because of different pram coverings.
Disclosure statement
No potential conflict of interest was reported by the author(s).
Correction Statement
This article has been corrected with minor changes. These changes do not impact the academic content of the article.
Additional information
Funding
References
- Alay, S., C. Alkan, and F. Göde. 2012. “Steady-State Thermal Comfort Properties of Fabrics Incorporated With Microencapsulated Phase Change Materials.” Journal of the Textile Institute 103 (7): 757–765. doi:10.1080/00405000.2011.606982.
- Birken, C. S., B. Lichtblau, T. Lenton-Brym, P. Tucker, J. L. Maguire, P. C. Parkin, and S. Mahant. 2015. “Parents’ Perception of Stroller Use in Young Children: A Qualitative Study.” BMC Public Health 15 (1): 808–808. doi:10.1186/s12889-015-1989-6.
- Brand, M. C., and H. A. Shippey. 2021. “Thermoregulation.” In Core Curriculum for Neonatal Intensive Care Nursing, edited by M. T. Verklan, 6th ed., 86–98. St. Louis, MO: Elsevier.
- Bull, Fiona C., Salih S. Al-Ansari, Stuart Biddle, Katja Borodulin, Matthew P. Buman, Greet Cardon, Catherine Carty, et al. 2020. “World Health Organization 2020 Guidelines on Physical Activity and Sedentary Behaviour.” British journal of Sports Medicine 54 (24): 1451–1462. doi:10.1136/bjsports-2020-102955.
- Burnie, R., and C. Howe. 2019. “Pram covers can overheat children inside, safety experts warn.” https://www.abc.net.au/news/2019-01-17/pram-covers-can-overheat-children-safety-experts-warn/10721062
- CDC (Centers for Disease Control and Prevention). 2021. Information for Parents of Infants & Toddlers. https://www.cdc.gov/parents/index.html
- Cramer, M. N., M. Huang, G. Moralez, and C. G. Crandall. 2020. “Keeping Older Individuals Cool in Hot and Moderately Humid Conditions: Wetted Clothing With and Without an Electric Fan.” Journal of Applied Physiology (Bethesda, Md.: 1985) 128 (3): 604–611. doi:10.1152/japplphysiol.00786.2019.
- Currie, J. L., and E. Develin. 2002. “Stroll Your Way to Well-Being: A Survey of the Perceived Benefits, Barriers, Community Support, and Stigma Associated With Pram Walking Groups Designed for New Mothers, Sydney, Australia.” Health care for Women International 23 (8): 882–893. doi:10.1080/07399330290112380.
- Danielsson, U. 2004. “Heat and Mass Transfer from a Baby Manikin: Impact of a Chemical Warfare Protective Bag.” European journal of Applied Physiology 92 (6): 689–693. doi:10.1007/s00421-004-1133-2.
- Elhaik, E. 2019. “Neonatal Circumcision and Prematurity Are Associated With Sudden Infant Death Syndrome (SIDS).” Journal of Clinical and Translational Research 4 (2): 136–151.
- Filingeri, D., H. Cowley, C. Merrick, P. S. Gang, and V. L. Filingeri. 2020. “The Effects of Clothing Layers on the Thermoregulatory Responses to Short Duration Babywearing in Babies Under 12 Months Old.” Physiological reports 8 (9): e14425–n/a. doi:10.14814/phy2.14425.
- Fleming, P. J., R. Gilbert, Y. Azaz, P. J. Berry, P. T. Rudd, A. Stewart, and E. Hall. 1990. “Interaction Between Bedding and Sleeping Position in the Sudden Infant Death Syndrome: A Population Based Case-Control Study.” BMJ (Clinical Research ed.) 301 (6743): 85–89. doi:10.1136/bmj.301.6743.85.
- Foster, J., J. W. Smallcombe, S. Hodder, O. Jay, A. D. Flouris, L. Nybo, and G. Havenith. 2022. “Quantifying the Impact of Heat on Human Physical Work Capacity; Part III: The Impact of Solar Radiation Varies With Air Temperature, Humidity, and Clothing Coverage.” International Journal of Biometeorology 66 (1): 175–188. doi:10.1007/s00484-021-02205-x.
