Abstract
We compared two >20 year long data sets of airborne and deposited pollen from northern Finnish Lapland (Kevo) and the middle boreal forest zone (Oulu) in terms of the plant taxa represented and their annual pollen quantities. Tree pollen (Betula spp., Pinus sylvestris) made up 92.8% of the total annual pollen in the air samples at Kevo, while in the ground‐level deposition samples the tree pollen fraction was 61.5%; the remaining 38.5% comprising pollen of ground and field level plants (Ericaceae, Cyperaceae and others). In Oulu, the proportion of tree pollen in the air and deposition samples was 90.6% and 89.0%, respectively. The annual fluctuations in the quantity of total pollen and tree pollen in both sampling systems were correlated, but no such correlation was detected for the low growing plants, except in the case of Urticaceae at Kevo. This suggests that pollen of these low‐growing plants mainly reflects the vegetation of the sampling site. Because tree pollen rises well into the atmosphere, it reflects pollen production from a larger area. Long‐range transported Betula spp. pollen recorded in the atmosphere prior to local flowering comprised a considerable part of the Betula spp. pollen at Kevo and was collected by both sampling systems.
Pollen preserved in sediments provides a record of past vegetation and, through that, climate. At an annual temporal resolution, tree pollen deposited on a sediment surface reflects pollen production of that year and, at high latitudes, this is correlated with the temperature of the growing season of the year prior to pollen emission (Autio & Hicks, Citation2004). Long‐term average pollen accumulation rates (PARs, pollen grains cm−2 year−1), on the other hand, appear to reflect the abundance (biomass) of plants surrounding the deposition site (Hicks, Citation2001).
The characteristics of pollen entrainment to the atmosphere and its subsequent deposition vary between taxa in accordance with numerous factors; including pollen size, weight and morphology, the height and pollination ecology of the plants producing the pollen and the atmospheric conditions affecting pollen release and dispersal (Faegri & Iversen, Citation1989; Sugita et al., Citation1999). Furthermore, in sub‐arctic regions where the trees are at their ecological limit, the quantity of pollen they produce may be small, so that pollen from the same species but originating in distant and more favourable areas (here called ‘long‐range transported’) can contribute significantly to the pollen present in the atmosphere and thus also to the pollen deposited. This is verified for birch pollen in northern Europe (Hicks et al., Citation1994; Hjelmroos, Citation1991; Ranta et al., Citation2006) and also for other tree pollen types, e.g. Pinus sylvestris (Lindgren et al., Citation1995). Even the pollen of some low growing anemophilous plants can be long‐range transported (Ranta & Pessi, Citation2006).
A comparison of pollen in air samples and deposition samples can provide useful empirical data about the dispersal of different pollen types and the occurrence of long‐range transported pollen in deposition samples. This information is useful for determining the source area of deposited pollen. Levetin et al. Citation(2000) provide details of pollen gathered with a volumetric air sampler (Burkard) and a deposition sampler (Tauber) in a warm temperate region with year round pollen emission. Both instruments were placed side by side on a roof top at a height of 12 m above the ground. The taxa and pollen quantities were compared on a monthly basis during a one year sampling period. The comparison shows a strong correlation between monthly pollen accumulation in the Tauber trap and the cumulative sum of average daily airborne pollen concentrations. The dominant taxa recorded by both methods are the same, and represent the anemophilous vegetation of the region. The conclusion from this study is that both methods are equally effective in estimating the regional prevalence on a yearly time scale. In this study, however, neither sampler collected pollen at ground level which would be the situation comparable with a mire/lake surface, the situation which has much greater relevance for palaeoecolgical studies.
We compared long term data sets collected by two methods 1) a Burkard continuous volumetric air sampler collecting airborne pollen and 2) a modified Tauber trap, collecting pollen as it is deposited at a sediment surface, and we compared results at two sites in northern Finland. In contrast to the Levetin et al. Citation(2000) study, the Burkard and Tauber traps did not stand side by side, but at different heights above the ground surface and at different distances from each other. Our overall objectives were to investigate which plant taxa were represented and the proportions of their pollen types in each sampler, and to look at the degree of correlation of inter‐annual variation in pollen quantities (total pollen and individual taxa) between the two types of sampler. With these comparisons we wanted to study whether the pollen of different plant types (for example trees, shrubs, grasses) becomes effectively entrained into the atmosphere, or whether it is mainly deposited near its source. In the first case pollen should be present in both samplers and the inter‐annual fluctuations should be synchronous. In the latter case the pollen type should be more abundant in the deposition sampler. The observations by Levetin et al. Citation(2000) gave us confidence to compare the two types of sampler.
We also considered the significance of Betula spp. and Pinus sylvestris pollen that originates from non‐local sources. By including phenological observations, we are able to distinguish the quantity of pollen present in the air before the onset of local flowering. We call this pre‐flowering pollen. Our hypothesis was that if the pre‐flowering pollen is equally recorded by both samplers then the inter‐annual variations in pollen quantities in the two sampler types will be synchronous.
Material and methods
Study locations
Annual pollen deposition, daily airborne pollen and phenological observations were analysed from two sites in northern Finland: Kevo in northern Finnish Lapland (Utsjoki municipality) and Oulu (the city of Oulu and surrounding municipalities) at the coast of the Gulf of Bothnia (Figure ).
