Abstract
The developmental events in the periplasmic space and the cytoplasm of microspores of Magnolia sieboldii during microspore ontogeny have been traced with transmission electron microscopy in detail and compared to some developmental stages of other members of Magnoliaceae. We show that in mature pollen grains some important features, such as a columellate infratectum and a lamellate endexine, are not evident and highlight the importance of ontogenetic studies for the proper evaluation of exine structure. One of our aims was to find out whether the sequence of sporoderm developmental events represents the sequence of self-assembling micellar mesophases, the initiation of which is genetically controlled. Another aim was to consider substructural models of exine by John Rowley and his other chief ideas, and to analyse how these ideas concur with micellar hypotheses of exine development.
The basal angiosperms have attracted the attention of many investigators (e.g. Sampson, Citation1977, Citation2000; Gottsberger, Citation1988; Dinis & Mesquita, Citation1993, Citation1994; El-Ghazaly et al., Citation2000; El-Ghazaly & Huysmans, Citation2001; Doyle, Citation2005, Citation2009; Taylor & Osborn, Citation2006; Xu & Kirchoff, Citation2008). In our early ontogenetic studies, we also focused on this group, investigating some members of the family Magnoliaceae (Gabarayeva, Citation1986a , Citation1986b , Citation1987a , Citation1987b , Citation1987c , Citation1990, Citation1991a , Citation1991b , Citation1996), Annonaceae (Gabarayeva, Citation1992, Citation1993a , Citation1995), Nymphaeaceae (Gabarayeva & Rowley, Citation1994; Gabarayeva & El-Ghazaly, Citation1997; Gabarayeva et al., Citation2001), Illiciaceae and Shizandraceae (Gabarayeva & Grigorjeva, Citation2003) and Cabombaceae (Gabarayeva et al., Citation2003a ). These studies have shown a great variability of some characters (e.g. exine patterns) on the one hand, and, on the other hand, uniformity of others (e.g. the presence of endexine in all species from all families investigated and a very similar substructure of the exine framework, the glycocalyx).
The scientific heritage John Rowley left us is encyclopaedic. One of his main visions was a universal model of exine structure, which has been refined by him over many years and tested by different methods. According to this model, there is a universal basic unit of any exine: a tuft. The strength of this model has been demonstrated in many of our studies. Later, in search of a common mechanism of exine pattern formation, self-assembly was suggested as a possible driver (Gabarayeva, Citation1993b ; see also Hemsley et al., Citation1992; Collinson et al., Citation1993). This led to the hypothesis about the participation of a self-assembling micellar system in exine formation (Gabarayeva & Hemsley, Citation2006; Hemsley & Gabarayeva, Citation2007). A hypothesis can be regarded as reasonable if it explains the majority of conserved facts. The aim of the present study was to analyse the factual data we received in the light of this hypothesis, and to establish how this hypothesis is compatible with Rowley's model and his other ideas.
Material and methods
Flower buds of Magnolia sieboldii K.Koch were collected from a single plant in the garden of the Komarov Botanical Institute, St Petersburg, Russian Federation, over two years in order to trace all the main developmental stages. Stamens were fixed in 3% glutaraldehyde and 2.5% sucrose in 0.1 M cacodylate buffer, with addition of lanthanum nitrate (1%) for better preservation of the plasma membrane glycocalyx, at pH 7.4 and 20 °C for 24 h. After post-fixation in 2% osmium tetroxide (20 °C, 4 h) and acetone dehydration, the samples were embedded in a mixture of Epon and Araldite. Unless not noted otherwise in the figure captions, ultrathin sections were contrasted with 2% aqueous solution of uranyl acetate and in 0.2% lead citrate. The test for the intine material (acetolysis) was applied to the late microspores and mature pollen grains. Sections were examined with a Hitachi H-600 transmission electron microscope (TEM).
Results
Early tetrad stage
The early tetrad stage (A–E) is preceded by meiosis (F). The presence of sinaptonemic ‘bouquets’ (synaptonemic complexes that are attached to the nuclear envelope by their terminal ends) suggests that this is the stage of late zygotene. Pairs of chromosomes are distinctly visible: The central and lateral elements of the synaptonemic complexes are clearly visible (F). The process of meiotic clarification of the cytoplasm is evident: Many concentric multimembrane formations, functioning as autolytic vacuoles, promote the reduction of ribosome and organelle numbers.
Figure 1. Early tetrad stage and meiosis in Magnoliaceae. A–E. Early tetrad stage in Magnolia sieboldii: A. Plasma membrane lacks any signs of the glycocalyx and contacts directly with callose envelope; the cytoplasm is packed with organelles and contains a large nucleus; B, C. Initial glycocalyx (ig) appears near the plasma membrane; D. Coated vesicles (cv) and coated pit of the plasma membrane (cp) are typical for this stage; E. Endocytotic channels (ec) are also typical feature of the early microspores. F. Meiosis in Magnolia delavayi: Synaptonemic complexes (sc, zygotene stage). Scale bars – 1 μm (A, F), 500 nm (B–E).
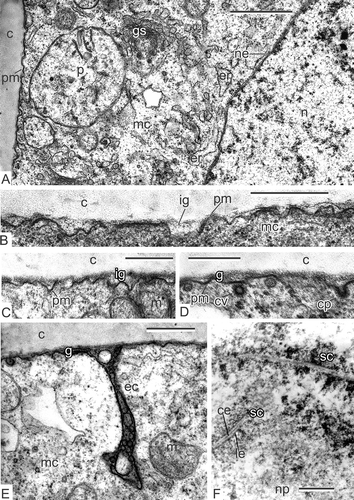
After meiosis completion, the plasma membrane looks ‘naked’, lacking evident signs of the glycocalyx (A). A large nucleus and numerous organelles are visible in the microspore cytoplasm. Very soon, the initial glycocalyx appears as a sparse layer between the plasma membrane and the callose layer (B, C). In some places, coated vesicles and coated pits are visible (D), in others, deep endocytotic channels are evident (E).
Young tetrad stage
A survey of a tetrad microspore shows the typical microspore form, characteristic of tetrahedral tetrads with a large nucleus and a nucleolus in the centre of the cell (A). One of the most specific characters of the microspore cytoplasm is the so-called chain-mail endoplasmic reticulum (er), which occupies a considerable part of the microspore volume (B). This type of endoplasmic reticulum is also evident in the middle tetrad stage (, ), at the late tetrad stage (A, B), as well as in other members of Magnoliaceae in the course of microspore development (F). Many plasma membrane vesicles and their outgrowths or sections of outgrowths – plasmalemmasomes – are visible at the microspore surface (C–E) simultaneously with the appearance of the initial glycocalyx. Then, spherical units appear at the evaginations of the plasma membrane and form clusters or are disposed in a row alongside the plasma membrane (F–H).
Figure 2. Young tetrad stage in Magnolia sieboldii. A. Fragment of a tetrad, showing a survey of a microspore with nucleus, nucleolus and many organelles in the cytoplasm. B. Fragment of a microspore; so-called chain-mail reticulum (cr) is a typical feature of the microspore cytoplasm during the sporoderm development. C, D. Plasmalemmasomes (sections through outgrowths of the plasma membrane, arrows) appear at first alongside the microspore surface. E. The glycocalyx layer (g), looking fibrillar, is added to plasmalemmasomes (arrows). F. The appearance of spherical units (arrowheads) in the glycocalyx layer at the peaks of the plasma membrane. G. Clusters of spherical units (arrowheads), interpreted as spherical micelles in the periplasmic space. H. Row of spherical units (spherical micelles, arrowheads) alongside the plasma membrane of the young microspore. Abbreviations: see . Scale bars – 10 μm (A), 500 nm (B–H).
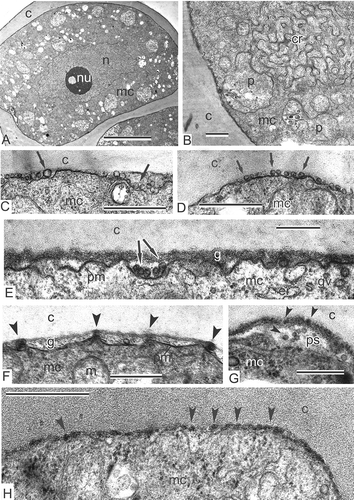
Figure 3. Transition to middle tetrad stage in Magnolia sieboldii. A. Fragment of the microspore cytoplasm and the developing sporoderm; the layer of the glycocalyx shows columns of spherical units-micelles (arrowheads) and also their re-arrangement to rod-like units (cylindrical micelles, arrows); chain-mail reticulum (cr) persists in the cytoplasm; first cup-like plastids (cp) appear here and there. B, C. Rod-like units (arrowheads) accumulate in the glycocalyx, whereas columns of spherical units are still observed in some places (C, arrows). Abbreviations: see . Scale bars – 500 nm.
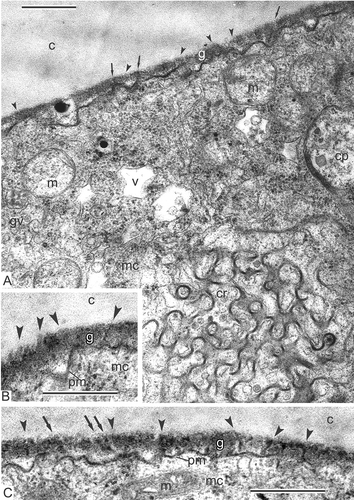
Figure 4. The transition stage from young to middle tetrad. A. Magnolia delavayi: Glycocalyx consists of spherical units (spherical micelles), some of them re-arranging into rod-like units (cylindrical micelles, arrowheads). B. Magnolia sieboldii: Microspore cytoplasmic organelles and inclusions; chain-mail reticulum (cr) and associated stack of membranes (asterisk) in the microspore cytoplasm; the cytoplasm is crowded with ribosomes, polysomes (po), golgi vesicles (gv) and cisternae of endoplasmic reticulum (er); rod-like units are visible in the glycocalyx layer (arrowheads); some mitochondria (m) and plastids (p) are underdifferentiated. C. Michelia fuscata: Glycocalyx consists of spherical units (spherical micelles), some of them re-arranging into rod-like units (cylindrical micelles, arrowheads). Abbreviations: see . Scale bars – 500 nm.
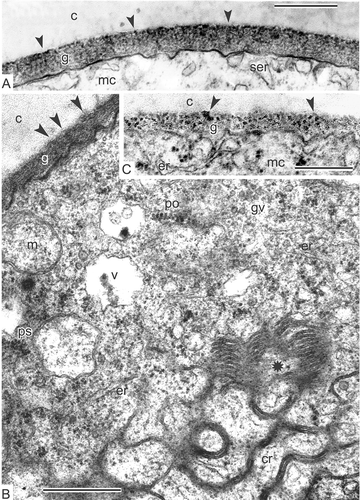
Figure 9. Transition from late tetrad stage to early free microspore stage in Magnolia sieboldii. A. The cytoplasm of the late tetrad microspore, containing different organelles: chain-mail reticulum (cr), its outer membranes form pockets, one of which envelops a cuplike plastid (cp) with inclusions, dilated cisternae of smooth endoplasmic reticulum (er), many mitochondria, plenty of ribosomes and polysomes. B. Fragment of early microspore, just free from a tetrad; a pocket of chain-mail reticulum (cr) includes two plastids and a lipid globule. C. Survey of a free microspore; note distal fold (df) in the aperture region. D. Initiation of the foot layer (arrows). E, F. The endexine lamella is formed (black arrowheads) with the white line visible in its centre, which corresponds to so-called laminate micelle with its typical gap (seen as white line) between the bilayers; the tectum perforations are marked (asterisks). G. Oblique section in the region of the infratectum: Cross-sectioned columellae appear as circles, giving a false impression of granules (black arrowheads); perforations in the tectum also appear as circles (white arrowheads). Abbreviations: see . Scale bars – 1 μm (A, B, C), 500 nm (D–G).
