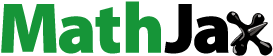
Abstract
Excessive solar radiation negatively affects cognitive performance. Occupational guidelines typically combine environmental components into one value, such as wet-bulb globe temperature (WBGT). Here, we evaluated cognitive performance in two similar 28.6 °C WBGT-effective (WBGTeff) that were designed differently; using high or low levels of solar radiation. Eight soldiers were exposed to a virtual-reality environment in a climate chamber set to high (900 Wm−2) or low solar radiation conditions (300 Wm−2). Soldiers walked 3 x 30 min at 5 kmh−1. Cognitive performance was evaluated using a virtual-reality scenario and a computerised test battery. There was no statistically significant effect of condition on the cognitive tasks (p > 0.05). Associations were found between mean body temperature (Tb) and visual detection (P ≤ 0.01). Differences in solar radiation with similar WBGTeff (28.6 °C) do not cause large systematic differences in cognitive performance. Certain aspects of cognitive performance (i.e. response inhibition) seem to be partly associated with Tb rather than solar radiation.
Practitioner summary: Cognitive performance was evaluated in two similar WBGT conditions that were designed differently; using high or low levels of solar radiation. Differences in solar radiation with similar WBGT do not cause systematic differences in cognitive performance. Certain aspects of cognition were partly associated with mean body temperature rather than solar radiation.
1. Introduction
As global urbanisation keeps increasing, the likelihood for future military operations to occur in urban regions grows simultaneously. Urban regions are characterised by relatively warm urban heat islands (Rizwan, Dennis, and Chunho Citation2008; Pyrgou, Santamouris, and Livada Citation2019; Oke Citation2010). Consequently, military operations in urban regions will be physically more demanding for soldiers. Urban regions also yield more complexity compared to rural regions and, in general, soldiers are exposed to an increasing amount of information by provided technological support. The complexity of urban regions and the increasing information potentially causes a shift towards more cognitively dominated performance for soldiers on foot (Billing et al. Citation2021).
Cognitive performance is known to be negatively affected by heat stress (Gaoua Citation2010; Kingma et al. Citation2020; Doohan Citation2022). Previous research demonstrated that following a severe body core temperature (Tc) elevation (∼2 °C), cognitive performance was deteriorated (Sun et al. Citation2012; Gaoua et al. Citation2011; Hocking et al. Citation2001; Lee et al. Citation2014; Liu et al. Citation2013; Racinais, Gaoua, and Grantham Citation2008; Simmons et al. Citation2008). Tc elevations up to ∼1 °C hamper cognitive performance less (Clarke et al. Citation2017; Gaoua et al. Citation2011; Hocking et al. Citation2001; Lee et al. Citation2014; Liu et al. Citation2013; McMorris et al. Citation2006; Racinais, Gaoua, and Grantham Citation2008; Schlader et al. Citation2013, Citation2015; Schmit et al. Citation2017; Sun et al. Citation2012; Hancock Citation1986; Kingma et al. Citation2020). Cognitive task performance could also be perturbed by skin temperature (Tsk), thermal comfort and thermal sensation without a Tc increase (Gaoua Citation2010; Gaoua et al. Citation2012; Ioannou et al. Citation2021; Gibbons et al. Citation2021). Recently, evidence suggested that high levels of solar radiation (∼800–1000 Wm−2) directed towards the head on itself deteriorated motor-cognitive performance (Piil et al. Citation2020). Further, a framework that highlights that severe hyperthermia or moderate hyperthermia in combination with solar radiation has a notable effect on cognitive performance (Piil et al. Citation2021). Previous research already suggested that different climate types could elicit the same WBGT but differ in terms of heat load (Lotens and Middendorp Citation1986). The authors emphasise the heat load difference in radiant and non-radiant climate types. The potential mechanism behind temperature-related cognitive performance deterioration has to do with vasodilation, which occurs during heat stress to maintain Tc. Due to vasodilation more blood is distributed towards the periphery and less towards the brain, which may explain the aforementioned cognitive performance reductions (Martin et al. Citation2019; Gaoua et al. Citation2012; Brothers et al. Citation2009; Wilson et al. Citation2006; Hancock and Vasmatzidis Citation2003). By direct sun exposure Tsk increases, augmenting the aforementioned process. Likewise, direct sun exposure could cause to discomfort, drawing away attention from the task.
The deployability of military personnel is determined based on occupational guidelines. Occupational guidelines to assess environmental heat stress typically do not do not distinguish between the intensity of solar radiation. Instead, occupational guidelines typically use a single value that represents the overall thermal environment (i.e. air temperature, mean radiant temperature, relative humidity and wind), such as the wet bulb globe temperature (WBGT) (ISO 7243 Citation2017). Based on ranges of WBGT-values, NATO proposed work-rest-ratios and fluid recommendation guidelines depending on the type of activity performed (Spitz et al. Citation2012). For example, having a WBGT of 31.1–32.2 °C whilst deploying a moderate work intensity (metabolic rate of ∼425 W), individuals should adhere to a 30/30 min work-rest-ratio whilst ingesting 0.75 Lh−1 of fluid (Spitz et al. Citation2012).