Kevo
– Both the Tauber trap and the Burkard volumetric air sampler were located in the vicinity of the Kevo Subarctic Research Station (69°45′N, 27°01′E) in Utsjoki (Finnish Lapland), which lies in the Northern Boreal Zone (Hämet‐Ahti, Citation1988). The undulating landscape is characterised by mountain birch (Betula pubescens ssp. czerepanovii), mires and treeless fells (Heikkinen & Birks, Citation1996). Although situated north of the continuous Scots pine forests, many Pinus sylvestris individuals are found close to the Kevo river on the steep slopes of the valley. The Burkard sampler collecting airborne pollen was situated 5 m above the ground on the roof top of a low building (the altitude at this point is 102 m a.s.l.) surrounded by mountain birch and Scots pine with an under‐storey of Ericaceous dwarf shrubs and grasses. The Tauber trap, in contrast, was sunk into a Sphagnum tussock on a small mire (75×100 m). This mire is ca. 1.3 km to the south of the Burkard site and at a higher altitude (182 m a.s.l.). The vegetation of the mire consisted of species of Cyperaceae and Ericales plus Betula nana. The Tauber trap is, therefore, 80 m above the Burkard in terms of altitude but the Burkard is 5 m higher up in the air than the Tauber.
The climate of the region shows a continental influence, with a mean annual precipitation of ca. 395 mm (30–40% as snow) and mean annual temperatures of −2°C, whereas maximum temperatures reach 32.9°C in July and minimum Winter temperatures can fall to −47.9°C (Finnish Meteorological Institute, Citation1991). Snow depth measurements are available for a site within 20 m of the Burkard sampler (measurements daily at 9.00 a.m.). The growing season (temperatures above 5°C) from June to August comprises 110–120 days (Heikkinen, Citation1998). The most common wind directions during the flowering season (March–May) are from the south and south–west.
Oulu
– The Oulu area, at the coast of the Gulf of Bothnia, lies within the middle boreal zone (Hämet‐Ahti, Citation1988), and is some 530 km south of Kevo. The regional vegetation is dominated by Pinus sylvestris forests with an admixture of Picea abies (Norwegian spruce). The Burkard sampler is located at the University of Oulu (65°04′N, 25°31′E; 25 m a.s.l.), on the roof of a building 15 m above ground level. The surrounding vegetation is patchy pine forest which is interspersed between urban developments. The Tauber trap used in the comparison is located in the centre of the island Hailuoto (65°01′N, 24°43′E; 6 m a.s.l.), which is ca. 35 km west of the Burkard sampler. The island, 200 km2 in size, is characterised by sand dune vegetation, mires, wet meadows, fields and forests. Pine forests dominate, occurring on the higher western and northern parts of the island, but birch forests are also common and, on more moist sites, these are admixed with Alnus and Salix (Colpaert, Citation1993). The Tauber trap is at ground level and located actually within pine forest with some influence of field and mire vegetation. The Burkard trap is, therefore, both further east than the Tauber, at a higher altitude and also much higher in the air.
The annual mean temperature (1961–1990) in Oulu is +2°C, the absolute maximum +32.4°C and the absolute minimum −41.5°C. Precipitation is 512.2 mm, the snow cover can reach 48 cm and the growing season lasts for 150 days (Väre et al., Citation2005). The climate of Hailuoto is slightly cooler, but even dryer (446.7 mm) than in Oulu. As at Kevo, the highest temperatures are reached in July and the lowest in February. The wind direction at the time of flowering is also the same as at Kevo, i.e. predominantly from the south and south–west (climate data from the Finnish Meteorological Institute, Citation1991).
Pollen Data: Air samples
We used the time series of annual accumulated pollen sums using the Burkard spore‐trap (Hirst, Citation1952), counted as the sum of the daily average pollen counts (pollen grains m−3 air) for the years 1982–2006 at Kevo and 1981–2006 at Oulu. The technique of volumetric trapping is a standard procedure throughout Europe (British Aerobiology Federation, Citation1995). Pollen grains were counted and identified on randomised fields observed under the microscope (Mäkinen, Citation1981). Pollen identification was to the level of genus or family. Neither the Betula nor the Ericaceae pollen data were differentiated as to species.
The contribution of pre‐flowering Betula spp. and Pinus sylvestris pollen was determined as the airborne pollen measured prior to the observed onset of local flowering with no reference to the distance from source. We use the term long‐range transport to refer to separate pollen dispersal episodes that occurred several weeks before the onset of local flowering and must, therefore, originate from sources further away than the normal mean distance (Nathan et al., Citation2003), in our case hundreds of kilometres, in contrast to regional (tens of kilometres) and local sources (within the close vicinity, up to a few kilometres). Long‐range transport episodes of Betula in Kevo in the years 2000 and 2002, and long‐range transport episodes of Betula and Pinus in Kevo in 2002, are considered in this study.
Pollen Data: Deposition samples
To collect the pollen deposited on the mire surface we used a modified Tauber trap (Tauber, Citation1974; Hicks & Hyvärinen, Citation1986) with a 5 cm diameter opening, a common method for obtaining annual pollen accumulation rate data (PAR: pollen grains cm−2 year−1) following the Pollen Monitoring Programme guidelines (Hicks et al., Citation1996, Citation1999). The trap remained in the field for the entire year and was emptied annually at the end of the flowering season (beginning of September). Pollen was identified to a lower taxonomic resolution than in the Burkard samples, e.g. Betula nana, B. pubescens and, B. pubescens ssp. czerepanovii are separately distinguished (Terasmäe, Citation1951). To allow comparison with the Burkard sample data these taxa were summed, as were the individually identified genera of Ericaceae and Empetraceae (Vaccinium, Calluna, Casiope, Ledum and Empetrum). The deposition data at Kevo is for the years 1982–2004 and at Oulu for the years 1981–2004 (ie. no data for the years 2001 and 2002).