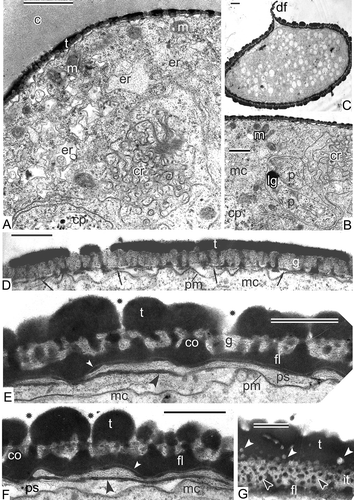
Figure 10. Young free microspore stages, formation of the endexine lamellae (A–E) and different aggregates of endoplasmic reticulum (F, G). A. Magnolia delavayi, a thick endexine lamella (arrow) is formed in the periplasmic space; its white line and another white line, separating the foot layer from the first endexine lamella, are marked (arrowheads); B, C. Michelia fuscata, early stage of the endexine lamella formation (arrows); white line still is not visible but evident already in the foot layer (arrowheads); D, E. Manglietia tenuipes, very thin endexine lamellae are being formed in the periplasmic space; the first lamellae are shown by arrowheads, the second ones by arrows; they correspond to so-called laminate micelle with its typical gap (seen as white line) between the bilayers. F. Chain-mail aggregate of endoplasmic reticulum (cr) in Michelia fuscata in contact with the developing sporoderm by means of cisternae of rough endoplasmic reticulum, stretched out to the plasma membrane (young tetrad stage, glycocalyx consists of spherical units). G. ‘Zebra’-aggregate (zr, with alternating osmiophilic and transparent cisternae) in Liriodendron chinense, undulating in the microspore cytoplasm (mid-tetrad period). Abbreviations: see . Scale bars – 500 nm.
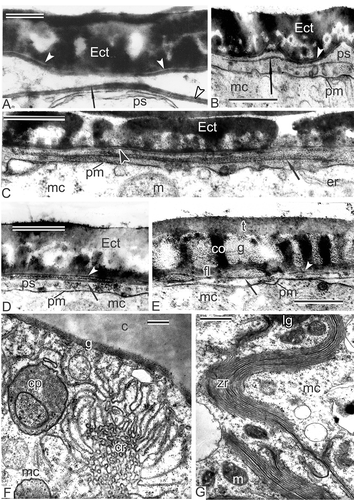
Transition to middle tetrad stage
Gradually spherical units form columns that are more or less perpendicular to the plasma membrane (A–C). Some of them re-arrange to form rod-like units (A–C). Cup-like plastids are typical for developing microspores as well as chain-mail endoplasmic reticulum; the latter seen as a combination of circles and sinusoidal elements in sections (A). In some places, a side branch of the chain-mail endoplasmic reticulum forms stacks of parallel-disposed cisternae, resembling a flaky pie in section (B). The cytoplasm is rich in ribosomes and polysomes, golgi vesicles, cisternae of smooth endoplasmic reticulum, vacuoles, under-differentiated mitochondria and large plastids. In other species, the same spherical units gradually re-arrange to form columns at the corresponding stages of microspore development (A, C: Magnolia delavayi Franch., Michelia fuscata Blume).
Middle tetrad stage
Rod-like units – future columellae – are obvious in the glycocalyx layer. They become more evident due to initial sporopollenin accumulation (A, C). Higher magnification allows for them to be distinguished in more detail and reveals that they are rooted into the cytoplasm (A). Some images allow initial sporopollenin acceptor particles (SAPs; C) to be distinguished. Coated vesicles are seen near the plasma membrane (A). In the distal region of the future aperture, the glycocalyx layer is very insignificant (B).
Figure 5. Middle tetrad stage and transition to late tetrad stage in Magnolia sieboldii. A. Rod-like units in the glycocalyx (cylindrical micelles, arrowheads) start to accumulate sporopollenin and become osmiophilic; they are initial columellae; many rod-like units are rooted in the cytoplasm (arrows). B. No glycocalyx layer in the apertural region (ap). C–E. Gradual accumulation of sporopollenin on the framework of primexine, rod-like glycocalyx units (cylindrical micelles), leads to appearance of procolumellae (arrowheads); in some places, sporopollenin acceptor particles (SAPs) are distinguished (C). F. Large vesicles (asterisks) appear between the plasma membrane of microspore and callose layer in the aperture site (ap). Abbreviations: see . Scale bars – 500 nm.
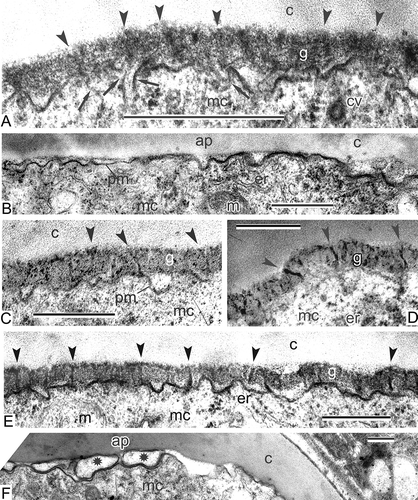
An interesting deviation of the normal process of development is seen at the middle tetrad stage in some microspores in some anthers: Many osmiophilic droplets cover all the microspore plasma membrane (A, B). Higher magnification shows that the glycocalyx layer in such atypical microspores is virtually absent (B), whereas in other stamens of the same material, microspores have a well pronounced glycocalyx with procolumellae (C). The reason for this can be atypical hyperactive tapetal cells with needle-like crystals within the vacuoles (C). In these tapetal cells, the cytoplasm is overcrowded with ribosomes (compare the tapetal cell in C to a normal one in D). The entire tapetal cell surface is incrusted with osmiophilic droplets (C).
Figure 6. Atypically developing microspore sporoderm and tapetal cells in Magnolia sieboldii at the middle tetrad stage. A. Plentiful osmiophilic droplets (arrows) on the surface of the plasma membrane, under the callose envelope; the cytoplasm of the microspore and its organelles looks normal. B. The periplasmic space of the microspore shown in (A) at higher magnification; the glycocalyx layer is absent. C. Atypically hyperactive tapetal cell with needle-like crystals, associated with vacuoles; some cells are packed with ribosomes to such extent that the restricting membranes of organelles are not visible; osmiophilic globules appear on the plasma membrane (C, arrowheads). D. In normal tapetal cells, mitochondria and vacuoles do not contain needle-like crystals, and there are no osmiophilic droplets on the plasma membrane; the membranes of organelles have a normal contrast; ribosomes are united to polysomes. Abbreviations: see . Scale bars – 500 nm.

Transition to late tetrad stage
Sporopollenin accumulation is in progress and procolumellae become more obvious (D, E). At some places, a thin initial tectum appears (E). At the aperture site, large membraneous vesicles appear in the periplasmic space (F). Unusual plastids can be observed (A, B), some of which are cup-like. Furthermore, and even more important, many of them are wrapped in a sheet of endoplasmic reticulum cisternae, often in a sheet of chain-mail reticulum, starting with the middle tetrad stage (A). Association of different forms of endoplasmic reticulum with plastids continues to happen at later stages (A, B). This feature seems to be common for many species of Magnoliaceae: It is also characteristic for Michelia fuscata (C, E, F) and Manglietia tenuipes Dandy (D). Stacks of endoplasmic reticulum cisternae, undulating through the microspore cytoplasm, are also visible (B). Very similar structures are typical for developing microspores of Liriodendron chinense (Hemsl.) Sarg. (G). In addition, the matrix of plastids is full of osmiophilic globules or droplets (A–C, E, F); the latter are preserved until the end of microsporogenesis (, ).
Figure 7. Specific plastids, typical for Magnoliaceae microspores. A, B. Magnolia sieboldii, mid-tetrad microspores: A. Plastids, associated with chain-mail reticulum (cr); plastids contain plentiful osmiophilic globules and they are wrapped in a sheet of endoplasmic reticulum (arrowheads); B. Plastids (two of them are wrapped in an endoplasmic reticulum sheet, arrowheads) and a stack of endoplasmic reticulum cisternae, undulating through the cytoplasm (arrows); compare with the corresponding structure in Liriodendron chinense, Figure 10G. C. Michelia fuscata: Plastids in contact with endoplasmic reticulum cisternae (arrows) contain many osmiophilic globules in their matrix in young free microspore; elements of chain-mail reticulum (ecr) are adjacent. D. Manglietia tenuipes: Cup-like plastids (cp) with osmiophilic globules (shown in side view) in contact with endoplasmic reticulum (arrows) and associated with elements of chain-mail reticulum in late tetrad microspore. E, F. Michelia fuscata: Early free microspores at stage of vacuolisation; plastids with characteristic osmiophilic globules in contact with rough endoplasmic reticulum (arrowheads). Abbreviations: see . Scale bars – 1 μm.
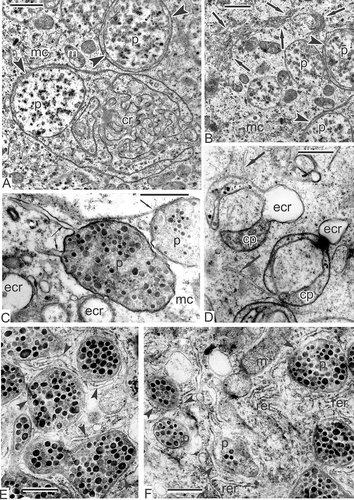
Figure 12. Formation of the first intine layer in Magnolia sieboldii. A. The appearance of middle contrasted substance (asterisk) in the periplasmic space (ps) and simultaneous formation of the second endexine lamella (both lamellae are marked by arrows). B. Microspore in close vicinity to the tapetum; first discrete spherical units of the intine in the periplasmic space (asterisk); tapetal orbicules in the anther loculus (arrowheads). C. The layer of intine-1 (i1) consists of many granule-like units; endexine lamellae are recognisable by their white lines (arrowheads). D. Spherical units in the intine-1 layer are slightly pressed to each other; endexine lamella is shown by arrowhead. E. The distal fold in the process of unfolding; note that the granular intine-1 layer is not yet laid down in the aperture site, because sporoderm regions, included into the distal fold, were inaccessible for accumulation of intine-1 material (see ). F. The distal fold is already spread out; the intine-1 is still late in development in comparison with the rest sporoderm regions. G. Somewhat later stage than in (F); the difference in intine-1 thickness is still evident. H. Somewhat later stage than in (G); the thickness of the intine-1 layer is now the same in aperture and in extra-aperture regions. Abbreviations: see . Scale bars – 1μm (E–H), 500 nm (A–D).