- Foster, K. G., E. N. Hey, and G. Katz. 1969. “The Response of the Sweat Glands of the New Born Baby to Thermal Stimuli and to Intradermal Acetylcholine.” The Journal of Physiology 203 (1): 13–29. doi:10.1113/jphysiol.1969.sp008846.
- Garcia-Souto, M. D. P., and P. Dabnichki. 2016. “Core and Local Skin Temperature: 3–24 Months Old Toddlers and Comparison to Adults.” Building and Environment 104: 286–295. doi:10.1016/j.buildenv.2016.04.016.
- Gardner, S. L., and B. H. Cammack. 2021. “Heat Balance.” In Merenstein & Gardner’s Handbook of Neonatal Intensive Care, edited by S. L. Gardner, 9th ed., 137–164. St. Louis, MO: Elsevier Inc.
- Graham, C., G. P. Lynch, T. English, L. Hospers, and O. Jay. 2021. “Optimal Break Structures and Cooling Strategies to Mitigate Heat Stress During a Rugby League Match Simulation.” Journal of Science and Medicine in Sport 24 (8): 793–799. doi:10.1016/j.jsams.2021.04.013.
- Green, A.C., S.C. Wallingford, and P. McBride. 2011. “Childhood Exposure to Ultraviolet Radiation and Harmful Skin Effects: Epidemiological Evidence.” Progress in Biophysics and Molecular Biology 107 (3): 349–355. doi:10.1016/j.pbiomolbio.2011.08.010.
- Guntheroth, W. G., and P. S. Spiers. 2001. “Thermal Stress in Sudden Infant Death: Is There an Ambiguity With the Rebreathing Hypothesis?” Pediatrics 107 (4): 693–698. doi:10.1542/peds.107.4.693.
- Harpin, V., G. Chellappah, and N. Rutter. 1983. “Responses of the Newborn Infant to Overheating.” Biology of the Neonate 44 (2): 65–75. doi:10.1159/000241698.
- Holmberg, H. 2014. “Swedes risk infants’ lives by covering up prams.” The Local. https://www.thelocal.se/20140711/swedes-risk-infants-lives-by-covering-up-prams
- Hospers, L., J. W. Smallcombe, N. B. Morris, A. Capon, and O. Jay. 2020. “Electric fans: A Potential Stay-at-Home Cooling Strategy During the COVID-19 Pandemic This Summer?” The Science of the Total Environment 747: 141180. doi:10.1016/j.scitotenv.2020.141180.
- Inoue, Y., T. Kuwahara, and T. Araki. 2004. “Maturation-and Aging-Related Changes in Heat Loss Effector Function.” Journal of Physiological Anthropology and Applied Human Science 23 (6): 289–294. doi:10.2114/jpa.23.289.
- Lahana, A., S. Delanaud, R. Erbani, A. Glusko-Charlet, E. Durand, E. Haraux, L. Ghyselen, J. Libert, and P. Tourneux. 2018. “Warming the Premature Infant in the Delivery Room: Quantification of the Risk of Hyperthermia.” Medical engineering & Physics 59: 70–74. doi:10.1016/j.medengphy.2018.06.002.
- Lighting Global, GOGLA, & ESMAP 2020. Off-grid solar market trends report 2020. https://www.lightingglobal.org/wp-content/uploads/2020/03/VIVID%20OCA_2020_Off_Grid_Solar_Market_Trends_Report_Full_High.pdf
- Lynch, G. P., J. D. Periard, B. M. Pluim, J. R. Brotherhood, and O. Jay. 2018. “Optimal Cooling Strategies for Players in Australian Tennis Open Conditions.” Journal of Science and Medicine in Sport 21 (3): 232–237. doi:10.1016/j.jsams.2017.05.017.
- McLaren, C., J. Null, J. Quinn. 2005. “Heat Stress from Enclosed Vehicles: Moderate Ambient Temperatures Cause Significant Temperature Rise in Enclosed Vehicles.” Ergonomics 116 (1): e109–12. doi:10.1542/peds.2004-2368.