Phenological observations
Phenological observations for just Betula and Pinus are for the years 1997–2006. The starting dates for male flowering of Betula pubescens ssp. czerepanovii and Pinus sylvestris at the northern site are from the Kevo Subarctic Research Station. For the southern site, however, the nearest available place for observations of Pinus sylvestris male flowering and Betula pendula bud break was the Muhos Research Station which is ca. 37 km south–east of Oulu and at an altitude of 25 m a.s.l. Temperatures in Muhos are slightly lower than in Oulu and Hailuoto, but precipitation is comparable to Oulu (Finnish Meteorological Institute, Citation1991). Leaf bud burst data was used for Oulu because no male flowering observations exist. Leaf bud burst and flowering of birch occur almost simultaneously. Linkosalo Citation(2000) reported that the difference between the observed first date for male flowering and leaf bud burst is 1.1 days, with male flowering observed first.
The result is based on over 750 observations of leaf bud burst and male flowering during 55 years in South and Central Finland.
At both sites the observed trees are adults growing in exposed habitats representing typical climatic conditions. Observations are made repeatedly by the same person at each of the trees (Kubin et al., Citation2004).
Analyses
Kevo data
– The total annual pollen sums (all terrestrial pollen both identified and unidentified but excluding spores) and the annual sums of Betula, Pinus, Juniperus, Ericaceae, Cyperaceae, Poaceae and Urticaceae from the volumetric airborne pollen samples were compared with the corresponding data from the deposition samples by using the Pearson correlation analysis. All data‐sets, except those of Poaceae, which were normally distributed, were normalised using log‐transformation.
Oulu data
– The annual sums of Betula spp., Juniperus, Picea, Poaceae, Salix and Urticaceae from the Burkard samples were compared with the corresponding data from the Tauber samples by using the Pearson correlation analysis. The total annual pollen sums and the annual sums of Alnus, Pinus, and Rumex were compared with the non‐parametric Spearman rank correlation because the data could not be normalized with the same transformation (Tables and ).
Table I. Percentage occurrence of the most common pollen taxa in the Burkard and Tauber traps at A. Kevo and B. Oulu.
Table II. Comparison of the annual sums of airborne pollen (Burkard) and annual pollen accumulation (Tauber) at A. Kevo and B. Oulu.
Results
At Kevo the pollen flora of the deposition samples consisted of 68 different genera, species or subspecies of vascular plants, while in the air samples the number of taxa, identified to the level of genera or family, was 27; a wider range was present but not separately identified. The corresponding figures for Oulu were 65 and 29. The big difference in the number of taxa is a result of the level of identification. The rationale behind obtaining airborne samples was to provide a forecast for allergy sufferers, and therefore greater attention was paid to identifying pollen types with allergenic properties. Deposition samples, in contrast, were used to interpret fossil pollen assemblages where the best possible ecological resolution is important. For both, however the record of the tree and shrub taxa is relevant, i.e. all tree and shrub taxa are identified for both sampler types and the more detailed range of identification in the deposition samples is with respect to low‐growing dwarf shrub and herbaceous species. The sum on which the percentages are based includes all terrestrial pollen both identified and non‐identified.
At Kevo, tree pollen (Betula spp. and Pinus sylvestris) made up 92.8% of all the pollen in the air samples, and in Oulu the figure was nearly as high, 90.6%. In the deposition samples the fraction of tree pollen was 61.5% in Kevo and 89.0% at Hailuoto (Oulu). At Kevo virtually all the tree pollen came from Betula and Pinus, but at Hailuoto Alnus and Picea were additionally present in low percentages (Table ).
In the deposition samples both the taxa and the pollen proportion from low‐growing plants differed between the two monitoring sites. At Kevo, where the deposition sampler was placed on a mire, the pollen of Ericaceae and Cyperaceae made up to 34.5% of the total pollen (i.e. the vast majority of the non‐tree pollen), while the proportion of these pollen types in the Hailuoto samples (near Oulu) was only 1.4%. At Hailuoto, the total proportion of non‐tree pollen was lower: 11.0% (Table ) but within this genera, Melampyrum, Myrica, and Filipendula were relatively abundant (Table ).
At Kevo, Poaceae and Urticaceae pollen were present in the air samples in all years except one (Urticaceae). In the deposition samples Poaceae pollen was present in all years except two. Urticaceae pollen, however, was not found in six years of the deposition samples.
In Oulu, Poaceae and Urticaceae pollen were present in the air samples in all years, and made up a considerable proportion of all airborne pollen, 3.1% and 2.8%, respectively (Table ). Artemisia, Rumex and Salix pollen were present every year and Chenopodium was found in all but one year of the air samples. Poaceae pollen was found in the deposition samples in all years and made up 2.6% of total pollen (Table ). In the 20 year record, Urticaceae pollen was missing in six years, Artemisia was represented in only 7 years and Chenopodium in only 5 years. Together they made up only 0.5% of total deposited pollen.
At Kevo, the annual fluctuations in the amounts of total pollen, tree pollen (Betula and Pinus) and Urticaceae pollen were correlated between the two sampling methods (Table ; Figures & ), but such a correlation was not detected for Ericaceae, Cyperaceae (Table ; Figure ) or Poaceae pollen (Table ). In Oulu, the annual fluctuations in the amounts of total pollen, tree pollen (Alnus, Betula, Pinus, Picea) and Rumex pollen were correlated (Table ; Figures & ), but unlike the Kevo results, a significant correlation was not detected for Poaceae pollen nor for Urticaceae. The trend line in Figure (Oulu) is affected by the abnormally high Betula pollen levels in 1989 and by large values of Melampyrum in the Hailuoto Tauber trap in the year 2000, and it is this latter result that determines the angle of the trend line. The Melampyrum is certainly local in origin and the value abnormal (insect transported). Its inclusion in the analysis misleadingly suggests that there is pollen recorded by the Burkard sampler when no pollen is collected by the Tauber trap. Without this point, the trend line would pass close to the origin. What is clear from Figure is that in the Oulu area, over the period considered, the total amount of pollen detected was nearly twice that recorded at Kevo.