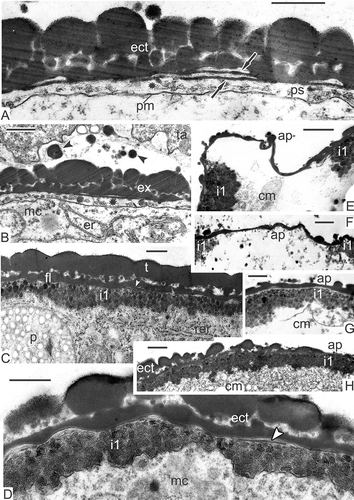
Figure 14. Formation of intine-2 and intine-3. A–E. Magnolia sieboldii: A. Condensation of a material (asterisk) for intine-2 formation in the periplasmic space; note that at this stage thin endexine lamellae are not seen because of the compression of the earlier formed layers of sporoderm; B. Mature acetolised pollen grain (LM) with the distal colpus (arrow); C. The appearance of the second (i2) and the third (i3) layers of the intine (inapertural region); i2 is a channelled layer (arrowheads); i3 is fine-fibrillar and weakly contrasted; D. The same three layers of the intine in aperture site (ap); E. Fragment of a mature pollen grain with generative cell and completely formed sporoderm (ms). F. Magnolia delavayi: mature psilate pollen grain with infolded distal colpus (SEM). Abbreviations: see . Scale bars – 15 μm (B), 10 μm (F), 1 μm (E), 500 nm (A, C, D).
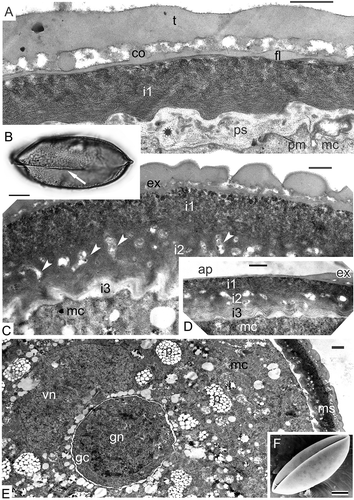
Late tetrad stage
Columellae are well pronounced (A, B), as well as the tectum (A). Higher magnification reveals high-ordered structure of the glycocalyx as ‘columns of coins’ with a dark site inside every ‘coin’ (B, C). Columellae, each based on one column, still preserve the outlines of each ‘coin’ (B, C). Many dividing mitochondria, plastids with droplet-like inclusions, active dictyosomes, rough endoplasmic reticulum cisternae, ribosomes and polysomes are seen in the microspore cytoplasm.
Figure 8. Late tetrad stage in Magnolia sieboldii. A. Not only columellae (arrowheads), but also the tectum (arrows) is well pronounced. B, C. Regular structure of the glycocalyx is evident: Cylindrical micelles, perpendicular to the plasma membrane, show the structure of ‘coin columns’, each ‘coin’ has a dark contrasted core and light halo (arrows); note that procolumellae are based on a single cylindrical micelle (‘coin column’, arrowheads). The tectum (t) is well developed; vesicles in the periplasmic space (B, asterisk) are sections of plasmalemmasomes. Abbreviations: see . Scale bars – 500 nm.
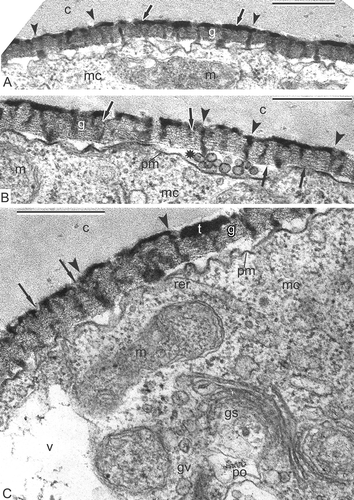
Transition to free microspore period and young free microspore stage
Some tetrads preserve the callose envelope (A), but in others, callose disappears (B). After the final release from the tetrads, the free microspores have a specific shape. They have a distal fold (an aperture site), very similar to the proximal fold of ferns (C).
In early free microspores, the foot layer starts to form, first as a discontinuous layer (D); later, the foot layer becomes continuous and thicker. The tectum and columellae are progressively becoming thicker. At this stage, endexine is developing in the periplasmic space. Being present at first in fragments, the endexine lamellae with the typical central white line becomes soon rather thick and continuous alongside the whole microspore surface (E, F). Magnolia sieboldii microspores develop two endexine lamellae: One at this stage that lies free in the periplasmic space, and another that is pressed tightly to the foot layer, separated from the latter by a white line (E, F). The tectum and the foot layer are much thicker than during the previous stages due to intensive accumulation of sporopollenin of tapetal origin. In oblique section in the region of the infratectum, cross-sectioned columellae appear as circles, giving a false impression of granules (G); perforations in the tectum also appear as circles.
The same sequence of events was observed in other species of Magnoliaceae. Similarly thick endexine lamellae with the central white lines appear at the same point of ontogeny in Magnolia delavayi (A). A much thinner endexine lamella forms in the periplasmic space of Michelia fuscata (B), preceded by a long, narrow cloud-like area (C). A thin endexine lamella also develops in young free microspores of Manglietia tenuipes (D, E). The same is observed at the beginning of the free microspore period in Liriodendron chinense. Note that the first white line, disposed in the lower part of the foot layer, always marks the border between ectexine and endexine (–E), so the first, primordial endexine lamella is tightly pressed to the foot layer.
Different complex aggregates of endoplasmic reticulum were found also in the tetrad and post-tetrad periods of microspore development in their cytoplasm: chain-mail endoplasmic reticulum in Michelia fuscata (F) and in Manglietia tenuipes (not shown), and ‘zebra’ aggregates, associated with lipid globules in Liriodendron chinense (G). As mentioned earlier, an oblique section through the Magnolia sieboldii microspore exine gives more the impression of a granular than a real columellate infratectum (A). A non-radial section is easy to distinguish from the radial one by the plasma membrane and endexine lamellae, which in this case look blurry (A). The distal fold appears in the aperture region, where the exine layer is thinner (B; see also C). A developing endexine lamella is also visible in the region of the fold (B). Simultaneously, tapetal cells of Magnolia sieboldii form pro-orbicules inside surface invaginations (C). During maturation of pro-orbicules, a core and orbicular wall were clearly visible (E), simultaneously with elongated, orbicule-like structures with a central white line (F). The distal folds are formed at the early free microspore stage in all other Magnoliaceae members studied, which seems to be characteristic of this family. The mechanism of their formation remains obscure. Later in development, these folds disappear, spreading to both sides as a result of the increasing volume of the growing microspore.
Figure 11. Young free microspore stages. A–C. Magnolia sieboldii: A. Oblique section through the microspore exine; if a section is not radial, columellae look like granules, plasma membrane and endexine lamella (arrowhead) look blurry; B. Distal fold (df), a peculiar ‘paraphrase’ of proximal fold of ferns, formed in the aperture region after the microspore release from the tetrad; fragments of the developing endexine lamella are visible also inside the fold (arrowheads); C. Tapetal cells (ta) form pro-orbicules (arrowheads) in the invaginations. D. Michelia fuscata, distal fold (df) of the microspore endexine in the vicinity of the tapetum. E, F. Magnolia delavayi: E. The inner border of a tapetal cell with orbicule (o) inside an invagination; the wall of orbicule (ow) and its core part (oc) are observed in a detached orbicule; F. Orbiculelike formations with central white line (arrowhead) appear alongside the radial wall of tapetal cell. Abbreviations: see . Scale bars – 1 μm.
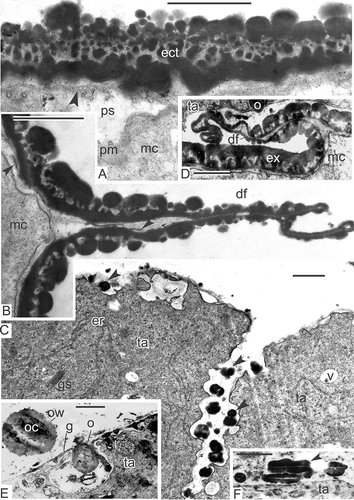
Middle free microspore stage
After the completion of endexine lamellae formation, the development of the first layer of intine is initiated. It starts as accumulations of electron-dense material in the periplasmic space with some single well-shaped spherical granules (B). Orbicules continue to be formed in the tapetal invaginations (B). Somewhat later, these granules accumulate in the periplasmic space and form the first layer of intine (C). Granules in plastids change their contrast and appear electron lucent (C). At higher magnification, the endexine lamellae are still evident between the ectexine and the intine (D). At the apertural site, the development of the granular intine-1 layer is late in comparison with extra-apertural regions (E–G). The reason for this is that at a previous stage, the configuration of the distal fold prevents accumulation of intine material in the aperture site (B). Gradual spreading of the distal fold (E, F) allows accretion of the intine-1 granules (G) and promotes the levelling of this layer alongside the whole wall (H). A special test, involving potassium permanganate contrasting and used to differentiate endexine from ectexine, has shown much weaker contrast of the granular layer than that in the conventionally stained material (compare A, B with C, D). After acetolysis, the granular layer has disappeared completely in different Magnoliaceae members (C–E).
Figure 13. Histochemical test of free microspores at the stage of already formed granular intine-1 with potassium permanganate. A, B. Test revealing endexine in Magnolia sieboldii, stained sections show the scarcely stained granular layer, disposed under endexine lamellae, especially in comparison with conventional staining with uranyl acetate and lead citrate (compare with Figure 12); endexine lamellae marked with arrowheads. C–E. Mature acetolised pollen grains of Magnolia sieboldii (C), Manglietia tenuipes (D) and Magnolia delavayi (E); after acetolysis, all the intine layers (including the first granular layer) have disappeared; endexine lamella is preserved (arrowhead). Abbreviations: see . Scale bars – 500 nm.

The completion of sporoderm development and formation of pollen grains
After the formation of the thick granular layer of intine-1, the next intine layers occur, first as accumulations of substances in the periplasmic space (A); subsequently, the channelled, intine-2 layer is formed and, at last, the intine-3 appears as a fine-fibrillar layer with lower contrast (C). All three intine layers are also seen in the aperture site (D); note that at this and later stages, the endexine lamellae are not visible due to compression.
Mature acetolysed pollen grains of Magnolia sieboldii with distal colpus are boat-shaped and have a psilate surface; this is similar in grains of Magnolia delavayi (B, F). After microspore mitosis, the generative cell appears (E) surrounded by the cytoplasm of the vegetative cell. The cytoplasm of the pollen grains contains many lipid globules, dispersed through the cytoplasm of the vegetative cell and surrounding the generative cell.