As future military operations will occur more and more in urban regions where solar radiation is a large contributor of heat stress (Rizwan, Dennis, and Chunho Citation2008; Pyrgou, Santamouris, and Livada Citation2019; Oke Citation2010; Billing et al. Citation2021), it is important to elaborate on the potential separate effects of solar radiation. This is even more relevant as current operational guidelines do not assume a separate effect of solar radiation on (cognitive) performance (ISO 7243 Citation2017). In the present study, we evaluated cognitive task performance in two 28.6 °C WBGT-effective conditions (WBGTeff = WBGT + Clothing Adjustment Value = 25.8 + 2.8) that were designed differently; using high or low solar radiation levels. Whilst in practice similar WBGT-values impose the same practical recommendations, reductions in cognitive performance could differ by solar radiation levels. The 28.6 °C WBGTeff falls in the green flag WBGT category of NATO HFM187 (Spitz et al. Citation2012), and is generally considered as the first (and lowest) level of heat stress, but does already imply work-rest restrictions for moderate to high activity levels. It is not known if the green-flag WBGT condition would elicit a cognitive performance decline per se. Here, we aim to test whether there would already be differences in cognitive performance that are operationally relevant for a military operation; and that may potentially require an adjustment of the guidelines to separately recommend work-rest cycles for physical activity versus cognitive activities. Since cognitive performance is known to be sensitive to solar radiation, and WBGT is an aggregate thermal stress index, we hypothesised that potential cognitive performance deterioration is larger in a high compared to a low solar radiation condition whilst WBGTeff is similar (Piil et al. Citation2021; Ioannou et al. Citation2021; Gibbons et al. Citation2021; Piil et al. Citation2020).
2. Material and methods
2.1. Participants
Experimental procedures were approved by the local Ethics Committee of TNO (2020-127). The study was conducted in accordance with the guidelines of the revised Declaration of Helsinki (2013). Written informed consent and a basic health check by a medical physician were obtained from all participants before participation in the study. Eight male soldiers on active duty (age: 25 ± 4 years; body mass: 76.5 ± 11.8 kg) participated in this study. Contrary to an a priori sample size analysis to detect a difference between two or more (in)dependent means, this study was set up to detect only large effect sizes. The rationale behind this logic is that if the difference cannot be clearly detected in any small group of participants, it may not be important in an operational setting (i.e. military squad size). Given α = 0.05, power = 0.8 and n = 8, we were able to detect a difference between two dependent means once the effect size exceeded 0.98 (which is considered a large effect size). To ensure hydration, participants were instructed to refrain from alcohol and drugs 24 h before the experiment and to consume 500 mL of water 0–2 h before starting the experiment. Participants self-reported that they did not take any prescription medication, had no history of heat-related illnesses or cardiovascular complications and did not have any known issues with thermoregulation.
2.2. Design
Participants reported to the laboratory on two occasions to repeat the exact same procedures in two different environmental conditions (i.e. within-subject design). Environmental conditions were set to either high or low solar radiation but with a similar WBGT of 25.8 °C. The effective WBGT (i.e. WBGTeff) represents an estimate of the heat stress provided by adding a clothing adjustment value (CAV) (WBGTeff = WBGT + CAV). In the present study, we added +2.8 °C to account for the worn clothing configuration (Spitz et al. Citation2012). The consequent WBGTeff of 28.6 °C is considered green-flag (i.e. WBGT range: 27.7–29.4 °C (Spitz et al. Citation2012)). The green flag condition is not considered as hot, but does already imply a work-rest cycle of 50 min/10 min per hour for moderate activity levels, that in a cooler environment would be possible to carry out during a full hour. Two large lamps (each 25 × 1 cm at a height of 2 m, halogen, 220–250 V, 1000 W, 9 J, Philips, Eindhoven) were used to set the high solar radiation condition (). The required solar radiation was determined using Climate CHIP (https://www.climatechip.org/excel-wbgt-calculator). An overview of the WBGT-values from head to feet is shown in Appendix B. By adjusting the relative humidity the water vapour pressure was kept similar (1.57 kPa) between conditions, allowing for an equal capacity to evaporate. The order of conditions was balanced among participants.
Table 1. Characteristics of the high and low solar radiation conditions.
2.3. Protocol
During each occasion, participants walked on a self-propelled treadmill (Bari Mill 55, Woodway, Waukesha, Wisconsin, USA) in a climate chamber (Weiss Technik, Tiel, The Netherlands). Participants intermittently walked three times 30 min at 5 kmh−1 whilst wearing a summer battle dress trousers (Woodland camouflage pattern, 65% polyester, 35% cotton), t-shirt (green, 100% polyester), combat shirt (beige core, 65% polyester, 35% cotton, multi-cam camouflage pattern sleeves, 50% nylon, 50% cotton), helmet and carrying a ∼15 kg backpack (adding up to ∼1.2–1.3 clo). Between the 30-min blocks there were 10 min of rest ().
Figure 1. Schematic overview of the study protocol: alternating 30 min of walking and 10 min of rest. Participants wore a ∼15 kg backpack throughout. Cognitive evaluation took place during both the 30-min walking blocks and 10-min breaks.

