Abstract
The study assesses differences in the results retrieved by Hirst samplers of the same model in order to determine their sampling variability. Five seven-day Burkard spore traps were compared. Four were located at ground level at the same site, separated by less than 2 m from each other. The fifth was placed on a terrace 16 m above ground, close to the other four samplers. An automatic meteorological station at ground level close to the spore traps was working simultaneously. Sampling was done for 51 days. The daily and hourly pollen concentration data were compared and the pollen distribution over the microscope slide surface was analysed. There were highly statistically significant strong positive correlations between spore traps themselves, with equivalent measurements of the daily and hourly patterns. Differences in hourly values between samplers were greater than differences in daily values, and the hourly peaks did not always match among samplers, with typically lags of two or more hours between traps. Analysis of the surface deposition of pollen on the slides showed pollen loss towards the edges and pollen appearing even outside the slit intake width. As hourly peaks indicated a degree of variability, this type of data requires to be interpreted with relative caution.
As noted by Welam-Henningson and Ahlberg (Citation1994), there is a wide variety of samplers for monitoring the atmosphere aerobiologically, but only some of them have been tested completely, and it is widely accepted that there is a need for standardised methods and procedures. Spore traps based on the original design of Hirst (Citation1952) are amongst the most widely used in aerobiology. Two are currently commercially available, the Burkard and the Lanzoni models, both of which comply with the original specifications: air is sucked in at an intake flow rate of 10 l/min through a slit of 14 × 2 mm to impact on an adhesive-coated surface of 19 mm width.
The efficiency of this kind of spore trap was tested on an individual model in the original work (Hirst, Citation1952). Nevertheless, sampling variability of devices corresponding to the same model needs to be tested using various samplers. Comparisons using spatially separated devices of the same type have typically been performed with the samplers located in different towns, with distances ranging from 3 km to more than 2300 km (). Although some of these studies were performed in the United States (Kramer & Eversmeyer, Citation1984; Eversmeyer & Kramer, Citation1987; Frenz et al., Citation1997; Irdi et al., Citation2002; Khattab & Levetin, Citation2008), most were carried out in Italy (Caramiello et al., Citation1985; Palmas & Cosentino, Citation1990; Rizzi et al., Citation1992; Gottardini & Cristofolini, Citation1997; Arobba et al., Citation2000; Giorato et al., Citation2003), Spain (Galán et al., Citation1995a ; Trigo et al., Citation2000; Alcázar et al., Citation2009), Denmark (Strandhede & Wihl, Citation1981; Pedersen & Moseholm, Citation1993), Poland (Corden et al., Citation2002; Piotrowska, Citation2004), Switzerland (Leuschner & Boehm, Citation1981), France (Meiffren, Citation1988), Germany (Koch et al., Citation2000) and other European countries (Lejoly-Gabriel & Leuschner, Citation1983; Kupias et al., Citation1989; Jäger et al., Citation1996; Ekebom et al., Citation1997).
Table I. Published sampler comparisons sorted after distances
Comparisons have also been performed with devices of exactly the same model testing the effect of height, with heights ranging from ground level to 55 m (). Most of these studies compared two (Rantio-Lehtimäki et al., Citation1991; Galán et al., Citation1995b ; Leuschner, Citation1999; Bergamini et al., Citation2004) or three points (Bryant et al., Citation1989; Hart et al., Citation1994; Fiorina et al., Citation1999; Alcázar & Comtois, Citation2000). Only Chakraborty et al. (Citation2001) compared six points.
Table II. Published sampler comparisons sorted after height of placement
The general conclusion from distance comparisons is that the phenology of the sources and weather differences are sufficient to explain the variability of the results. The height comparisons show that pollen content generally decreases with height, although the contrary has sometimes been found to be the case for the smaller spores (Chakraborty et al., Citation2001).
A few comparisons of spore traps located in the same place have been carried out and, when Hirst spore traps were used, the comparisons were always done by two traps only. Eversmeyer and Kramer (Citation1987) performed an experiment using 15 Rotorod samplers on five towers 1 m apart from each other. The data were subjected to an analysis of variance (ANOVA), which showed that there were no significant differences between the measurements of samplers at the same height above the ground. However, significant differences in spore concentrations measured by samplers operating at different heights above ground level appeared when wind speed was larger than 5 m/s. The authors concluded that, although a single volumetric sampler will provide adequate measurements of spore concentrations, its placement should be selected with extreme care.