Figure 2 The annual pollen sum recorded by the Tauber sampler(deposited pollen) plotted against the corresponding sum from the Burkard sampler (airborne pollen) at: A. Kevo (n = 23) and B. Oulu (n = 22) for the years 1982–2004 (Kevo) and 1981–2004 (Oulu).
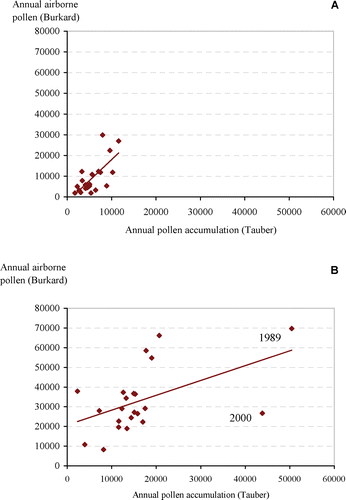
Figure 3 Variation in annual pollen sums ofPinus (A), Betula (B), Cyperaceae (C), Ericaceae (D) and Urticaceae (E), as recorded by Burkard and Tauber traps in Kevo.
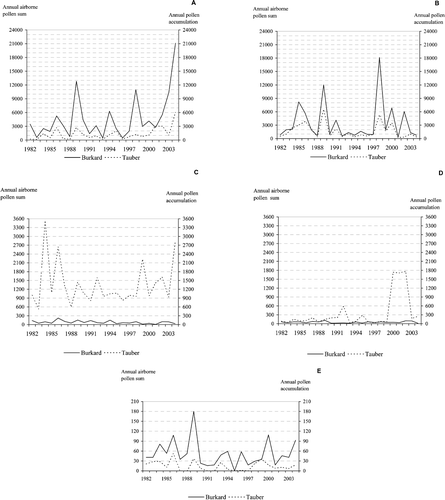
Figure 4 Variation in annual pollen sums ofPinus (A) and Betula (B), as recorded by Burkard and Tauber traps in Oulu and Hailuoto, respectively.
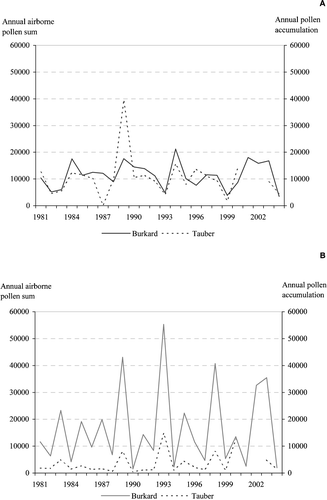
In most years, airborne Pinus sylvestris and Betula spp. pollen was recorded before the onset of the local flowering at both sampling localities. The amount of pre‐flowering pollen varied greatly between the years, with high counts being measured in Kevo and Oulu independently of each other (Figure ). However, the proportion of pre‐flowering airborne pollen out of the total annual pollen, for the whole monitoring period, at Kevo and Oulu, respectively, was 24.3% and 14.8% for Betula, and 9.6% and 6.5% for Pinus, i.e. at both sampling localities the quantity of pre‐flowering Betula pollen was at least double that of pre‐flowering Pinus pollen. At both Kevo and Oulu south and south–west winds are common during Spring (March–May), 43.8% and 39.4% of the measured winds respectively for the two localities (Alalammi, Citation1987), which is relevant for the transport of arboreal pollen from areas where flowering starts earlier.
Figure 5 The measured amounts(sum of daily average pollen counts in m3 of air) of airborne Pinus (A) and Betula (B) pollen before the observed start of the Pinus and Betula male flowering in Kevo, and Pinus male flowering and Betula bud burst at Muhos 1997–2006.
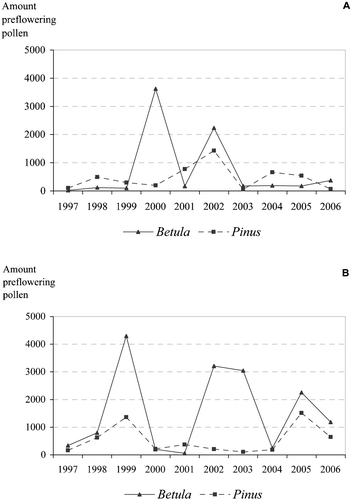
Exceptionally strong pulses of airborne long‐range transported Betula pollen were detected in Kevo in the years 2000 and 2002, nearly a month before the onset of local flowering. In 2000, long‐range transported pollen comprised as much as 44.7% of the annual airborne pollen catch. In 2002 the figure was 33.4%. It is likely that on both occasions the long‐range transported pollen was deposited on the snow cover (Figure ). The year 1999 was characterised by large amounts of long‐range transported tree pollen in the Oulu area, even though the annual pollen sums of Betula (5 390 pollen grains m−3) and Pinus (3 883 pollen grains m−3) were relatively low. For example, 79.7% of the annual total birch pollen was detected about one month before local flowering started (Figures & ). In addition, long‐range transported pine pollen values (1 368 pollen grains m−3) were 35.2% of all Pinus pollen in that year, whereas in other years, between 1997 and 2006, the proportion of pre‐flowering pollen fluctuated between 0.8% (105 pollen grains m−3) in 2003 and 6.2% (1 517 pollen grains m−3) in 2005. In Kevo, the highest proportions of pre‐flowering pine pollen fluctuated between <1% (2003) to a high of 30.2% of total pollen values (2002). The amount of pre‐flowering pollen varies both between individual years and between different sampling sites (Figure ). Mostly, the two taxa behave in different ways in different years.