Participants were exposed to a virtual-reality urban region using the ‘mobile immersive cognitive environment’ (MICE; ) (Beurden and Roijendijk Citation2019). By walking on the self-propelled treadmill, participants could move through the virtual-reality environment. Participants had to navigate through the streets of an urban scene in West-Central Asia using the mission navigation belt (https://elitacwearables.com/projects/mission-navigation-belt/) (). This belt, worn around the waist, was programmed to vibrate on the left or right side prior (∼10 s) to each crossing at which participants had to change direction. While walking and navigating, participants had to perform three cognitive tasks simultaneously. The first task comprised of participants having to visually detect friends or foes (i.e. 50 per 30 min, wearing a slightly different camouflage pattern) that stood in alleys between buildings in the virtual-reality environment. When seeing a foe, participants had to push a button that was attached to the right handgrip of the treadmill whilst they had to ignore friends. In the second task, participants had to detect high (440 Hz for 500 ms) or low frequency signals (311 Hz for 500 ms) during walking. By hearing the high tone, participants had to push a button that was attached to their left index finger whilst they had to ignore the low tone signal. The third task was a memory task in which participants had to detect and imprint a total of ten billboards that they encountered during each 30-min block in the on buildings next to the route. In the 10-min rest periods, participants had to recall the billboards in the order of appearance by selecting ten billboards from a set of 30 billboards and placing them in the correct order. The pictures on the billboards were selected from the Nencki Affective Picture System (Marchewka et al. Citation2014). Six sets of 10 pictures were selected in this experiment. These were all selected from the categories ‘happiness’ and ‘happiness, surprise’. The selected pictures were then categorised in ten groups of six pictures with similar arousal ratings. Immediately after that, participants conducted the Groton maze learning test (GMLT) (Snyder et al. Citation2005). In this test, the aim is to find a hidden pathway concealed within a 10 × 10 tile grid presented on a computer screen (). Each trial starts in the top left corner and has 28 moves and 11 turns to approach the bottom right corner. Participants find the route bey clicking step-by-step on the adjacent tiles. After each step feedback was provided on whether the step was correct or incorrect. When the step was incorrect, participants continued the task from the last correctly identified tile. When the step was correct, participants received instructions to continue. Participants completed five consecutive trials followed by a 2 min break and a delayed trial.
2.4. Measurements
To confirm hydration, urine specific gravity (USG) was measured with a handheld refractometer (PAL-S, Atago, Bellevue, USA; USG ≤ 1.020). Heart rate (HR) was monitored continuously using a chest strap and watch (OH1 and M430, Polar, Kemele, Finland). As an indication of Tc, gastrointestinal temperature was measured using a telemetric capsule (BodyCap, e-Celcius, Hérouville-Saint-Clair, France) that was ingested an hour before starting the experiment. Tsk sensors (i-Button DS1922L, Maxim Integrated, USA) were attached to four skin locations according to ISO 9886 (ISO 9886 Citation2004) using sticking adhesive (Fixomull stretch, BSN Medical, Almere) and a weighed mean Tsk was calculated (ISO 9886 Citation2004). Mean body temperature (Tb) was calculated using EquationEq. 1(1)
(1) . Cognitive workload was rated on a 5-point scale with continuous values (1 – very high, 2 – high, 3 – average, 4 – low, 5 – very low) (Brennan Citation1992). To ensure hydration throughout the protocol, participants drunk ∼65 mL of water every five minutes (i.e. adding up to ∼0.78 Lh−1 which corresponds to the recommended 0.75 Lh−1 (Spitz et al. Citation2012)). The water temperature was adjusted to the observed Tc (±0.5 °C) (Hardy, Du Bois, and Soderstrom Citation1938).
(1)
(1)
Multiple functions of cognitive performance were evaluated. An overview of the tasks, corresponding cognitive function that was addressed, and the actual cognitive performance measures taken is listed in . No measures were taken for the tactile navigation task as participants all succeeded to successfully navigate through the scenario. For the visual and auditory detection tasks four measures were taken: accuracy, hit rate, false alarm rate and response time. Accuracy was also obtained from the short-term memory task. Lastly, for the spatial working memory task the number of errors and the mean correct moves per second (CMS) were registered and reported. CMS is also known as maze efficiency index ().
Table 2. Cognitive performance measures.
2.5. Statistics
All data were synced and formatted using Matlab (The Mathworks, Natick). HR, Tc, mean Tsk and Tb data were converted into 30-min and 10-min block averages. Statistical analyses were performed using IBM SPSS Statistics 28.0. Physiological, perceptual and cognitive outcomes were assessed using a mixed effect model with condition and block as fixed effects and participant as random effect (i.e. both intercept and slope). The selected covariance structure was variance components. To find out if there were relations (p < 0.05) between physiological and cognitive outcome parameters, one could opt for linear regression analysis. However, in the military it is essential to know what parameters are risk factors (or not) for cognitive performance. Since to our knowledge there is no specific value or percentage of cognitive performance reduction known that should be avoided in a military setting, the group average on each variable was taken as threshold value. To analyse the potential relation between the physiological and cognitive parameters, binary logistical regressions on accumulated data of both solar conditions were conducted. Cognitive performance was considered as the dependent variable and was coded dichotomously (‘0’ denotes result < group average and ‘1’ denotes result > average). Tc, mean Tsk and Tb were factors in the analysis. Non-significant variables were removed from the model in a backwards stepwise manner. Lastly, total thermal load was established by calculating the area under the curve for Tb (EquationEquation 2(2)
(2) ). In this equation, Tb-i is Tb at time point i (°C) and dt is the duration of the thermal stimulus (min).
(2)
(2)
3. Results
3.1. Physiological
For all physiological variables, there was a main effect of block (p < 0.001, ), indicating that there were changes over time. For HR, there was no significant main effect of condition (p = 0.274), neither an interaction effect of condition x block (p = 0.828). Such findings indicate that HR overall did not significantly differ between the high and low solar radiation condition. Neither was the increase in HR over blocks larger in one of the conditions (). Moreover, there was a significant main effect of condition on Tc (p = 0.018), indicating that Tc was higher in the low solar radiation condition. The condition x block interaction on Tc was statistically significant as well (p = 0.039), indicating that the raise in Tc was larger in the low compared to the high solar radiation condition (). Furthermore, there was a significant main effect of condition on mean Tsk (p < 0.001). This indicates that mean Tsk was continuously higher in the high solar radiation condition (). No interaction effects were found for mean Tsk by condition x block (p = 0.811). For Tb there was no statistically significant effect of condition per se (p = 0.510) but the interaction of condition x block of Tb was significant (p = 0.033). Such findings indicate that overall Tb was not higher or lower in one condition but the raise in Tb was larger in the low solar low condition (). The total thermal load for Tb in the high solar radiation condition was 3708°Cmin and 3707°Cmin in the low solar condition (p > 0.05).