Discussion
Sporoderm substructure
Ectexine
Various models of exine substructure have much in common (review in Gabarayeva, Citation2000, p. 3). The early model of Rowley and Flynn (Citation1968) had been worked out for decades in many studies using different methods (Gabarayeva et al., Citation2009a ). The most important milestones of John Rowley's work involve his accounts on the fundamental substructure of the pollen exine (Rowley, Citation1990; Rowley et al., Citation1995), substructure within the endexine (Rowley, Citation1987–1988), sporopollenin receptors (Skvarla & Rowley, Citation1987) and two types of sporopollenin: Receptor-dependent and receptor-independent as introduced by Skvarla and Rowley (Citation1987) and Rowley and Claugher (Citation1991). The main ideas of Rowley and his co-authors are that the exine substructure is universal for pteridophyta, gymnosperms and angiosperms and is characterised by tubule-like units, consisting of core and binder subunits so-called ‘tufts’, and that these units are functionally plasmodesmata-like and are capable of transferring substances/nutrients from the sporangium/anther loculus into the developing spores/microspores (Rowley & Dunbar, Citation1970; Rowley et al., Citation2003). This implies that the spore/pollen wall functions as a living, dynamic system – despite the gradual accumulation of biopolymer sporopollenin. In other words, a complex polymer wall of spores/microspores develops around a framework of tubular glycocalyx (primexine matrix), which persists living until the late stages of spore/microspore development.
Our early studies on basal angiosperms from Magnoliaceae (see earlier) have revealed rod-like or tubule-like units in the glycocalyx (tufts) from the middle tetrad stage onwards. At later stages of the tetrad period, these units gradually accumulate sporopollenin and turn into thin procolumellae, which become massive after intensive sporopollenin deposition during the free microspore period. Subsequent ontogenetic studies on ten selected species of cycads and basal and advanced angiosperms (e.g. Gabarayeva & Grigorjeva, Citation2003, Citation2004), confirmed the uniform character of the glycocalyx consisting of rod-like or cylinder-like units with variations only in their diameter. However, early stages of the tetrad period showed roundish units rather than rods in all species under study.
Later, a new hypothesis linked Rowley's model with his knowledge from the field of colloidal chemistry on the self-assembling behaviour of non-equilibrium micellar systems: All developmental events during microspore sporoderm development are nothing but an unfolding sequence of micellar mesophase (Gabarayeva & Hemsley, Citation2006; Hemsley & Gabarayeva, Citation2007; Blackmore et al., Citation2007, Citation2010). The periplasmic space, which is an arena for sporoderm development, contains glycoproteins (Rowley, Citation1971; Pettitt & Jermy, Citation1974; Rowley & Dahl, Citation1977; Pettitt, Citation1979) and lipopolysaccharides (Rowley, Citation1975). Later in development, sporopollenin (SP) precursors (phenylalanine; Rittscher & Wiermann, Citation1988; Gubatz & Wiermann, Citation1992, Citation1993) and sporopollenin monomers (fatty acids and phenylpropanoids, especially p-coumaric acid; Gubatz et al., Citation1986; Wehling et al., Citation1989) are added to the periplasmic space. All substances are surface-active, which should inevitably form the sequence of self-assembling micellar mesophases following increasing concentration of these substances in a water-based periplasmic medium (spherical micelles, then cylindrical, lamellar and other kinds of micelles).
The present data on Magnolia sieboldii and reconsideration of our earlier data on other Magnoliaceae confirms this hypothesis. At the early tetrad stage, some roundish units are visible in the periplasmic space (–H, , ), as also observed in many other species at the same stage. Additional ontogenetic data also documented roundish units rather than rods at early stages of the tetrad period (Dickinson, Citation1976a , Citation1976b ; Hesse, Citation1985; El-Ghazaly & Jensen, Citation1987; Skvarla & Rowley, Citation1987; Rowley et al., Citation1992). This is in good accordance with the fact that the first mesophase, which appears in a micellar sequence, is characterised by spherical micelles. This phase seems to be rather brief due to dramatic growth of the concentration of surfactants in the periplasmic space and the next mesophase is characterised by cylindrical micelles. This is exactly what has been observed: Rod-like units (tufts) are well visible elements in the periplasmic space. In essence, the whole glycocalyx consists of many radially oriented tufts (cylindrical micelles), ‘standing’ on the plasma membrane on their butt-ends and are tightly packed. The transition from spherical to cylindrical units occurs gradually, so that it is possible to see two types of units simultaneously for some time (, ) and columns of spherical micelles, which are in the process of re-arranging to cylindrical micelles (, , ). An investigation of Brasenia sporoderm ontogeny also showed first spherical units during the early tetrad stage, followed by columns derived from these units (Taylor & Osborn, Citation2006).
From the middle tetrad stage, tuft-micelles start to accumulate sporopollenin or its precursors. From this moment, young procolumellae become more and more evident (). Hence, this period is predominant during the tetrad period. The term ‘rod-like’ does not necessarily refer to straight, ideal column-like elements; there are no geometrically-regular structures in living systems and columellae are not ideal in form in many species, which especially concerns basal angiosperms with their many ancestral features (Sampson, Citation2000; Doyle, Citation2005, Citation2009). In Magnolia, columellae appearing on the base of cylindrical tuft-micelles are always uneven or bent (‘lazy columellae’ in Rowley's words) during development and become very massive in mature pollen grains. In many sections, they look like granules or columellae fragments, though some single real granules occur in the infratectum as a natural consequence of SP accumulation on the first micelle mesophase characterised by spherical micelles. The infratectum in Magnoliaceae has been misinterpreted (e.g. Walker, Citation1976), because the peculiar nature of columellae had been overlooked, which can only be understood when considering ontogenetic data. A recent study of 20 members of the Magnoliaceae confirms the columellate structure of exine (Xu & Kirchoff, Citation2008). Sometimes, however, fragmentary ontogenetic data does not allow correct interpretation of infratectum structure (Galati et al., Citation2011).
Rod-like units are far from being homogeneous structures. Each apparently has a central core and a surrounding sheath, a binder (Rowley et al., Citation1981), ultimately forming a cylindrical structure. The sheath appears to be helically organised, with a diameter range 70–200 nm. So-called normal cylindrical micelles have a hydrophobic liquid-hydrocarbon core and hydrophilic sheath, which causes dark-contrasted cores and moderate-contrasted sheaths in tuft-micelles (B, С). The phenomenon of initial dark-staining of the glycocalyx units' core with lighter periphery to lighter cores with darker binder later in development is called stain reversal, first described by Rowley and co-authors (Dunbar & Rowley, Citation1984; Rowley, Citation1987–1988; Rowley & Claugher, Citation1991; Rowley & Rowley, Citation1998) and later also found in other species (Gabarayeva et al., Citation1998, Citation2010; Gabarayeva & Grigorjeva, Citation2004). If the glycocalyx units are truly micelles, stain reversal is likely connected with inversion of normal to so-called reverse micelles (surface hydrocarbon chains and polar groups becoming directed toward the micelle interior) after changing the hydrophilic/hydrophobic substances ratio in the system (Kelker & Hatz, Citation1980; Gabarayeva & Hemsley, Citation2006, ). The functional meaning of micelle-units re-arrangement is that hydrocarbon centres of micelles allow the passage of hydrophobic substances from the anther loculus to the microspore cytoplasm. After inversion, the hydrophilic centres allow the passage of water-soluble substances in the second period of microspore development, when the volume of microspores increases considerably. This is in good accordance with the idea of Rowley that the developing exine is a living, dynamic system, and that the glycocalyx units are plasmodesmata-like structures, confirmed by experiments with tracers (Rowley et al., Citation2003).
Honeycomb exine patterns, shown in transverse sections in Classopollis by Rowley (Citation1990) and later by Gabarayeva and Grigorjeva (Citation2002, Citation2004) in Stangeria eriopus (Kunze) Nash and Encephalartos altenstenii Lehm., clearly demonstrate close packing of cylindrical micelles-tufts in the glycocalyx layer – the next self-assembling micellar phase, the so-called ‘middle’ mesophase. This pattern is visible in transverse sections of the glycocalyx in any species, but it is especially distinct in the two cycads mentioned, because SP accumulates only on the sheaths of every cylindrical micelles. However, in most species, only few tuft-micelles accumulate SP. The orderly-arranged glycocalyx (B, C) is especially illustrative of how it is constructed. Some cylindrical units are in the process of SP accumulation, whereas others in between are rather transparent, with a distinct pattern of a spiral or cross banding and dark cores. The capability to accept SP belongs apparently to those units, which have receptors for SP accumulation [cf. receptor-dependent SP in Skvarla and Rowley (Citation1987) and Rowley and Claugher (Citation1991)]. After chemical degradation, SAPs were found to be connected like strings of beads, coiled around exine components in Nuphar (Rowley et al., Citation1995), Lavatera arborea L. (Gabarayeva et al., Citation2003a ) and in Cabomba aquatica Aubl. (Gabarayeva et al., Citation2003b ), or as a system of interconnected hollow spaces, corresponding to receptive sites (Blackmore & Barnes, Citation1987, Citation1990). SAPs have been revealed also after conventional fixation in cycads (Gabarayeva & Grigorjeva, Citation2002, Citation2004) and in Symphytum officinale L. (Gabarayeva et al., Citation2011). In general, the observation of SAPs in ontogeny is a rare phenomenon, because initial SP accumulation proceeds very fast and masks the SAPs. In Magnolia sieboldii, we have been lucky to observe them as dark spots at one stage only (C).
Glycocalyx units are rooted in the microspore cytoplasm, probably via cytoskeletal microfilaments (A). The same structures are visible in the tetrad period of Trevesia burckii Boerl. (Gabarayeva et al., Citation2009a ) and in Symphytum (Gabarayeva et al., Citation2011). This phenomenon could be connected with the cytoskeleton-extracellular matrix continuum (Wang et al., Citation1993) and the tensegrity phenomenon (Ingber & Jamieson, Citation1985; Ingber, Citation1993; Gabarayeva et al., Citation2009a ). In an earlier study, Abadie et al. (Citation1986–1987, p. 8) wrote: ‘… at tetrad stage, microfilamentous structures are detectable without any interruption running from the deep cytoplasm to the callosic wall …’, considering this continuum to be connected with transfer of energy and macromolecules. This phenomenon seems to be common during microspore development, but its meaning is still unclear.
Endexine
The foot layer and endexine in Magnolia sieboldii are formed at the beginning of the free microspore period almost simultaneously with insignificant advance of the foot layer. First appearing in fragments (D), they soon develop a continuous layer (E, F). This layer is thickened only from the outer ectexinous part, which actually represents the foot layer and gradually becomes very thick (E, F). Such an asymmetrical construction of the basal part of ectexine is a general feature for many species from different taxa. This and some other reasons led Nabli (Citation1975) to the conclusion that the white line is an area between ectexine and endexine and that the inner lamella is the primordial lamella of endexine. The second endexine lamella in Magnolia sieboldii is rather thick and well-distinguishable in the developmental process. In fact, the second lamella is the last one as is in Magnolia delavayi (A), Michelia fuscata (B), Manglietia tenuipes (D, E) and Liriodendron chinense (Gabarayeva, Citation1996, figures 22, 23). In the course of microspore maturation and development of the intine layers, the endexine lamellae become less and less distinguishable and during the pollen grain stage are actually difficult to observe. This may be the reason why earlier ideas, influenced by the analysis of mature pollen grains, denied the presence of endexine in archaic angiosperms. Taylor and Osborn (Citation2006) showed well-discernible endexine lamellae in the course of exine development in Brasenia emphasising the importance of ontogenetic data. Archaic angiosperms are especially interesting by various unexpected and unusual features such as columellae in developing tetrad microspores of Hedycarya arborea Forst., termed ‘radial processes’ by Sampson (Citation1977, ) that have distinct white lines in the centre, a feature typical rather for tangentially oriented endexine lamellae.