Figure 6 The development of daily (Julian days) average airborne birch pollen sums in Kevo during the spring of 2000 (A) and 2002 (B). The dashed line shows the depth of snow in millimetres (second y‐axis). The start of local birch pollination is indicated with an arrow.
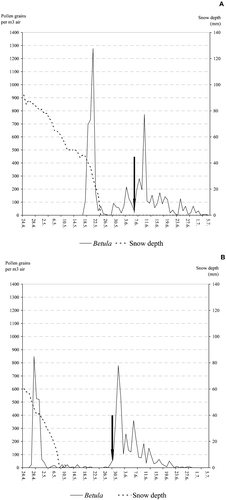
Despite the long‐range episodes and the high proportion of pre‐flowering pollen, the annual fluctuations in the total amounts of Betula recorded by both samplers and at both monitoring sites were strongly correlated (Table ; Figures & ). Only in 2002 was the annual count of airborne birch pollen relatively abundant, but the amount of deposited pollen low (Figure ).
Discussion
The correlation in the fluctuations of total annual pollen in both sampling systems can be most clearly seen from the Kevo results (Table ). However, the significance of sampling height was also evident; the correlation was mainly due to the synchronous fluctuations in the annual sums of tree pollen (Betula spp. and Pinus sylvestris), the most abundant component in both sample types. No similar correlation occurred in the low‐growing plants, with the exception of Urticaceae (Kevo) and Rumex (Oulu). This, together with the small amount of Ericaceae and Cyperaceae pollen found in the air samples at Kevo, strongly suggests that Ericaceae and Cyperaceae pollen does not rise very high above the ground, and that the deposited pollen originates primarily from local sources. The result is not likely to be due to the different abilities of the two samplers to catch pollen; Levetin et al. Citation(2000) demonstrated that if the Burkard and Tauber samplers are placed side by side at the same height on a roof top they are equally effective at recording the annual contribution of anemophilous pollen of the region. Hicks and Hyvärinen Citation(1986) also observed that airborne pollen concentration of low‐growing plants decrease sharply with height; a Tauber trap placed at ground level trapped 3.5 times more overall pollen than one situated nearby on a one metre pole, with the main difference being the lack of pollen from low‐growing plants. In the dwarf‐shrubs belonging to the Ericaceae insect pollination plays an important role (Fröborg, Citation1996; Jacquemart, Citation1993; Nuortila et al., Citation2002), so that dispersal to and the entrainment into the air is not an adaptive trait. Tree pollen, on the other hand, is released at height and becomes easily entrained and transported in the atmosphere and thus reflects pollen production at local and regional scales. This is in accordance with Hicks Citation(1992), who shows that the spatial components of the pollen assemblage recorded by a deposition sampler with its opening at ground level can be divided into a regional record, representing mostly arboreal pollen and a local record, which is best illustrated by the non‐arboreal component.
In the Tauber samples, the proportion of pollen from low growing plants differed substantially between the two sampling localities; Cyperaceae and Ericaceae pollen alone made up 34% of the total pollen in Kevo, while in the Oulu area only some 11% of the deposited pollen came from plants other than trees (Table ). The result is easily explained by the vegetation characteristics of the sampling sites and the location of the Tauber traps. At Kevo, the Tauber trap was sunk into a small mire dominated by Cyperaceae and Ericaceae species. In the Oulu area the trap is within a pine forest in the middle of the island, Hailuoto, but there are fields and ditches in close vicinity. Oulu lies in the middle boreal forest zone more than 500 kilometres south of Kevo, which explains the presence of more warmth‐demanding species such as Myrica, while the cultural landscape, typical for central Hailuoto (Väre et al., Citation2005), explains the presence of Artemisia, Chenopodium, Filipendula, Melampyrum and Rumex. In contrast, for the air samples the proportion of tree pollen was equally high at both sampling localities. The most abundant low growing taxa in the air samples, Poaceae and Urticaceae, were also similar at both localities.
The correlation between the two samplers of the fluctuations in the annual quantities of total pollen and Pinus pollen were less clear in the Oulu area than at Kevo. The difference probably reflects both the distance between the two samplers and the slightly differing forest composition around each; at Kevo the Burkard and Tauber samplers are 1 km apart, while in the Oulu area they are 35 km apart. In Oulu the dominant (tree) pollen type in the Burkard samples was Betula, but in the Hailuoto Tauber samples it was Pinus. In the Oulu area, the annual fluctuations of Betula and Pinus pollen quantities were not correlated with each other (analyses not shown) in either the Tauber or the Burkard samples. This lack of synchronisation of high and low pollen years for Pinus and Betula reflects differences in their flowering biology and phenological development. Autio and Hicks Citation(2004) show that in northern Finland, the amount of pollen deposited by Betula and Pinus is affected by thermal factors during different time periods in the preceding year. However, these forests show distinct pollen years in which pollen deposition tends to be high in several tree taxa including Betula spp. and Pinus sylvestris (Hicks, Citation2001). Ranta and Satri Citation(2007) demonstrate that the interannual fluctuations of airborne pollen sums are positively correlated among combinations of broadleaved tree genera belonging to the plant families of Fagales and Salicaceae. In this case, fluctuations are connected to the summer temperatures of the year preceding flowering.
Interestingly, with respect to Betula, the correlation of the annual pollen quantities in both sampling systems was equally strong at both sites (rp>0.8), which suggests that birch pollen becomes evenly mixed in the atmosphere. Moreover, the annual fluctuations in Betula male catkin numbers are strongly correlated over distances of several hundreds of kilometres (Ranta et al., Citation2008). The same applies for most wind‐pollinated forest trees: the male cones in Pinus spp., for example (Koenig & Knopps, Citation2000).