Figure 4. Heart rate (HR), core temperature (Tc) and mean skin temperature (mean Tsk) over time. Grey dots show the high solar radiation condition. Black dots show the low solar radiation condition. Light grey rectangles represent the three 30-min walking blocks. * represent a significant (p < 0.05) interaction effect of condition x block. # represent a significant main effect of condition (n = 8).
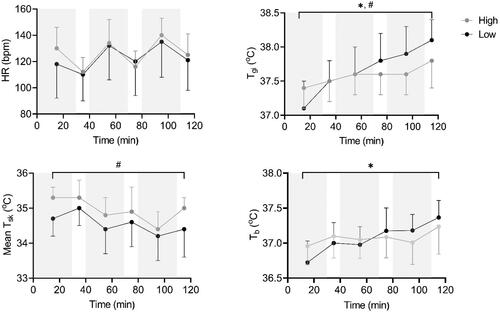
Figure 5. Total thermal load (or area under the curve) for mean body temperature (Tb). Grey dots and the grey filling pattern show the high solar radiation condition. Black dots and the black filling pattern show the low solar radiation condition. Light grey rectangles represent the three 30-min walking blocks (n = 8).
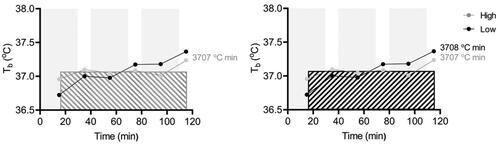
2.2. Cognition
In general, cognitive performance was high on all tasks (visual detection, auditory response, memory and GMLT), with absence of significant condition and condition x block effects on any of the cognitive performance outcomes (p > 0.05). For an overview of all cognitive performance measures in both solar radiation conditions see Appendix A. Further, there were no condition or interaction effects found for experienced cognitive workload. This indicates that the cognitive workload was not perceived differently due to the solar condition and that the decrease in cognitive workload score over time was not larger in one of the solar radiation conditions ().
2.3. Associations
As there was no separate effect of condition on Tb, the two data points per participants (i.e. Tb and cognitive result in both conditions) are included in the following graphs. Two significant relations were observed between physiological and cognitive outcome parameters. Firstly, there was a significant positive relation between Tb and scoring above average on accuracy of the visual detection task (p = 0.019, ). The odds (i.e. probability to occur divided by the probability to not occur) were 6.3, indicating that for a Tb raise from 37 °C to 37.5 °C there was a 6.3 times higher chance of accuracy being less accurate. The probability (i.e. fraction of times expecting to see it in many trials) of this performance decline to occur at Tb = 37.5 °C was 82%. The opposite goes for Tb and scoring above average on false alarm rate of the visual detection task, where a significant negative relation was observed (p = 0.003, ). The odds were 10.5, indicating that for a Tb raise from 37 °C to 37.5 °C there was a 10.5 times higher chance of false alarms being 1 (i.e. more false alarms). The probability of this performance decline to occur at Tb = 37.5 °C was 79%.
Figure 7. Sigmoid curves that show the significant relations between mean body temperature (Tb) and scoring below (0) or above average (1) on false alarm rate (%) and accuracy (%) of the visual detection task. 0 denotes a score below average (i.e. disadvantageous in terms of accuracy but beneficial in terms of false alarms). 1 denotes a score below average. The corresponding regression equation and p-values are shown in the graphs.
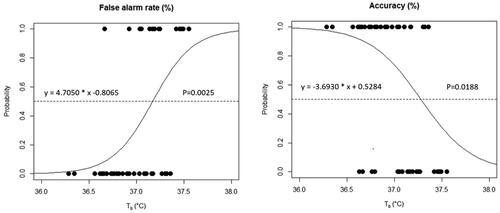
3. Discussion
The aim of the present study was to evaluate cognitive performance in two green-flag (28.6 °C) WBGTeff conditions that were designed differently; using high or low solar radiation levels (900 Wm−2 and 300 Wm−2 respectively). We found that differences in solar radiation with similar WBGT may not cause large systematic differences in cognitive performance; but there were significant associations to performance decline dependent on the level of individual heat strain. This finding supports the theory that cognitive performance deterioration is dependent on the total heat load, which was similar in both solar conditions (i.e. 3707°Cmin in high and 3708°Cmin in low). Because Tc was higher in the low solar radiation condition and mean Tsk was higher in the high solar radiation condition, it seems that the body has distributed heat differently between conditions. Only certain aspects of cognitive performance (i.e. attention and inhibition) seem to be partly associated with Tb rather than solar radiation during relative low (green flag) heat stress levels.
4.1. Physiological
First and foremost, it is essential to elaborate on the physiological responses that occurred in the present study. Where HR was unaffected by the conditions, Tc increased more with low solar radiation. The larger raise in Tc is probably due to the higher environmental temperature in that condition (32 °C and 23 °C). The relatively high environmental temperature causes a relatively small air-to-skin temperature ratio, restricting conductive and convective heat loss. The higher Tc and larger Tc raise in the low solar radiation (but higher environmental temperature) condition could be caused by the restricted conductive heat loss potential. The direct effect of solar radiation on human skin was observed in the present study as a continuously higher mean Tsk in the high compared to the low solar radiation condition. When combining Tc and mean Tsk in one single parameter, Tb, we found that the Tb increase in the low solar radiation condition was higher compared to the high solar radiation condition. However, baseline Tb was slightly higher in the high solar radiation condition and increased less. This resulted in a similar total heat load ().