Currently, we interpret the nature of endexine lamellae with white lines as representation of the sequence end-point of transitory self-assembling micellar mesophases (laminate or so-called neat micelles, appearing in the periplasmic space as the concentration of glycoprotein surfactants increases). Neat micelles consist of two bilayers of surfactants with a hydrophilic gap in between. Two or more neat micelles arranged parallel and separated by gaps form a stack (Hemsley & Gabarayeva, Citation2007, ), which, filled with water-based periplasmic medium, appear in transmission electron microscopy as white lines. Tripartite lamellae are a common feature for all plant groups including the sporoderm of spore plants and gymnosperms. The widespread occurrence of these structures in nature clearly points to a common origin of this phenomenon and may imply a simple and universal mechanism for their formation. Scott (Citation1994) suggested that tripartite lamellae are formed by self-arrangement of long molecules with hydrophilic-hydrophobic ends.
Our interpretation of the endexine lamellae differs slightly from that of Rowley (Citation1987–1988, plate 7, figure 40) and Rowley and Skvarla (Citation2006), who see the white lines as a the result of broken and repaired radially oriented tufts, in a strict sense not as real structures, but rather as a result of structure perturbation. In our interpretation, the white lines are gaps between bilayers of radially-oriented cylindrical micelles and are a regular intrinsic feature of neat micelles. More important is the flexibility and permeability of the layers of this micelle system to some but not all substances, whereby they can act as selective filter in substance transfer from the anther fluid to the microspore cytoplasm, confirming Rowley's idea that endexine lamellae are flexible, can move and act as a pump for nutrients to developing microspores (Rowley, Citation1987–1988).
Intine ‘under question’
Tests on intine
The first layer appearing after endexine establishment consists of granular units that gradually accumulate in the periplasmic space (). This layer was attributed to the first layer of intine in all investigated Magnoliaceae (Gabarayeva, Citation1986b , Citation1987c , Citation1991a , Citation1996). To corroborate this, mature pollen grains were acetolised and in fact, this is the case (D, E). Hence, the granular layer is true intine. However, El-Ghazaly et al. (Citation2000), investigating selected stages of development in a number of species including Magnolia grandiflora L., did not attribute this granular layer to either ectexine or endexine, and considered it ‘a transitory layer’. This layer in Magnolia was resistant to acetolysis (El-Ghazaly & Huysmans, Citation2001), but the authors refrained from illustrating the finding. In contrast, Galati et al. (Citation2011) were able to show the vulnerability of this granular layer with the acetolysis test.
To confirm our opinion, we made an acetolysis test with mature pollen grains of Magnolia sieboldii (see C), and used a simple method to detect the endexine by staining with potassium permanganate (Weber & Ulrich, Citation2010). With glutar-osmium fixation and conventional staining with uranyl acetate and lead citrate, we got pronounced contrast of the granular layer (B–H). When replacing the conventional staining by potassium permanganate, the layer is hard to see and the boundaries of granules are not visible. If this layer belonged to endexine, the result of staining would be much stronger.
The first globular layer of intine is formed by large Golgi vesicles. It is very likely that these globular and nearly-globular units represent spherical micelles. The delay of the intine accumulation in the aperture site is the consequence of the distal fold formation at the early free microspore stage. For some reasons, the pressure of the medium in the periplasmic space under this thinnest region of the exine appeared to be lower than elsewhere, resulting in the formation of the fold and squeezing the microspore cytoplasm inwards (B). The vesicles observed in the periplasmic space in the aperture site at the middle tetrad stage (F) very likely play some role in this change of pressure. Rupture of these large vesicles with subsequent delivery of their contents to the loculus at the late tetrad stage with its thin tetrad callose envelope could cause a local pressure loss in the periplasmic space and the folding (adhesion) of the thin apertural exine. Reversion in the ectexine contrast, which becomes weak in mature pollen grains in comparison with intine contrast, is a well-known phenomenon for most species (see A, C–E).
Chain-mail and ‘zebra’ endoplasmic reticulum in Magnoliaceae
Relatively unusual aggregates of endoplasmic reticulum persist in the microspore cytoplasm during the tetrad and early post-tetrad periods: chain-mail endoplasmic reticulum (, , , , B, ), ‘zebra’ endoplasmic reticulum (B) and transition between chain-mail endoplasmic reticulum and ‘zebra’ endoplasmic reticulum (B). The most unusual form, the ‘zebra’ type, described earlier in Liriodendron chinense microspores (Gabarayeva, Citation1990, Citation1991b ), got its name from the alternating dark and light stripes. This aggregated endoplasmic reticulum very likely appears at the base of bicontinuous double-labyrinth structures with alternation of water/oil phases, a kind of complex micelles (Hemsley & Gabarayeva, Citation2007, ). The chain-mail endoplasmic reticulum is most probably a regular bicontinuous structure with minimal interface between two equally interpenetrating sub-volumes, the so-called periodical surface of Schwarz (Hemsley & Gabarayeva, Citation2007, ). When sectioned, this structure appears as a combination of rings and saddle-like (sinusoid) profiles, often seen in contact with usual cisternae of rough endoplasmic reticulum or smooth endoplasmic reticulum, and, via the latter, with the plasma membrane (F). Judging by B, these macrostructures are associated and can easily change one into another.
Many suggestions exist for probable functions of chain-mail endoplasmic reticulum with emphasis upon synthesis and fast excretion of different substances (Eymé, Citation1967; Wooding, Citation1967; Mepham & Lane, Citation1970; Esau & Hoefert, Citation1980; Gabarayeva, Citation1986a , Citation1991b ; Gabarayeva & Hemsley, Citation2006). Sometimes supposed to be an artefact of fixation, repeated observations of this type of endoplasmic reticulum, particularly in Magnoliaceae, reject such a supposition, these unusual aggregates being apparently typical of representatives of Magnoliaceae. We detected both types of aggregates in developing microspores of species from other families (Trevesia, Chamaedorea, Acer, Symphytum, Swida), but they were not as numerous as in species of Magnoliaceae and we did not describe them in respective publications. However, visible structures serving as framework for chain-mail and ‘zebra’ endoplasmic reticulum included clusters of curved cylindrical micelles in the microspore cytoplasm of Trevesia (Gabarayeva et al., Citation2009b , plate 2, , ) and bundles of liquid crystals in the tapetal cytoplasm of Persea (Gabarayeva et al., Citation2010, ).
We suggest that the function of these complex endoplasmic reticulum-aggregates persisting in the microspore cytoplasm mainly during the tetrad period could be the synthesis of sporopollenin precursors. It is clear that the glycocalyx facilitates the first deposition of sporopollenin monomer and that the uneven distribution of polymer initiation sites within the glycocalyx causes the exine pattern. Recent studies of several species revealed that the synthesis of both most probable sporopollenin precursors, i.e. fatty acids and phenylpropanoids, takes place in the tapetum during the tetrad period (Piffanelli et al., Citation1998), but it has not been shown in other species that such a synthesis is impossible in the tetrad microspores themselves. Moreover, in Lycopodium, the bulk of sporoderm material seems to be derived from the spore cytoplasm (Uehara & Kurita, Citation1991). Evidence exists that sporopollenin can be produced both from the tapetum and the micropore. We believe that at least a partial source of the sporopollenin monomers in the tetrad period is the microspore itself (Echlin, Citation1971; Heslop-Harrison, Citation1976; Dickinson, Citation1976b ; Dickinson & Potter, Citation1976; Audran, Citation1981; Gabarayeva, Citation1991a , Citation1991b , Citation1993b , Citation1996; Gabarayeva & Grigorjeva, Citation2002; Hemsley & Gabarayeva, Citation2007). Oxidation and other degradation experiments (Southworth, Citation1974, Citation1986; Rowley & Dahl, Citation1977; Rowley & Prijanto, Citation1977; Blackmore & Claugher, Citation1987; Blackmore, Citation1990; Rowley & Claugher, Citation1991; Gabarayeva et al., Citation2003a ) have shown that some differences in the chemical composition of sporopollenin really exist and may also be indicative of the microspore sporopollenin monomer source in the tetrad period.
Atypical microspores
Some microspores of Magnolia sieboldii deviate from the normal pathway of development and lack the glycocalyx almost completely (A, B). Such atypical microspores accumulate SP in an abnormal way by droplets (A, B). Although different aberrations in exine structure in Arabidopsis mutants are explainable by gene mutations (Paxson-Sowders et al., Citation1997, Citation2001; Ariizumi et al., Citation2004, Citation2005, Citation2008; Ariizumi & Toriyama, Citation2011), this is not the case in Magnolia sieboldii, where all the material was collected on the same plant. The appearance of atypical microspores may be connected with the simultaneous appearance of atypically hyperactive tapetal cells with needle-like crystals within the mitochondria and vacuoles, as well as plenty of osmiophilic droplets on the surface of the plasma membrane (C). Similar needle-like crystals were also found in double-membrane organelles in some abnormally developing microspores of Chamaedorea microspadix Burret (Gabarayeva & Grigorjeva, Citation2010).
The cases with under-developed or lacking microspore glycocalyx are of special interest because they once more demonstrate the importance of the normal glycocalyx development for exine pattern formation. A number of studies on Arabidopsis mutants have shown that an aberrant primexine matrix (=glycocalyx) results in a distorted exine (Paxson-Sowders et al., Citation2001; Ariizumi et al., Citation2004, Citation2005, Citation2008; Guan et al., Citation2008; Chang et al., Citation2011). Disturbances in SP monomer synthesis also lead to different abnormalities of exine structure (Dobritsa et al., Citation2009, Citation2011). The key role of the callose jacket in the tetrad period for exine pattern formation was evidenced by studies with callose-deficient mutants (Dong et al., Citation2005; Nishikawa et al., Citation2005).
We believe that in genetically altered mutants with their aberrant biosynthesis a change in the concentrations of the substances in the periplasmic space occurs. This unbalance leads to an under-developed or completely absent glycocalyx and, thus, to aberrant exine patterning or random SP accumulation. Another explanation is probably the late development of some tetrads in many species in comparison with the bulk. Such atypical tetrad microspores are very young post-meiotic microspores, for which many osmiophilic droplets on the plasma membrane are a typical transitive feature.