The total amount of pollen in the air at Kevo was only half of the amount present in the air in the Oulu area (Figure ). This reflects the difference in pollen productivity between the northern and middle boreal zones. In the middle boreal zones the trees are both taller and denser and temperature conditions do not limit pollen production to the same extent as in the northern zone. In both situations, the amount of pollen in the deposition samples appeared to be less than in the air samples but the figures are not comparable since Burkard samplers measure pollen per m−3 of air and the Tauber samplers measure deposition on a cm2 of land surface.
At Kevo Urticaceae pollen was frequently found in both types of sampler and the fluctuations in the annual quantities of this type in deposition and air samples were correlated. Urticaceae pollen is often considered as an anthropogenic indicator (Behre, Citation1981; but see Court‐Picon et al., Citation2006). In the Kevo study area, however, U. dioica, the only species constantly found in the area, is a component of the natural luxurious tall herb vegetation alongside rivers and brooks (Mäkinen et al., Citation1982). In contrast to the Kevo situation, U. dioica is more common in the Oulu region where it most frequently occurs in anthropogenic situations (Väre et al., Citation2005). The absence of any correlation between the annual fluctuations of Urtica pollen in the deposition and air samples in the Oulu area is probably due to the difference in abundance of Urtica stands in the immediate vicinity of the two sampling devices (Hicks, Citation1992). Sampling height also affects the amount of Urticaceae and also Poaceae pollen collected (Fiorina et al., Citation1999). Long‐range transport pollen may also contribute to the presence of Urticaceae pollen in air and deposition samples, since the pollen grain is very small and light and easily reaches the atmosphere. Theoretical considerations and case studies suggest that the same applies to other pollen types that become entrained into the atmosphere (Cecchi et al., Citation2006; Ranta & Pessi, Citation2006; Sofiev et al., Citation2006).
Poaceae pollen was constantly found in both samplers, making up 1.1% and 3.1% of total airborne pollen, and 0.7% and 2.6% of total deposited pollen, at Kevo and Oulu, respectively. However, the annual fluctuations in the amounts of grass pollen in the two samplers were not correlated at either site. This suggests that the relevant source area for some grass pollen may be quite small, as has been reported by Bunting et al. Citation(2004). On the other hand, the constant presence of grass pollen at >1% of all airborne pollen indicates that some grass pollen types disperse well into the atmosphere. At both Kevo and Oulu, the Poaceae pollen flora originates from tens of species, some of which benefit from anthropogenic impact and others that are confined to natural environments (Heikkinen & Kalliola, Citation1990).
The annual sums of airborne and deposited Betula pollen were not correlated for the year 2002 and in this year long‐range transported Betula pollen comprised 33.4% of the annual airborne birch pollen sum at Kevo. The majority of this long‐range transported pollen originated in Russia, over 1000 km distant from Kevo, but Belorusian and Ukrainian forests also contributed to the peak. The potential source areas for this pollen were analysed by Siljamo et al. Citation(2007) and Sofiev et al. Citation(2006), with inverse simulations performed with an integrated pollen emission and transport model constructed on the basis of the emergency modelling system SILAM (http://silam.fmi.fi). For comparison, in 2000, the fraction of long‐range transported Betula pollen of all airborne pollen was greater, 44.7%, and yet the annual sums of airborne and deposited Betula pollen correlated. In both years, the pre‐flowering long‐range transported pollen was probably deposited on snow (Figure ), and therefore, could have been washed away with the snow‐melt water. However, the fact that the fraction of long‐range transported pollen in the Kevo area is constantly high (Hicks et al., Citation1994; Oikonen et al., Citation2005), together with the observed strong correlation between the annual sums of airborne and deposited pollen suggests that long‐range transported birch pollen is equally measured by both devices.
Hicks et al. Citation(1994) report a good correlation between the annual amounts of airborne birch pollen measured before local flowering, and the non‐local, Betula pubescens/pendula type pollen in the deposition samples at Kevo during the years 1984 to 1993. However, the fraction of deposited non‐local pollen was substantially higher compared to pre‐flowering airborne Betula pubescens/pendula type pollen. This is interpreted as indicating that long‐range transport continues to occur during and after the time of local pollination. In the present study, the pollen in the air samples which originates from remote areas is distinguished only if the episodes take place before the onset of local pollination, as defined by phenological observations.
Pinus, on the other hand, shows a different behaviour: during the study period (1997–2006), at both Kevo and Oulu, Pinus pollen was detected in the air before the onset of local male flowering in much smaller amounts than pre‐flowering Betula (Figure ). A strong pulse of long‐range transported pine pollen before the onset of local pine flowering was detected only once, in 2001 at Kevo, and even then the amount of 28% of total annual pollen was a modest contribution. This suggests that the proportion of long‐range transported Pinus pollen in northern tree line areas is relatively small.
Conclusions
The pollen of trees, and especially of Betula spp., in deposition samples reflects both the vegetation and the annual variation in pollen production at the regional scale. The pollen of Ericaceae and Cyperaceae reflects the vegetation of the sampling site rather than that of a larger region. However, more comparisons between time series or airborne and deposited pollen in different environments are needed to reveal general patterns of pollen dispersion in the air and deposition in sediments.
The presence of Urticaceae pollen in the deposition samples may not always reflect human influence. This has implications if the taxon is regarded as an indicator of anthropogenic impact on the natural vegetation. The same applies to the Poaceae pollen; especially since the different grass species are indistinguishable from one another on the basis of their pollen morphology (except cereals).