4.2. Cognition
There were no statistical effects of condition on any of the cognitive outcome measures (Appendix A). An explanation for this finding could be that the total thermal load for Tb was similar between conditions and Tb is known to negatively affect cognitive performance (Martin et al. Citation2019; Gaoua et al. Citation2012; Brothers et al. Citation2009; Wilson et al. Citation2006; Hancock and Vasmatzidis Citation2003). Scores on cognitive outcomes measures were in general relatively high. This could imply that the participants were able to cope well with the situation, preventing a considerable cognitive performance reduction. Another possibility could be that the Tc raise was insufficient to actually deteriorate cognitive performance. Previous research reported considerable cognitive performance reduction when Tc increased by 2 °C (Sun et al. Citation2012; Gaoua et al. Citation2011; Hocking et al. Citation2001; Lee et al. Citation2014; Liu et al. Citation2013; Racinais, Gaoua, and Grantham Citation2008; Simmons et al. Citation2008). The effect was less in situation with a 1 °C Tc increase. In the present study, the reported Tc increase was up to 1 °C. In relation to this, another study found that there was no effect of solar radiation on cognition in neutral conditions (20 °C WBGT) (Ioannou et al. Citation2021). The physiological responses of their 20 °C WBGT condition (high solar: Tc ∼37.6 °C and Tsk ∼34.5 °C; low solar: Tc ∼37.6 °C and Tsk ∼34.3 °C) were relatively similar to the present study (high solar: Tc ∼37.6 °C and Tsk ∼35.0 °C; low solar: Tc ∼37.6 °C and Tsk ∼34.6 °C). It might therefore be that in such environmental conditions the thermal stimulus was not sufficient to elicit a cognitive performance reduction on group level per se. The aforementioned study did find a cognitive performance reduction in a hot condition (30 °C WBGT) (Ioannou et al. Citation2021). Corresponding physiological responses in the high solar (Tc ∼38.3 °C, Tsk 38.5 °C) and low solar radiation condition (Tc ∼38.1 °C, Tsk 36.8 °C) considerably exceeded the present study. Whilst Tc did not differ much between their high and low solar radiation condition, the ∼3 °C higher Tsk stands out and might have caused (part of their) cognitive performance reduction. It could also be that the worn helmet reduced radiative exposure of the head. In a recent study (Piil et al. Citation2020) where a direct effect of sun exposure was found, indeed participants did not wear any type of hat. On the other hand, the military helmet is highly insulative and limits the ability to dissipate heat. Likewise, as the clothing ensemble covered almost the entire body surface (i.e. except from the hands, part of the neck and face) this could have been protective against the impact of solar radiation on cognitive performance. Further, based on logistic regressions we found that Tb is a risk factor for the attention and inhibition aspects of cognitive performance (i.e. as elucidated by the visual detection task). This finding is in agreement with previous research (Hancock Citation1986; Doohan Citation2022; Schmit et al. Citation2017; Hancock and Vasmatzidis Citation2003). It could be that the visual detection task was mostly affected due to distraction as this task was carried out during the scenario. Other tasks such as the GMLT where carried out during breaks. However, for instance the auditory response task was also performed during walking and was unaffected. Therefore, it remains unclear why only this aspect of cognitive performance were affected.
Interestingly, the cognitive workload scores did not differ due to solar radiation. The cognitive scores varied between ∼3 (‘average’) and ∼4 (‘low’) in both conditions. During the last experimental blocks, scores of experienced cognitive workload seemed higher (i.e. less experienced cognitive workload) in the high solar radiation condition. However, this difference was not statistically significant. Previous research suggested a hypothetical relation between (cognitive) task demands, workload and effort (Veltman and Jansen Citation2003). Their model shows that an inverted-U relation can be observed between task demands and performance in a task situation depending on the workload. At the moderate or high levels of workload, performance can still be unchanged but the extra effort that was put in could have been significantly increased. In relation to the present study, cognitive performance was not affected by high or low solar radiation at a certain workload. According to the model presented, it could however also be that the effort that was put in the task to sustain the optimal performance differed between conditions.
4.3. Practical implication
Based on the findings in the present study, there does not seem to be reason to adjust WBGT guidelines for the military regarding cognitive performance in a green-flag WBGTeff (28.6 °C) condition. Even though body heat was likely distributed differently (i.e. towards the core or the periphery), the total thermal load may have the most impact on cognitive performance. Future research should confirm such suggestions and, more importantly, should focus on the effects of solar radiation with higher corresponding WBGT-values (i.e. orange, red, and black flag).
4.4. Limitations
A limitation to the present study could be that we evaluated cognitive performance in a virtual-reality rather than a real life setting. The most common way to evaluate cognitive performance is using computer based tasks. Due to the nature of military employment it is not ecologically valid to evaluate cognitive performance on a computer. By using the virtual-reality environment and selecting cognitive tests that are relevant for military field work, we have tried to reach a real life setting in a controllable way. It could be that real-life situations would lead to different results. On the other hand, using the virtual-reality environment computer based tasks were brought closer to reality. This could be considered a strength as well. In addition to the virtual-reality setting rather that a real life setting for cognitive evaluation, the environmental conditions were also a representation from reality (i.e. climate chamber rather than outside in the field). It could be that there are separate effects of wind and ultraviolet radiation, for example, which have not been considered here. In brief, it is recommended that the translation of such findings to reality will be verified. Another limitation could be that the complexity of the cognitive tasks was not high enough to show differences in cognitive performance. The relatively high cognitive scores would support this theory. However, there is no measurement that confirms such ideas. Lastly, it would have been interesting to evaluate the effect of solar radiation on cognition in women too. As the distribution of heat across the body varies as a function of gender, the physiological and cognitive outcomes may be different in women. On the other hand, we suggested that total thermal load rather than heat distribution determines cognitive performance. If true, the gender differences in this experiment may be negligible. Future research should clarify this. Lastly, whilst the number of participants in this study (n = 8) is a relevant amount for the military (i.e. in agreement with military squad size), for research purposes it would be good to include more participants in future research.