Unusual plastids
Plastids with numerous spherical osmiophilic globule-inclusions, permanently associated with rough endoplasmic reticulum and smooth endoplasmic reticulum cisternae and with chain-mail endoplasmic reticulum, are far from being typical of developing microspores (). Such osmiophilic inclusions, coupled with reticular sheaths around plastids (see also A, B), are not typical either for starch-containing amiloplasts or for protein-containing leucoplasts. The deposits, which stain with osmium tetroxide, are most likely phenolic substances (Alfenito et al., Citation1998; Muravnik, Citation2007). We suppose that the plastid osmiophilic inclusions can be related to the synthesis of SP integral constituents. The smooth endoplasmic reticulum may play a significant role in the synthesis and transport of phenolic substances (Wagner & Hrazdina, Citation1984; Schopker et al., Citation1995; Muravnik, Citation2007). Enzymes of phenylpropanoid metabolism were found in the endoplasmic reticulum of Arabidopsis thaliana (L.) Heynh. (Burbulis & Winkel-Shirley, Citation1999) and Nicotiana tabacum L. (Achnine et al., Citation2004). Here, multi-enzyme complexes or metabolons, situated on reticular membranes, are responsible for the assembly of flavonoids and phenylpropanoids. Leucoplasts take part in the synthesis of different phenolics, including p-coumaric acid and esters of hydroxycinnamic acids (Goodwin & Mercel, Citation1983; Zaprometov, Citation1993). It could be that either plastids contain the necessary multi-enzyme complexes and are capable of synthesising phenolics, or that plastids synthesise phenolic precursors, which are transported into associated endoplasmic reticulum cisternae for further modification (Muravnik & Shavarda, Citation2011). The latter view coincides with the view of Turner et al. (Citation2000) that some ultrastructural characteristics may be linked to specialisation for secretion of phenolic compounds: a dense network of endoplasmic reticulum associated with leucoplasts in the form of reticular sheaths.
These ultrastructural characteristics were also evidenced for microspores of Magnoliaceae (). When plastids are in close association with endoplasmic reticulum cisternae, these endoplasmic reticulum/plastid-complexes may facilitate removal of secondary metabolites from plastids (Turner et al., Citation2000). In this case, endoplasmic reticulum and especially chain-mail endoplasmic reticulum, may play a role in delivery of these metabolites up to the cell surface. However, endoplasmic reticulum and its complex aggregates may synthesise and secrete these metabolites themselves and deposit the surplus in plastids. At later free microspore stages, the chemical composition of the plastid inclusions changes as judged by their contrast: They appear electron translucent (, ).
Conclusions
The ectexine of Magnolia sieboldii develops on the glycocalyx (=primexine matrix) during the tetrad period with the exception of the foot layer. Early developmental stages of the ectexine comprise roundish units on the plasma membrane, gradually accumulating and forming a grainy layer, which we interpret as spherical micelles. At the middle tetrad stage, this layer undergoes a re-arrangement resulting in the appearance of rod-like units. These rod-like units or tufts are most probably the next micellar mesophase, i.e. cylindrical micelles. At the same time, first accumulation of sporopollenin proceeds on cylindrical tuft-micelles and procolumellae appear. They are thin and many of them are bent. The entire primexine layer has a highly-ordered structure. It consists of hexagonally-packed cylindrical units, standing on the microspore plasma membrane on their butt-ends. Such a substructure corresponds to the so-called middle or hexagonal micellar mesophase. At late tetrad stage, procolumellae become distinct and a thin tectum appears. After the release from the tetrads, young free microspores develop the foot layer and one or two endexine lamellae, which are rather thick and have typical central white lines. These white lines appear most probably as part of laminate micelles, which have typical gaps between bilayers. Simultaneously, the tectum, columellae and the foot layer become very thick as a consequence of massive accumulation of sporopollenin of tapetal origin. A typical feature of the young free microspores is the formation of a distal fold at the aperture site, where the exine consists only of the foot layer and the endexine lamellae. The ontogenetic data on the presence of the endexine in basal angiosperms do not support the hypothesis of its secondary evolutionary origin in the flowering plants. The intine is three-layered. The first layer is granular, the second is radially-channelled and the third is fine-fibrillar. The development of the intine-1 in the aperture region is late in comparison with inapertural regions, because the distal fold, appearing at the previous stage, prevents its accumulation. Sporopollenin accumulation tectum, columallae and foot layer in mature pollen is so intensive that rather short columellae often look like granules and the endexine often is completely obscure. The same problem is encounered with other Magnoliaceae. This illustrates the necessity of ontogenetic studies for the proper evaluation of exine structure. This study again confirms the previously suggested hypothesis that a considerable part of the developmental events can be regarded as an unfolding sequence of self-assembling micelle mesophases in the colloidal system of the periplasmic space. Evidently, initial parameters are genetically controlled; then self-assembly realises a design. Unusual macro-aggregates of endoplasmic reticulum (chain-mail endoplasmic reticulum and ‘zebra’ endoplasmic reticulum) in the microspore cytoplasm are characteristic for members of Magnoliaceae. They form on the framework of more complex micelles, bicontinuous double-labyrinth structures, and are in close association with plastids and the plasma membrane. Their probable role is the synthesis of sporopollenin precursors/monomers during the tetrad period. These macrostructures disappear after releasing microspores from the tetrads, when the access of sporopollenin of tapetal origin becomes free.
Acknowledgements
This study was supported by grant RFBR No. 11-04-00462 to NG. The authors wish to thank Peter Tzinman for assistance with the Hitachi H-600 TEM. They are also very grateful to Bruce Sampson for language corrections and appreciate the suggestions of the reviewers, which helped to improve the manuscript.
Notes
References
- Abadie , M. , Hideux , M. and Rowley , J. R. 1986–1987 . Ultrastructural cytology of the anther. II. Proposal for a model of exine considering a dynamic connection between cytoskeleton, glycolemma and sporopollenin-synthesis . Annales des Sciences Naturelles, Botanique , 13 : 1 – 16 .
- Achnine , L. , Blancaflor , E. B. , Rasmussen , S. and Dixon , R. A. 2004 . Colocalization of l-phenylalanine ammonia-lyase and cinnamate 4-hydroxylase for metabolic channeling in phenylpropanoid biosynthesis . Plant Cell , 16 : 3098 – 3109 .
- Alfenito , M. R. , Souer , E. , Doodman , C. D. , Buell , R. , Mol , J. , Koes , R. and Walbot , V. 1998 . Functional complementation of antocyanin sequestration in the vacuole by widely divergent glutathione S-transferases . Plant Cell , 10 : 1135 – 1149 .
- Ariizumi , T. and Toriyama , K. 2011 . Genetic regulation of sporopollenin synthesis and pollen exine development . Annual Review of Plant Biology , 62 : 1.1 – 1.24 .
- Ariizumi , T. , Hatakeyama , K. , Hinata , K. , Sato , S. , Kato , T. , Tabata , S. and Toriyama , K. 2005 . The HKM gene, which is identical to the MS1 gene of Arabidopsis thaliana, is essential for primexine formation and exine pattern formation . Sexual Plant Reproduction , 18 : 1 – 7 .
- Ariizumi , T. , Kawanabe , T. , Hatakeyama , K. , Sato , S. , Kato , T. , Tabata , S. and Toriyama , K. 2008 . Ultrastructural characterization of exine development of the transient defective exine-1 mutant suggests the existence of a factor involved in constructing reticulate exine architecture from sporopollenin aggregates . Plant Cell Physiology , 49 : 58 – 67 .
- Ariizumi , T. , Hatakeyama , K. , Hinata , K. , Inatsugi , R. , Nishida , I. , Sato , S. , Kato , T. , Tabata , S. and Toriyama , K. 2004 . Disruption of the novel plant protein NEF1 affects lipid accumulation in the plastids of the tapetum and exine formation of pollen, resulting in male sterility in Arabidopsis thaliana . Plant Journal , 39 : 170 – 181 .
- Audran , J.-C. 1981 . Pollen and tapetum development in Ceratozamia mexicana (Cycadaceae): Sporal origin of the exinic sporopollenin in cycads . Review of Palaeobotany and Palynology , 33 : 315 – 346 .
- Blackmore , S. 1990 . Sporoderm homologies and morphogenesis in land plants, with a discussion of Echinops sphaerocephala (Compositae) . Plant Systematics and Evolution , 5 : 1 – 12 .
- Blackmore , S. and Barnes , S. H. 1987 . Pollen wall morphogenesis in Tragopogon porrifolius L. (Compositae: Lactuceae) and its taxonomic significance . Review of Palaeobotany and Palynology , 52 : 233 – 246 .
- Blackmore , S. and Barnes , S. H. 1990 . “ Comparative studies of mature and developing pollen grains ” . In Scanning electron microscopy in taxonomy and functional morphology , Edited by: Claugher , D. 1 – 21 . Oxford : Clarendon Press . Systematics Association Special Volume No. 41
- Blackmore , S. and Claugher , D. 1987 . Observations of the substructural organization of the exine in Fagus sylvatica L. (Fagaceae) and Scorzonera hispanica L. (Compositae: Lactuceae) . Review of Palaeobotany and Palynology , 53 : 175 – 184 .
- Blackmore , S. , Wortley , A. H. , Skvarla , J. J. , Gabarayeva , N. and Rowley , J. R. 2010 . Developmental origins of structural diversity in pollen walls of the Compositae . Plant Systematics and Evolution , 284 : 17 – 32 .
- Blackmore , S. , Wortley , A. H. , Skvarla , J. J. and Rowley , J. R. 2007 . Pollen wall development in flowering plants . New Phytologist , 174 : 483 – 498 .
- Burbulis , I. E. and Winkel-Shirley , B. 1999 . Interactions among enzymes of the Arabidopsis flavonoid biosynthetic pathway . Proceedings of the National Academy of Sciences, USA , 96 : 12929 – 12934 .
- Chang , H.-S. , Zhang , C. , Chang , Y.-H. , Zhu , J. , Xu , X.-F. , Shi , Z.-H. , Zhang , X-L. , Xu , L. , Huang , H. , Zhang , S. and Yang , Z.-N. 2011 . No primexine and plasma membrane undulation (NPU) is essential for primexine deposition and plasma membrane undulation during microsporogenesis in Arabidopsis thaliana . Plant Physiology , 158 : 264 – 272 .
- Collinson , M. E , Hemsley , A. R. and Taylor , W. A. 1993 . Sporopollenin exhibiting colloidal organization in spore walls . Grana, Supplement , 1 : 31 – 39 .
- Dickinson , H. G. 1976a . The deposition of acetolysis-resistent polymers during the formation of pollen . Pollen et Spores , 18 : 321 – 334 .
- Dickinson , H. G. 1976b . “ Common factors in exine deposition ” . In The evolutionary significance of the exine , Edited by: Ferguson , I. K. and Muller , J. 67 – 87 . London : Academic Press .
- Dickinson , H. G. and Potter , U. 1976 . The development of patterning in the alveolar sexine of Cosmos bipinnatus . New Phytologist , 76 : 543 – 550 .
- Dinis , A. M. and Mesquita , J. F. 1993 . The F-actin distribution during microsporogenesis in Magnolia soulangeana Soul. (Magnoliaceae) . Sexual Plant Reproduction , 6 : 57 – 63 .
- Dinis , A. M. and Mesquita , J. F. 1994 . Ultrastructural and cytochemical evidence for the presence of peroxisomes in the generative cell of Magnolia × soulangeana pollen grains . Annals of Botany , 73 : 83 – 90 .
- Dobritsa , A. A. , Geanconteri , A. , Shrestha , J. , Carlson , A. , Koovers , N. , Coerper , D. , Urbanczyk-Wochniak , E. , Bench , B. J. , Sumner , L. W. , Wanson , R. and Preuss , D. 2011 . A large-scale genetic screen in Arabidopsis to identify genes involved in pollen exine production . Plant Physiology , 157 : 947 – 970 .