- Mitchell, J. B., B. K. McFarlin, and J. P. Dugas. 2003. “The Effect of Pre-exercise Cooling on High Intensity Running Performance in the Heat.” International journal of Sports Medicine 24 (2): 118–124. doi:10.1055/s-2003-38203.
- Morris, N. B., G. K. Chaseling, T. English, F. Gruss, M. F. B. Maideen, A. Capon, and O. Jay. 2021. “Electric Fan Use for Cooling During Hot Weather: A Biophysical Modelling Study.” The Lancet Planetary Health 5 (6): e368–e377. doi:10.1016/S2542-5196(21)00136-4.
- Morris, N. B., F. Gruss, S. Lempert, T. English, L. Hospers, A. Capon, and O. Jay. 2019. “A Preliminary Study of the Effect of Dousing and Foot Immersion on Cardiovascular and Thermal Responses to Extreme Heat.” JAMA 322 (14): 1411–1413. doi:10.1001/jama.2019.13051.
- NHS (National Health Service). 2021. “Keeping your baby safe in the sun”. https://www.nhs.uk/conditions/baby/first-aid-and-safety/safety/safety-in-the-sun/
- NSW Health Government. 2020. Babies and children in the heat. https://www.health.nsw.gov.au/environment/beattheheat/Pages/babies-children-hot-weather.aspx
- O’Callaghan, P. W., and S. D. Probert. 1976. “Thermal Insulation Provided by Dry, Single-Layer Clothing Materials.” Applied Energy 2 (4): 269–277. doi:10.1016/0306-2619(76)90013-1.
- Ravanelli, N. M., S. G. Hodder, G. Havenith, and O. Jay. 2015. “Heart Rate and Body Temperature Responses to Extreme Heat and Humidity With and Without Electric Fans.” JAMA 313 (7): 724–725. doi:10.1001/jama.2015.153.
- Rutter, N., and D. Hull. 1979. “Response of Term Babies to a Warm Environment.” Archives of Disease in Childhood 54 (3): 178–183. doi:10.1136/adc.54.3.178.
- Scipioni, Marco, Edmir Silva, Faramarz Farahi, and Faramarz Farahi. 2020. “Solar Absorption Index (SAI) as a Parameter to Assess the Coolness of Fabrics Exposed to Sunlight.” Fibres and Textiles in Eastern Europe 28 (5(143): 44–49. doi:10.5604/01.3001.0014.2383.
- Sehat, A. R., U. Nirmal, and Z. Jin. 2017. “State of the Art Baby Strollers: Design Review and the Innovations of an Ergonomic Baby Stroller.” Cogent Engineering 4 (1): 1333273. doi:10.1080/23311916.2017.1333273.
- Sulyok, E., E. Jéquier, and L. S. Prod’hom. 1973. “Respiratory Contribution to the Thermal Balance of the Newborn Infant under Various Ambient Conditions.” Pediatrics, 51 (4): 641–650. doi:10.1542/peds.51.4.641.
- Székely, M., and J. Garai. 2018. “Thermoregulation and Age.” Handbook of Clinical Neurology 156: 377–395. doi:10.1016/B978-0-444-63912-7.00023-0.
- Vanos, J. K., A. Middel, M. N. Poletti, and N. J. Selover. 2018. “Evaluating the Impact of Solar Radiation on Pediatric Heat Balance Within Enclosed, Hot Vehicles.” Temperature (Austin, Tex.) 5 (3): 276–292. doi:10.1080/23328940.2018.1468205.
- Wailoo, M. P., S. A. Petersen, and H. Whitaker. 1990. “Disturbed Nights and 3–4 Month Old Infants: The Effects of Feeding and Thermal Environment.” Archives of Disease in Childhood 65 (5): 499–501. doi:10.1136/adc.65.5.499.
- WHO (World Health Organisation). 1997. Thermal Protection of the Newborn: A Practical Guide. Geneva: World Health Organization.