Long‐range transport of pollen affects both the quantity and species composition of the airborne and deposited pollen flora in northern latitudes and may thus complicate the interpretation of pollen records incorporated in sediments (i.e. deposited) in terms of past vegetation. Betula pollen, in particular, is readily transported over long distances, and Betula pollen counts are likely to be affected more than Pinus pollen counts. During our study period we detected only one clear pine pollen long‐range transportation event. This suggests that the pine pollen in this study region originates primarily from local and regional sources. However, Pinus flowers later in the year than Betula does and frequently the hot dry conditions which trigger off pollen emission are experienced over hundreds kilometres, so the possibility of pine pollen being present in the air long before local flowering is less likely than in the case of birch.
At present, our knowledge of which non‐arboreal, anemophilous pollen types are potentially transported over long distances is limited, although Urtica appears to be one example. The simultaneous use of volumetric and deposition samplers, together with phenological observations of local plants and meteorological data, is clearly helpful in evaluating the presence of non‐local pollen, a piece of information which is highly relevant for palaeoecologists.
Acknowledgements
The airborne pollen data for Oulu and Kevo was produced by the Finnish Aerobiology Unit. This study received financial support from the Finnish Academy (HR), the Thule Institute, Oulu (CS) and the Finnish Geology Graduate School (CS), and forms a contribution to both the Pollen Monitoring Programme and the EU 6FP project ‘Millennium: European climate of the last millennium’, contract number 017008. We would like to thank Raija‐Liisa Huttunen, Heidi Hyyppä, Jacqueline van Leeuwen and Tiina Lankila for counting the Tauber trap pollen.
References
- Alalammi , P. 1987 . Atlas of Finland , Helsinki : Natl Board Surv./Geogr. Soc. Finland . Folio 131. Climate
- Autio , J. and Hicks , S. 2004 . Annual variations in pollen deposition and meteorological conditions of the fell Aakenustunturi in northern Finland: Potential for using fossil pollen as a climate proxy. . Grana , 43 : 31 – 47 .
- Behre , K.‐E. 1981 . The interpretation of anthropogenic indicators in pollen diagrams. . Pollen Spores , 23 : 225 – 245 .
- British Aerobiology Federation . 1995 . Harpenden : BAF . Airborne pollens and spores, a guide to trapping and counting, (1st ed.)
- Bunting , M. J. , Gaillard , M. J. , Sugita , S. , Middleton , R. and Broström , A. 2004 . Vegetation structure and pollen source area. . Holocene , 14 : 651 – 660 .
- Cecchi , L. , Morabito , M. , Domeneghetti , M., P. , Crisci , A. , Onorari , M. and Orlandini , S. 2006 . Long distance transport of ragweed pollen as a potential cause of allergy in central Italy. . Ann. Allergy Asthma Immunol. , 96 : 86 – 91 .
- Colpaert , A. 1993 . Land use mapping with Landsat 5 TM Imagery: A case study from Hailuoto, Finland. . Fennia , 171 : 1 – 23 .
- Court‐Picon , M. , Butler , A. and de Beaulieu , J.‐L. 2006 . Modern pollen/vegetation/land‐use relationships in mountain environments: An example from Champsaur valley (French Alps). . Veget. Hist. Archaeobot. , 15 : 151 – 168 .
- Faegri , K. and Iversen , J. 1989 . Textbook of pollen analysis , Chichester/New York/Brisbane/Toronto/Singapore : J. Wiley & Sons . (4th ed. by K. Faegri, P. E. Kaland & K. Krzywinski)
- Finnish Meteorological Institute . 1991 . Meteorological Yearbook of Finland, Vol. 90 Suppl., Part 1–1990 , Helsinki : FMI .
- Fiorina , A. , Mincarini , M. , Sivori , M. , Brichetto , L. , Scordamaglia , A. and Canonica , G. W. 1999 . Aeropollinic sampling at three different heights by personal volumetric collector (Partrap FA 52). . Allergy , 54 : 1309 – 1315 .
- Fröborg , H. 1996 . Pollination and seed production in five boreal species of Vaccinium and Andromeda (Ericaceae). . Can. J. Bot. , 74 : 1363 – 1368 .
- Hämet‐Ahti , L. 1988 . Atlas of Finland , Helsinki : Natl Board Surv./Geogr. Soc. Finland . Folio 141–143. Biogeography, Nature conservation
- Heikkinen , R. K. 1998 . Can richness patterns of rarities be predicted from mesoscale atlas data? A case study of vascular plants in the Kevo Reserve. . Biol. Conserv. , 83 : 133 – 143 .
- Heikkinen , R. and Birks , H. J. B. 1996 . Spatial and environmental components of variation in the distribution patterns of subarctic plant species at Kevo, N Finland – a case study at the meso‐scale level. . Ecography , 19 : 341 – 351 .
- Heikkinen , R. and Kalliola , R. 1990 . The vascular plants of Kevo Nature Reserve (Finland): An ecological‐environmental approach. . Kevo Notes , 9 : 1 – 56 .
- Hicks , S. 1992 . Aerobiology and palaeoecology. . Aerobiologia , 8 : 220 – 230 .
- Hicks , S. 2001 . The use of annual arboreal pollen deposition values for delimiting tree‐lines in the landscape and exploring models of pollen dispersal. . Rev. Palaeobot. Palynol. , 117 : 1 – 29 .
- Hicks , S. and Hyvärinen , V.‐P. 1986 . Sampling modern pollen deposition by means of “Tauber traps”: Some considerations. . Pollen Spores , 28 : 219 – 242 .
- Hicks , S. , Helander , M. and Heino , S. 1994 . Birch pollen production, transport and deposition for the period 1984–1993 at Kevo, northernmost Finland. . Aerobiologia , 10 : 183 – 191 .