- Dobritsa , A. A. , Shrestha , J. , Morant , M. , Pinot , F. , Matsuno , M. , Swanson , R. , Møller , B. L. and Preuss , D. 2009 . CYP704B1 is a long-chain fatty acid ω-hydroxylase essential for sporopollenin synthesis in pollen of Arabidopsis . Plant Physiology , 151 : 574 – 589 .
- Dong , X. Y. , Hong , Z. L. , Sivaramakrichnan , M. , Mahfouz , M. and Verma , M. P. S. 2005 . Callose synthase (CalS5) is required for exine formation during microgametogenesis and for pollen viability in Arabidopsis . Plant Journal , 42 : 315 – 328 .
- Doyle , J. A. 2005 . Early evolution of angiosperm pollen as inferred from molecular and morphological phylogenetic analysis . Grana , 44 : 227 – 251 .
- Doyle , J. A. 2009 . Evolutionary significance of granular exine structure in the light of phylogenetic analyses . Review of Palaeobotany and Palynology , 156 : 198 – 210 .
- Dunbar , A. and Rowley , J. R. 1984 . Betula pollen development before and after dormancy: Exine and intine . Pollen et Spores , 26 : 299 – 338 .
- Echlin , P. 1971 . “ The role of the tapetum during microsporogenesis of angiosperms ” . In Pollen development and physiology , Edited by: Heslop-Harrison , J. 41 – 61 . London : Butterworths .
- El-Ghazaly , G. and Huysmans , S. 2001 . Re-evaluation of a neglected layer in pollen wall development with comments on its evolution . Grana , 40 : 3 – 16 .
- El-Ghazaly , G. and Jensen , W. A. 1987 . Development of wheat (Triticum aestivum) pollen. II. Histochemical differentiation of wall and Ubish bodies during development . American Journal of Botany , 74 : 1396 – 1418 .
- El-Ghazaly , G. , Moate , R. , Huysmans , S. , Skvarla , J. and Rowley , J. R. 2000 . “ Selected stages in pollen wall development in Echinodorus, Magnolia, Betula, Rondeletia, Borago and Matricaria ” . In Pollen and spores: Morphology and biology , Edited by: Harley , M. M. , Morton , C. M. and Blackmore , S. 17 – 29 . Whitstable : Whitstable Printers Ltd .
- Esau , K. and Hoefert , L. L. 1980 . Endoplasmic reticulum and its relation to microtubulus in sieve elements of sugerbeet and spinach . Journal of Ultrastructural Research , 71 : 249 – 257 .
- Eymé , J. 1967 . Nouvelles observations sur l'infrastructure des tissues nectarigènes floraux . Le Botaniste , 50 : 169 – 184 .
- Gabarayeva , N. I. 1986a . The development of exine in Michelia fuscata (Magnoliaceae) in connection with changes of cytoplasmic organelles and tapetum . Botanicheski Zhurnal (Leningrad) , 71 : 311 – 322 . (in Russian, with English summary)
- Gabarayeva , N. I. 1986b . Ultrastructural study on the development of intine in Michelia fuscata (Magnoliaceae) in connection with changes of cytoplasmic organelles and tapetum . Botanicheski Zhurnal (Leningrad) , 71 : 416 – 428 . (in Russian, with English summary)
- Gabarayeva , N. I. 1987a . Ultrastructure and development of sporoderm in Manglietia tenuipes (Magnoliaceae) in the course of the tetrad period: The formation of primexine in connection with cytoplasmic organelles activity . Botanicheski Zhurnal (Leningrad) , 72 : 281 – 290 . (in Russian, with English summary)
- Gabarayeva , N. I. 1987b . Ultrastructure and development of endexine lamellae in Manglietia tenuipes (Magnoliaceae) in connection with the question about the presence of the endexine in primitive angiosperms . Botanicheski Zhurnal (Leningrad) , 72 : 1310 – 1317 . (in Russian, with English summary)
- Gabarayeva , N. I. 1987c . Ultrastructure and development of the pollen wall in Manglietia tenuipes (Magnoliaceae) the intine formation in connection with cytoplasmic organelles activity . Botanicheski Zhurnal (Leningrad) , 72 : 1470 – 1477 . (in Russian, with English summary)
- Gabarayeva , N. I. 1990 . On the site of synthesis of sporopollenin precursors in the developing pollen grains of Magnoliaceae representatives . Botanicheski Zhurnal (Leningrad) , 75 : 783 – 791 . (in Russian, with English summary)
- Gabarayeva , N. I. 1991a . Ultrastructure and development of exine and orbicules in Magnolia delavayi (Magnoliaceae) in the course of the tetrad and post-tetrad periods . Botanicheski Zhurnal (Leningrad) , 76 : 10 – 19 . (in Russian, with English summary)
- Gabarayeva , N. I. 1991b . “ Patterns of development in primitive angiоsperm pollen ” . In Pollen and spores: Patterns of diversification , Edited by: Blackmore , S. and Barnes , S. H. 257 – 268 . Oxford : Clarendon Press .
- Gabarayeva , N. I. 1992 . Sporoderm development in Asimina triloba (Annonaceae). I. The developmental events before callose dissolution . Grana , 31 : 213 – 222 .
- Gabarayeva , N. I. 1993a . Sporoderm development in Asimina triloba (Annonaceaе). II. The developmental events after callose dissolution . Grana , 32 : 210 – 220 .
- Gabarayeva , N. I. 1993b . Hypothetical ways of exine pattern determination . Grana , 33 ( Supplement 2 ) : 54 – 59 .
- Gabarayeva , N. I. 1995 . Pollen wall and tapetum development in Anaxagorea brevipes (Annonaceae): Sporoderm substructure, cytoskeleton, sporopollenin precursor particles and the endexine problem . Review of Palaeobotany and Palynology , 85 : 123 – 152 .
- Gabarayeva , N. I. 1996 . Sporoderm development in Liriodendron chinense (Magnoliaceae): A probable role of the endoplasmic reticulum . Nordic Journal of Botany , 16 : 1 – 17 .
- Gabarayeva , N. I. 2000 . “ Principles and recurrent themes in sporoderm development ” . In Pollen and spores: Morphology and biology , Edited by: Harley , M. M. , Morton , C. M. and Blackmore , S. 1 – 17 . Whitstable : Whitstable Printers Ltd .
- Gabarayeva , N. I. , Blackmore , S. and Rowley , J. R. 2003a . Observations on the experimental destruction and substructural organisation of the pollen wall of some selected gymnosperms and angiosperms . Review of Palaeobotany and Palynology , 124 : 203 – 226 .
- Gabarayeva , N. I. and El-Ghazaly , G. 1997 . Sporoderm development in Nymphaea mexicana (Nymphaeaceae) . Plant Systematics and Evolution , 204 : 1 – 19 .
- Gabarayeva , N. I. and Grigorjeva , V. V. 2002 . Exine development in Stangeria eriopus (Stangeriaceae): Ultrastructure and substructure, sporopollenin accumulation, the equivocal character of the aperture, and stereology of microspore organelles . Review of Palaeobotany and Palynology , 122 : 185 – 218 .
- Gabarayeva , N. I. and Grigorjeva , V. V. 2003 . Comparative study of the pollen wall development in Illicium floridanum (Illiciaceae) and Schisandra chinensis (Schisandraceae) . Taiwania , 48 : 147 – 167 .
- Gabarayeva , N. I. and Grigorjeva , V. V. 2004 . Exine development in Encephalartos altensteinii (Cycadaceae): Ultrastructure, substructure and the modes of sporopollenin accumulation . Review of Palaeobotany and Palynology , 132 : 175 – 193 .
- Gabarayeva , N. I. and Grigorjeva , V. V. 2010 . Sporoderm ontogeny in Chamaedorea microspadix (Arecaceae): Self-assembly as the underlying cause of development . Grana , 49 : 91 – 114 .
- Gabarayeva , N. I. , Grigorjeva , V. V. and Polevova , S. 2011 . Exine and tapetum development in Symphytum officinale (Boraginaceae): Exine substructure and its interpretation . Plant Systematics and Evolution , 296 : 101 – 120 .
- Gabarayeva , N. I. , Grigorjeva , V. V. and Rowley , J. R. 2003b . Sporoderm ontogeny in Cabomba aquatica (Cabombaceae) . Review of Palaeobotany and Palynology , 127 : 147 – 173 .
- Gabarayeva , N. I. , Grigorjeva , V. V. and Rowley , J. R. 2010 . A new look at sporoderm ontogeny in Persea americana: Micelles and the hidden side of development . Annals of Botany , 105 : 939 – 955 .
- Gabarayeva , N. I. , Grigorjeva , V. V. , Rowley , J. R. and Hemsley , A. R. 2009a . Sporoderm development in Trevesia burckii (Araliaceae). I. Tetrad period: Further evidence for participating of self-assembly processes . Review of Palaeobotany and Palynology , 156 : 211 – 232 .
- Gabarayeva , N. I. , Grigorjeva , V. V. , Rowley , J. R. and Hemsley , A. R. 2009b . Sporoderm development in Trevesia burckii (Araliaceae). II. Post-tetrad period: Further evidence for the participation of self-assembly processes . Review of Paleobotany and Palynology , 156 : 233 – 247 .
- Gabarayeva , N. I. and Hemsley , A. R. 2006 . Merging concepts: The role of self-assembly in the development of pollen wall structure . Review of Paleobotany and Palynology , 138 : 121 – 139 .
- Gabarayeva , N. I. and Rowley , J. R. 1994 . Exine development in Nymphaea colorata (Nymphaeaceae) . Nordic Journal of Botany , 14 : 671 – 691 .
- Gabarayeva , N. I. , Rowley , J. R. and Skvarla , J. J. 1998 . Exine development in Borago (Boraginaceae). 1. Microspore tetrad period . Taiwania , 43 : 203 – 214 .
- Gabarayeva , N. I. , Walles , B. , El-Ghazaly , G. and Rowley , J. R. 2001 . Exine and tapetum development in Nymphaea capensis (Nymphaeaceae): A comparative study . Nordic Journal of Botany , 21 : 529 – 548 .
- Goodwin , T. W. and Mercel , E. I. 1983 . Introduction to plant biochemistry , Oxford : Pergamon .
- Galati , B. G. , Zarlavsky , G. , Rosenfeldt , S. and Gotelli , M. M. 2011 . Pollen ontogeny in Magnolia liliflora Desr . Plant Systematics and Evolution , 29803 : 527 – 534 .
- Gottsberger , G. 1988 . The reproductive biology of primitive angiosperms . Taxon , 37 : 630 – 643 .
- Guan , Y.-F. , Huang , X.-Y. , Zhu , J. , Gao , J.-F. , Zhang , H.-X. and Yang , Z.-N. 2008 . Ruptured pollen grain1, a member of the MtN3/saliva gene family, is crucial for exine pattern formation and cell integrity of microspores in Arabidopsis . Plant Physiology , 147 : 852 – 863 .
- Gubatz , S. , Herminghaus , S. , Meurer , B. , Strack , D. and Wiermann , R. 1986 . The location of hydroxycinnamic acid amides in the exine of Corylus pollen . Pollen et Spores , 28 : 347 – 354 .