4.5. Conclusions
Differences in solar radiation (900 Wm−2 and 300 Wm−2) with similar green-flag effective WBGT (28.6 °C) do not cause large systematic differences in cognitive performance. Certain aspects of cognitive performance (i.e. response inhibition and spatial working memory) seem to be partly associated with mean body temperature rather than solar radiation.
Acknowledgements
We would like to thank the participants who dedicated their time to this research.
Disclosure statement
No potential conflict of interest was reported by the author(s).
Additional information
Funding
References
- Beurden, Maurice van, and Linsey Roijendijk. 2019. “Towards a Mixed Reality Platform for Applied Cognitive Load Evaluation.” International Conference on Human-Computer Interaction, 123–136. Springer.
- Billing, Daniel C., Graham R. Fordy, Karl E. Friedl, and Henriette Hasselstrøm. 2021. “The Implications of Emerging Technology on Military Human Performance Research Priorities.” Journal of Science and Medicine in Sport 24 (10): 947–953. doi:10.1016/j.jsams.2020.10.007.
- Brennan, S.D. 1992. “An Experimental Report on Rating Scale Descriptor Sets for the Instantaneous Self Assessment (ISA) Recorder.” Portsmouth: DRA Maritime Command and Control Division. DRA Technical Memorandum (CAD5) 92017.
- Brothers, R.Matthew, Jonathan E. Wingo, Kimberly A. Hubing, and Craig G. Crandall. 2009. “The Effects of Reduced End‐Tidal Carbon Dioxide Tension on Cerebral Blood Flow during Heat Stress.” The Journal of Physiology 587 (Pt 15): 3921–3927. doi:10.1113/jphysiol.2009.172023.
- Clarke, Neil D., Michael J. Duncan, Mike Smith, and Joanne Hankey. 2017. “Pre-Cooling Moderately Enhances Visual Discrimination during Exercise in the Heat.” Journal of Sports Sciences 35 (4): 355–360. doi:10.1080/02640414.2016.1164885.
- Doohan, M. 2022. “Does Increased Core Temperature Alter Cognitive Performance during Exercise-Induced Heat Stress? A Review.” International Conference on Environmental Ergonomics, Niagara.
- Gaoua, N., S. Racinais, J. Grantham, and F. El Massioui. 2011. “Alterations in Cognitive Performance during Passive Hyperthermia Are Task Dependent.” International Journal of Hyperthermia 27 (1): 1–9. doi:10.3109/02656736.2010.516305.
- Gaoua, N. 2010. “Cognitive Function in Hot Environments: A Question of Methodology.” Scandinavian Journal of Medicine & Science in Sports 20: 60–70. doi:10.1111/j.1600-0838.2010.01210.x.
- Gaoua, N., J. Grantham, Sebastien Racinais, and F. El Massioui. 2012. “Sensory Displeasure Reduces Complex Cognitive Performance in the Heat.” Journal of Environmental Psychology 32 (2): 158–163. doi:10.1016/j.jenvp.2012.01.002.
- Gibbons, Travis D., Philip N. Ainslie, Kate N. Thomas, Luke C. Wilson, Ashley P. Akerman, Joseph Donnelly, Holly A. Campbell, and Jim D. Cotter. 2021. “Influence of the Mode of Heating on Cerebral Blood Flow, Non‐Invasive Intracranial Pressure and Thermal Tolerance in Humans.” The Journal of Physiology 599 (7): 1977–1996. doi:10.1113/JP280970.
- Hancock, Peter A. 1986. “Sustained Attention under Thermal Stress.” Psychological Bulletin 99 (2): 263–281. doi:10.1037/0033-2909.99.2.263.
- Hancock, Peter A., and Ioannis Vasmatzidis. 2003. “Effects of Heat Stress on Cognitive Performance: The Current State of Knowledge.” International Journal of Hyperthermia 19 (3): 355–372. doi:10.1080/0265673021000054630.
- Hardy, James D., Eugene F. Du Bois, and G F. Soderstrom. 1938. “Basal Metabolism, Radiation, Convection and Vaporization at Temperatures of 22 to 35 C. Six Figures.” The Journal of Nutrition 15 (5): 477–497. doi:10.1093/jn/15.5.477.
- Hocking, Chris, Richard B. Silberstein, Wai Man Lau, Con Stough, and Warren Roberts. 2001. “Evaluation of Cognitive Performance in the Heat by Functional Brain Imaging and Psychometric Testing.” Comparative Biochemistry and Physiology. Part A, Molecular & Integrative Physiology 128 (4): 719–734. doi:10.1016/s1095-6433(01)00278-1.
- Ioannou, L., L. Tsoutsoubi, K. Mantzios, G. Gkikas, J. Piil, P. Dinas, S. Notley, G. Kenny, L. Nybo, and A. Flouris. 2021. “The Impacts of Sun Exposure on Worker Physiology and Cognition: Multi-Country Evidence and Interventions.” International Journal of Environmental Research and Public Health 18 (14): 7698. doi:10.3390/ijerph18147698.