- Hicks , S. , Tinsley , H. , Pardoe , H. and Cundill , P. 1999 . Supplement to the guidelines. European Pollen Monitoring Programme , Oulu : Oulu Univ. Press . (http://wdc.obs‐mip.fr/paleo/pmp/guide.html)
- Hicks , S , Ammann , B , Latalowa , M , Pardoe , H and Tinsley , H , eds. 1996 . European Pollen Monitoring Programme: Project description and guidelines , Oulu : Oulu Univ. Press . (http://wdc.obs‐mip.fr/paleo/pmp/pmp.html)
- Hirst , J. M. 1952 . An automatic volumetric spore trap. . Ann. Appl. Biol. , 39 : 29 – 33 .
- Hjelmroos , M. 1991 . Evidence of long‐distance transport of Betula pollen. . Grana , 30 : 215 – 228 .
- Jacquemart , A.‐L. 1993 . Floral visitors of Vaccinium species in the High Ardennes, Belgium. . Flora , 188 : 263 – 273 .
- Koenig , W. D. and Knops , J. M. H. 2000 . Patterns of annual seed production by northern hemisphere trees: A global perspective. . Am. Natur. , 155 : 59 – 69 .
- Kubin , E. , Poikolainen , J. , Hokkanen , T. , Karhu , J. and Pasanen , J. 2004 . Field instructions for plant‐phenological observations , Muhos : Muhos Res. St. Finn. Forest Res. Inst .
- Levetin , E. , Roger , C. A. and Hall , S. 2000 . Comparison of pollen sampling with Burkard spore trap and a Tauber trap in a warm temperate climate. . Grana , 39 : 294 – 302 .
- Lindgren , D. , Paule , L. , Shen , X. H. , Yazdani , R. , Segerström , U. , Wallin , J. E. and Lejdebro , M. L. 1995 . Can viable pollen carry Scots pine genes over long distances? . Grana , 34 : 64 – 69 .
- Linkosalo , T. 2000 . Mutual regularity of spring phenology of some boreal tree species: Predicting with other species and phenological models. . Can. J. Forest Res. , 30 : 667 – 673 .
- Mäkinen , Y. 1981 . Random sampling in the study of microscopic slides. . Rep. Aerobiol. Lab. Univ. Turku , 5 : 27 – 43 .
- Mäkinen , Y. , Kallio , P. and Nurmi , J. 1982 . Vascular flora of Inari Lapland. 5. Urticaceae – Caryophyllaceae. . Rep. Kevo Subarctic Res. St. , 18 : 10 – 94 .
- Nathan , R. , Perry , G. , Cronin , J. T. , Strand , A. E. and Cain , M. L. 2003 . Methods for estimating long‐distance dispersal. . Oikos , 102 : 261 – 273 .
- Nuortila , C. , Tuomi , J. and Laine , K. 2002 . Inter‐parent distance affects reproductive success in two clonal dwarf shrubs, Vaccinium myrtillus and Vaccinium vitis‐idaea (Ericaceae). . Can. J. Bot. , 80 : 875 – 884 .
- Oikonen , M. , Hicks , S. , Heino , S. and Rantio‐Lehtimäki , A. 2005 . The start of the birch pollen season in Finnish Lapland: Separating non‐local from local birch pollen and the implication for allergy sufferers. . Grana , 44 : 181 – 186 .
- Ranta , H and Pessi , A.‐M , eds. 2006 . The Finnish Pollen Bulletin. Supplement , Turku : Digipaino .
- Ranta , H. and Satri , P. 2007 . Synchronized inter‐annual fluctuation of flowering intensity affects the exposure to allergenic tree pollen in North Europe. . Grana , 46 : 274 – 284 .
- Ranta , H. , Hokkanen , T. , Linkosalo , T. , Laukkanen , L. and Bondestam , K. 2008 . Male flowering of birch; Spatial synchronization, year to year variation and relation of catkin numbers and airborne pollen counts. . Forest Ecol. Manag. , 255 : 643 – 650 .
- Ranta , H. , Kubin , E. , Siljamo , P. , Sofiev , M. , Linkosalo , T. , Oksanen , A. and Bondestam , K. 2006 . Long‐distance pollen transport causes problems for determining the timing of birch pollen season in Fennoscandia by using phenological observations. . Grana , 45 : 297 – 304 .
- Siljamo , P. , Sofiev , M. and Ranta , H. 2007 . “ An approach to simulation of long‐range atmospheric transport of natural allergens: An example of birch pollen. ” . In Air pollution modelling and its application , Edited by: Borrego , C and Norman , A.‐L . Vol. XVII , 331 – 339 . New York : Springer Sci./Bus. Media .
- Sofiev , M. , Siljamo , P. , Ranta , H. and Rantio‐Lehtimäki , A. 2006 . Towards numerical forecasting of long‐range air transport of birch pollen: theoretical considerations and a feasibility study. . Int. J. Biometeor. , 50 : 392 – 402 .
- Sugita , S. , Gaillard , M.‐J. and Broström , A. 1999 . Landscape openness and pollen records: A simulation approach. . Holocene , 9 : 409 – 521 .
- Tauber , H. 1974 . A static non‐overload pollen collector. . N. Phytol. , 73 : 359 – 369 .
- Terasmäe , J. 1951 . On the pollen morphology of Betula nana. . Sv. Bot. Tidskr. , 45 : 358 – 361 .
- Väre , H. , Ulvinen , T. , Vilpa , E. and Kalleinen , L. 2005 . Oulun kasvit ‐ Piimäperältä Pilpasuolle , Helsinki : Bot. Mus. Finn. Mus. Nat. Hist. Univ. Helsinki . Norrlinia, 11 (in Finnish)