- Gubatz , S. and Wiermann , R. 1992 . Studies on sporopollenin biosynthesis in Tulipa anthers. 3. Incorporation of specifically labelled C-14 phenylalanine in comparison to other precursors . Botanica Acta , 105 : 407 – 413 .
- Gubatz , S. and Wiermann , R. 1993 . Studies on sporopollenin biosynthesis in Cucurbita maxima. 1. The substantial labelling of sporopollenin from Cucurbita maxima after application of [C-14] phenylalanine . Journal of Biosciences , 48 : 10 – 15 .
- Hemsley , A. R. , Collinson , M. E. and Brain , A. P. R. 1992 . Colloidal crystal-like structure of sporopollenin in the megaspore walls of recent Selaginella and similar fossil spores . Botanical Journal of Linnean Society , 108 : 307 – 320 .
- Hemsley , A. R. and Gabarayeva , N. I. 2007 . Exine development: The importance of looking through a colloidal chemistry “window” . Plant Systematics and Evolution , 263 : 25 – 49 .
- Heslop-Harrison , J. 1976 . “ The adaptive significance of the exine ” . In The evolutionary significance of the exine , Edited by: Ferguson , I. K. and Muller , J. 27 – 37 . London : Academic Press .
- Hesse , M. 1985 . Hemispherical surface processes of exine and orbicules in Calluna (Ericaceae) . Grana , 24 : 93 – 98 .
- Ingber , D. 1993 . Cellular tensegrity: Defining new rules of biological design that govern the cytoskeleton . Journal of Cell Science , 104 : 613 – 627 .
- Ingber , D. E. and Jamieson , J. D. 1985 . “ Cells as tensegrity structures: Architectural regulation of histo-differentiation by physical forces transduced over basement membrane ” . In Gene expression during normal and malignant differentiation , Edited by: Andersson , L. C. , Gahmberg , C. G. and Ekblom , P. 13 – 32 . Orlando , FL : Academic Press .
- Kelker , H. and Hatz , R. 1980 . Handbook of liquid crystals , Weinheim : Verlag Chemie .
- Mepham , R. H. and Lane , G. R. 1970 . Observation on the fine structure of the developing microspores of Tradescantia bracteata . Protoplasma , 70 : 1 – 20 .
- Muravnik , L. E. 2007 . Morphology and ultrastructure of the pericarp trichomes in Juglans species (Juglandaceae) in relation to synthesis of secondary metabolites . Botanicheski Zhurnal , 92 : 96 – 107 . (in Russian, with English summary)
- Muravnik , L. E. and Shavarda , A. L. 2011 . Pericarp peltate trichomes in Pterocarya rhoifolia: Histochemistry, ultrastructure and chemical composition . International Journal of Plant Sciences , 172 : 159 – 172 .
- Nabli , M. A. 1975 . Mise en évidence de deux lamelles primordiales, ectexinique et endexinique, dans l'exine de quelques Labiatae . Comptes Rendus de l'Académie des Sciences Paris, série D , 281 : 251 – 253 .
- Nishikawa , S. , Zinkl , G. M. , Swanson , R. J. , Maruyama , D. and Preuss , D. 2005 . Callose (β-1,3 glucan) is essential for Arabidopsis pollen wall patterning, but not tube growth . BMC Plant Biology , 5 : 22 – 30 .
- Paxson-Sowders , D. M. , Dodrill , C. J. , Owen , H. A. and Makaroff , C. A. 2001 . DEX1, a novel plant protein, is required for exine pattern formation during pollen development in Arabidopsis . Plant Physiology , 127 : 1739 – 1749 .
- Paxson-Sowders , D. M. , Owen , H. A. and Makaroff , C. A. 1997 . A comparative ultrastructural analysis of exine pattern development in wild-type Arabidopsis and a mutant defective in pattern formation . Protoplasma , 198 : 53 – 65 .
- Pettitt , J. M. 1979 . “ Ultrastructure and cytochemistry of spore wall morphogenesis ” . In The experimental biology of ferns , Edited by: Dyer , A. F. 211 – 252 . London : Academic Press .
- Pettitt , J. M. and Jermy , A. C. 1974 . The surface coats on spores . Botanical Journal of Linnean Society , 6 : 245 – 257 .
- Piffanelli , P. , Ross , J. H. E. and Murphy , D. J. 1998 . Biogenesis and function of the lipidic structures of pollen grains . Sexual Plant Reproduction , 11 : 65 – 80 .
- Rittscher , M. and Wiermann , R. 1988 . Studies on sporopollenin biosynthesis in Tulipa anthers. I. The development of an appropriate application method for radiolabeled precursors . Sexual Plant Reproduction , 1 : 125 – 131 .
- Rowley , J. R. 1971 . “ Implications on the nature of sporopollenin based upon pollen development ” . In Sporopollenin , Edited by: Brooks , J. , Grant , P. R. , Muir , M. , van Gijzel , P. and Shaw , G. 174 – 219 . London : Academic Press .
- Rowley , J. R. Lipopolysaccharide embedded within the exine of pollen grains . Proceedings of the 33rd Annual Meeting of the Electron Microscopy Society of America . August 11–15 1975 , Las Vegas , USA. pp. 572 – 573 .
- Rowley , J. R. 1987–1988 . Substructure within the endexine, an interpretation . Journal of Palynology , 23 ( 24 ) : 29 – 42 .
- Rowley , J. R. 1990 . The fundamental structure of the pollen exine . Plant Systematics and Evolution, Supplement , 5 : 13 – 29 .
- Rowley , J. R. and Claugher , D. 1991 . Receptor-independent sporopollenin . Botanica Acta , 104 : 316 – 323 .
- Rowley , J. R. and Dahl , A. O. 1977 . Pollen development in Artemisia vulgaris with special reference to glycocalyx material . Pollen et Spores , 19 : 169 – 284 .
- Rowley , J. R. , Dahl , A. O. , Sengupta , S. and Rowley , J. S. 1981 . A model of exine substructure based on dissection of pollen and spore exines . Palynology , 5 : 107 – 152 .
- Rowley , J. R. and Dunbar , A. 1970 . Transfer of colloid iron from sporophyte to gametophyte . Pollen et Spores , 12 : 305 – 328 .
- Rowley , J. R. and Flynn , J. J. 1968 . Tubular fibrils and the ontogeny of the yellow water lily pollen grain . Cell Biology , 39 : 159
- Rowley , J. R. , Flynn , J. J. and Takahashi , M. 1995 . Atomic force microscope information on pollen exine substructure in Nuphar . Botanica Acta , 108 : 300 – 308 .
- Rowley , J. R. and Prijanto , B. 1977 . Selective destruction of the exine of pollen grains . Geophytology , 7 : 1 – 23 .
- Rowley , J. R. and Rowley , J. S. 1998 . “ Stain reversal in pollen exines ” . In Current concepts in pollen-spore and biopollution research , Edited by: Dutta , N. M. , Gupta-Bhattacharya , S. , Mandal , S. and Bhattacharya , K. 223 – 232 . Houston , TX : Research Periodicals & Book Publishing House .
- Rowley , J. R. and Skvarla , J. J. 2006 . Pollen development in Epilobium (Onagraceae): Late microspore stages (a review) . Review of Paleobotany and Palynology , 140 : 91 – 112 .
- Rowley , J. R. , Skvarla , J. J. and El-Ghazaly , G. 2003 . Transfer of material through the microspore exine – from the loculus into the cytoplasm . Canadian Journal of Botany , 81 : 1070 – 1082 .
- Rowley , J. R. , Skvarla , J. J. and Pettitt , J. M. 1992 . Pollen wall development in Eucommia ulmoides (Eucommiaceae) . Review of Palaeobotany and Palynology , 70 : 297 – 323 .
- Sampson , F. B. 1977 . Pollen tetrads of Hedycarya arborea J. R. et G. Forst. (Monimiaceae) . Grana , 16 : 61 – 73 .
- Sampson , F. B. 2000 . Pollen diversity in some modern magnoliids . International Journal of Plant Sciences , 161 : S193 – S210 .
- Schopker , H. , Kneisel , M. , Beerhues , L. , Robenek , H. and Wiermann , R. 1995 . Phenylalanin ammonia-lyase and chalkone synthasein glands of Primula kewensis (W. Wats): Immunofluorescence and immunogold localization . Planta , 196 : 712 – 719 .
- Scott , R. J. 1994 . “ Pollen exine – the sporopollenin enigma and the physics of pattern ” . In Molecular and cellular aspects of plant reproduction , Edited by: Scott , R. J. and Stead , M. A. 49 – 81 . Cambridge : Cambridge University Press . Society for Experimental Biology Seminar Series 55
- Skvarla , J. J. and Rowley , J. R. 1987 . Ontogeny of pollen in Poinciana (Leguminosae). I. Development of exine template . Review of Palaeobotany and Palynology , 50 : 239 – 311 .
- Southworth , D. 1974 . Solubility of pollen exines . American Journal of Botany , 61 : 36 – 44 .
- Southworth , D. 1986 . “ Substructural organization of pollen exines ” . In Pollen and spores: Form and function , Edited by: Blackmore , S. and Ferguson , I. K. 61 – 69 . London : Academic Press .
- Taylor , M. L. and Osborn , J. M. 2006 . Pollen ontogeny in Brasenia (Cabombaceae, Nympheales) . American Journal of Botany , 93 : 344 – 356 .
- Turner , G. W. , Gershenzon , J. and Crotaux , R. B. 2000 . Development of peltate glandular trichomes of peppermint . Plant Physiology , 124 : 665 – 679 .
- Uehara , K. and Kurita , S. 1991 . Ultrastructural study on spore wall morphogenesis in Lycopodium clavatum (Lycopodiaceae) . American Journal of Botany , 78 : 24 – 36 .
- Wagner , G. J. and Hrazdina , G. 1984 . Endoplasmic reticulum as a site of phenylpropanoid and flavonoid metabolism in Hippeastrum . Plant Physiology , 74 : 901 – 906 .
- Walker , J. W. 1976 . “ Evolutionary significance of the exine in the pollen of primitive angiosperms ” . In The evolutionary significance of the exine , Edited by: Ferguson , I. K. and Muller , J. 251 – 308 . London : Academic Press .
- Wang , N. , Butler , J. P. and Ingber , D. E. 1993 . Mechano-transduction across the cell surface and through the cytoskeleton . Science , 260 : 1124 – 1127 .
- Weber , M. and Ulrich , S. 2010 . The endexine: A frequently overlooked pollen wall layer and simple method for detection . Grana , 49 : 83 – 90 .
- Wehling , K. , Niester , C. , Boon , J. J. , Willemse , M. T. M. and Wiermann , R. 1989 . p-Coumaric acid – a monomer in the sporopollenin skeleton . Planta , 179 : 376 – 380 .
- Wooding , F. B. 1967 . Endoplasmic reticulum aggregates of ordered structure . Planta , 76 : 205 – 208 .
- Xu , F.-X. and Kirchoff , B. K. 2008 . Pollen morphology and ultrastructure of selected species of Magnoliaceae . Review of Palaeobotany and Palynology , 150 : 140 – 153 .
- Zaprometov , M. N. 1993 . Phenolic compounds: Distribution, metabolism and functions in plants , Moscow : Nauka (in Russian) .