- ISO 7243. 2017. “International Organization for Standardization (2017). Ergonomics of the Thermal Environment—Assessment of Heat Stress Using the WBGT (Wet Bulb Globe Temperature) Index (ISO Standard No. 7243:2017).”
- ISO 9886. 2004. “International Organization for Standardization (2004). Ergonomics—Evaluation of Thermal Strain by Physiological Measurements (ISO Standard No. 9886:2004).”
- Kingma, Boris R.M., Linsey M.M. Roijendijk, Leendert Van Maanen, Hedderik Van Rijn, and Maurice H.P.H. Van Beurden. 2020. “Time Perception and Timed Decision Task Performance during Passive Heat Stress.” Temperature 8 (1): 53–63. doi:10.1080/23328940.2020.1776925.
- Lee, Jason K.W., Aldrich C.H. Koh, Serene X.T. Koh, Glen J.X. Liu, Amanda Q.X. Nio, and Priscilla W.P. Fan. 2014. “Neck Cooling and Cognitive Performance following Exercise-Induced Hyperthermia.” European Journal of Applied Physiology 114 (2): 375–384. doi:10.1007/s00421-013-2774-9.
- Liu, Kai., Gang Sun, Bo Li, Qingjun Jiang, Xiao Yang, Min Li, Li Li, Shaowen Qian, Lun Zhao, Zhenyu Zhou, Karen M. von Deneen, and Yijun Liu. 2013. “The Impact of Passive Hyperthermia on Human Attention Networks: An FMRI Study.” Behavioural Brain Research 243: 220–230. doi:10.1016/j.bbr.2013.01.013.
- Lotens, W.A., and H. Middendorp. 1986. How Well Does WBGT Predict Heat Strain? Estimates from a Mathematical Model. Soesterberg: TNO Institute for Perception.
- Marchewka, Artur, Łukasz Zurawski, Katarzyna Jednoróg, and Anna Grabowska. 2014. “The Nencki Affective Picture System (NAPS): Introduction to a Novel, Standardized, Wide-Range, High-Quality, Realistic Picture Database.” Behavior Research Methods 46 (2): 596–610. doi:10.3758/s13428-013-0379-1.
- Martin, Kristy, Emily McLeod, Julien Périard, Ben Rattray, Richard Keegan, and David B. Pyne. 2019. “The Impact of Environmental Stress on Cognitive Performance: A Systematic Review.” Human Factors 61 (8): 1205–1246. doi:10.1177/0018720819839817.
- McMorris, Terry, Jon Swain, Marcus Smith, Jo Corbett, Simon Delves, Craig Sale, Roger C. Harris, and Julia Potter. 2006. “Heat Stress, Plasma Concentrations of Adrenaline, Noradrenaline, 5-Hydroxytryptamine and Cortisol, Mood State and Cognitive Performance.” International Journal of Psychophysiology 61 (2): 204–215. doi:10.1016/j.ijpsycho.2005.10.002.
- Oke, Timothy R. 2010. “Urban Heat Islands.” In The Routledge Handbook of Urban Ecology, edited by Ian Douglas, P. M. L. Anderson, David Goode, Michael C. Houck, David Maddox, Harini Nagendra, and Puay Yok Tan, 144–155. Boca Raton, FL: Routledge.
- Piil, J., L. Christiansen, N. Morris, C. Mikkelsen, L. Ioannou, A. Flouris, J. Lundbye-Jensen, and L. Nybo. 2020. “Direct Exposure of the Head to Solar Heat Radiation Impairs Motor-Cognitive Performance.” Scientific Reports 10 (1): 1–10. doi:10.1038/s41598-020-64768-w.
- Piil, J., B. Kingma, N. Morris, L. Christiansen, L. Ioannou, A. Flouris, and L. Nybo. 2021. “Proposed Framework for Forecasting Heat-Effects on Motor-Cognitive Performance in the Summer Olympics.” Temperature 8 (3): 262–283. doi:10.1080/23328940.2021.1957367.
- Pyrgou, Andri, Mattheos Santamouris, and Iro Livada. 2019. “Spatiotemporal Analysis of Diurnal Temperature Range: Effect of Urbanization, Cloud Cover, Solar Radiation, and Precipitation.” Climate 7 (7): 89. doi:10.3390/cli7070089.
- Racinais, S., N. Gaoua, and J. Grantham. 2008. “Hyperthermia Impairs Short‐Term Memory and Peripheral Motor Drive Transmission.” The Journal of Physiology 586 (19): 4751–4762. doi:10.1113/jphysiol.2008.157420.
- Rizwan, Ahmed Memon, L.Y.C. Dennis, and Liu Chunho. 2008. “A Review on the Generation, Determination and Mitigation of Urban Heat Island.” Journal of Environmental Sciences 20 (1): 120–128. doi:10.1016/s1001-0742(08)60019-4.
- Schlader, Zachary J., Daniel Gagnon, Amy Adams, Eric Rivas, C. Munro Cullum, and Craig G. Crandall. 2015. “Cognitive and Perceptual Responses during Passive Heat Stress in Younger and Older Adults.” American Journal of Physiology. Regulatory, Integrative and Comparative Physiology 308 (10): R847–54. doi:10.1152/ajpregu.00010.2015.
- Schlader, Zachary J., Rebekah A.I. Lucas, James Pearson, and Craig G. Crandall. 2013. “Hyperthermia Does Not Alter the Increase in Cerebral Perfusion during Cognitive Activation.” Experimental Physiology 98 (11): 1597–1607. doi:10.1113/expphysiol.2013.074104.
- Schmit, Cyril, Christophe Hausswirth, Yann Le Meur, and Rob Duffield. 2017. “Cognitive Functioning and Heat Strain: Performance Responses and Protective Strategies.” Sports Medicine 47 (7): 1289–1302. doi:10.1007/s40279-016-0657-z.
- Simmons, Shona E., Brian K. Saxby, Francis P. McGlone, and David A. Jones. 2008. “The Effect of Passive Heating and Head Cooling on Perception, Cardiovascular Function and Cognitive Performance in the Heat.” European Journal of Applied Physiology 104 (2): 271–280. doi:10.1007/s00421-008-0677-y.
- Snyder, Peter J., Martin M. Bednar, Jennifer R. Cromer, and Paul Maruff. 2005. “Reversal of Scopolamine-Induced Deficits with a Single Dose of Donepezil, an Acetylcholinesterase Inhibitor.” Alzheimer’s.” Alzheimer’s & Dementia 1 (2): 126–135. doi:10.1016/j.jalz.2005.09.004.
- Spitz, M., J. Castellani, B. Freund, and M. Sawka. 2012. “Management of Heat and Cold Stress Guidance to NATO Medical Personnel.” https://apps.dtic.mil/sti/pdfs/ADA571324.pdf
- Sun, Gang, Xiao Yang, Qingjun Jiang, Kai Liu, Bo Li, Li Li, Lun Zhao, and Min Li. 2012. “Hyperthermia Impairs the Executive Function Using the Attention Network Test.” International Journal of Hyperthermia 28 (7): 621–626. doi:10.3109/02656736.2012.705217.
- Veltman, Hans J.A., and Chris Jansen. 2003. “Consequences for Adaptive Automation.” Operator Functional State: The Assessment and Prediction of Human Performance Degradation in Complex Tasks 355: 249.
- Wilson, Thad E., Jian Cui, Rong Zhang, and Craig G. Crandall. 2006. “Heat Stress Reduces Cerebral Blood Velocity and Markedly Impairs Orthostatic Tolerance in Humans.” American Journal of Physiology. Regulatory, Integrative and Comparative Physiology 291 (5): R1443–48. doi:10.1152/ajpregu.00712.2005.
Appendix A
Figure A1. Response time, hit rate, false alarm rate and accuracy on the visual detection task. Grey dots show the high solar radiation condition. Black dots show the low solar radiation condition (n = 8).
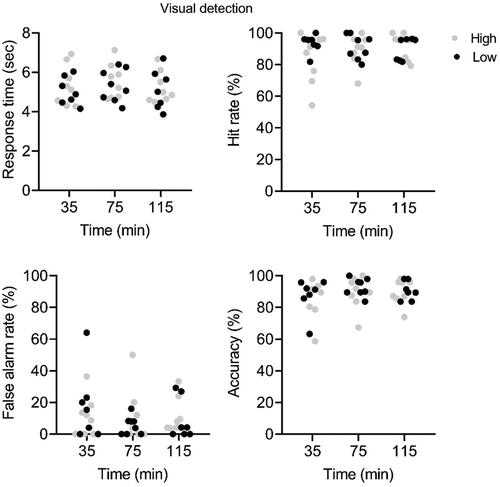
Figure A2. Response time, hit rate, false alarm rate and accuracy on the auditory response task. Grey dots show the high solar radiation condition. Black dots show the low solar radiation condition (n = 8).
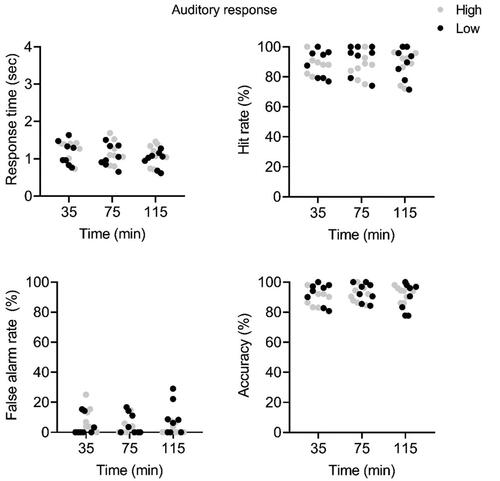
Figure A3. Mean correct moves per second (CMS) and number of errors on the Groton maze learning test (GMLT). Grey dots show the high solar radiation condition. Black dots show the low solar radiation condition (n = 8).
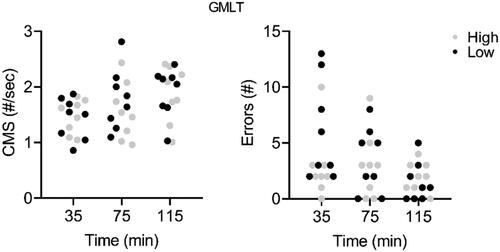
Figure A4. Number of correct pictures on the memory task. Grey dots show the high solar radiation condition. Black dots show the low solar radiation condition (n = 8).
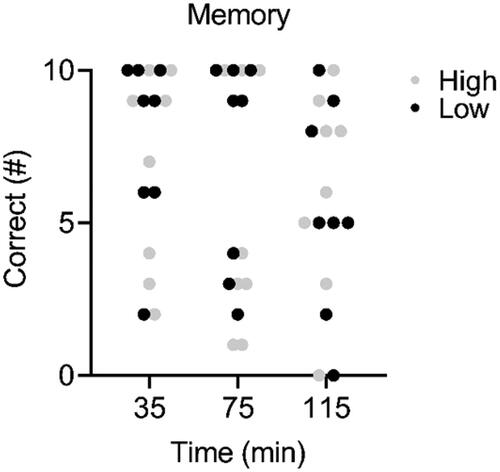
Appendix B