Pedersen and Moseholm (Citation1993) assessed the differences between two spore traps placed side by side (estimated distance from the photograph about 3 m). The work analysed pollen data for 20 days, including a comparison between results from three trained pollen counters, and a detailed examination under a microscope of 12 transverse strips corresponding to every second hour. An ANOVA showed that inter-counter differences contributed significantly to the pollen count variance while the traps themselves did not. There was, however, a day-trap interaction, which the authors attributed to either a problem of instrumental variation of the traps over time or to changes in weather conditions. They proposed that, although the increase in the area counted on the microscope slides would make the procedure more costly in time, it would reduce the error in the measurements. Irdi et al. (Citation2002) used two samplers separated at a distance of 1 m, collecting data over 14 consecutive days. Application of a t-test showed no statistically significant differences between the samplers, and a correlation analysis revealed their data to be strongly correlated. They also tested the transversal distribution of pollen and spores on the microscope slides, using data of one day for pollen and from another day for spores. The aim was to count ten adjacent longitudinal transects in the centre of the slide, which corresponded to strips of 4.6 × 48 mm for pollen and 1.87 × 48 mm for spores taken for from the total 19 × 48 mm surface area of the tape. An ANOVA indicated no statistically significant differences in the pollen grain transect counts, but significant differences in the fungal spore counts. Giorato et al. (Citation2003) compared eight weeks of data from two Lanzoni VPPS 2000 traps, 2 m apart. Applying a simple regression analysis, they found no statistically significant differences between the two sets of data. Khattab and Levetin (Citation2008) compared two Burkard spore traps placed side by side, but with different orifice sizes – one 14 mm × 2 mm and the other 14 mm × 0.5 mm. While the former was more efficient at trapping the larger particles such as Alternaria spores, the latter was more efficient for the smaller particles such as Penicillium-Aspergillus spores.
Other comparisons have compared results obtained from the use of different microscopes, i.e. light versus scanning electron microscopy (Jones et al., Citation2007), or pollen count precision (Comtois et al., Citation1999; Cariñanos et al., Citation2000). The former concluded that the efficiency of the light microscope is completely sufficient for aerobiological purposes, while the latter two studies found there to be a problem with the different methodological approaches that are used for counting, and that a consensus on unification is far from being reached.
The objective of the present work is to assess sampling variability with the use of seven-day Burkard samplers, using more than two spore traps and examining both daily and hourly variability, and with comparisons made at the same place with traps placed side by side and close to a meteorological station. We also analysed pollen distribution over the surface of the slides. The immediate reason for this experiment was to take advantage of the availability of a larger number of samplers prior to their definitive placement in their locations in the Extremadura Aerobiological Network (southwest Spain).
Material and methods
The spore traps and the meteorological station were located at the campus of the University of Extremadura in Badajoz, Spain (). Sampling was carried out at the Faculty of Sciences. Five seven-day Burkard spore traps were placed in the same location. Four of them (spore traps ST1–ST4) were at ground level, 2 m apart from each other. The fifth (spore trap STT) was located 16 m above the ground on a terrace close to the other samplers. The study period ran from 27 January to 18 March 2011, corresponding to a total of 51 days of sampling. At any given time during the study period there were at least three ground level samplers operating simultaneously. The terrace spore trap (STT) and one of the ground level traps (ST4) operated throughout the complete period ().
Figure 1. Map of the university campus showing the locations of the spore traps (circled red crosses; ST1–ST4, spore traps on ground level; STT, spore trap on the terrace) and the pollinating trees during the sampling period.
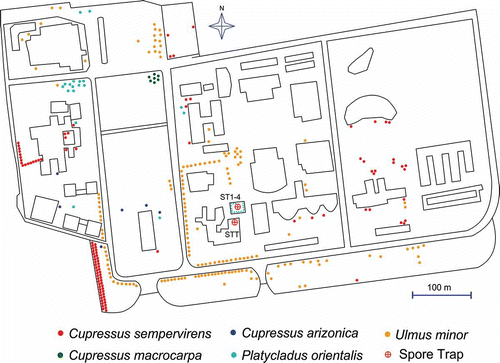
Figure 2. Sampling period data. Top: The intervals during which the five spore traps were used (ST1–ST4, spore traps on ground level; STT, spore trap on the terrace). Bottom: Daily temperatures and rainfall during the sampling period.
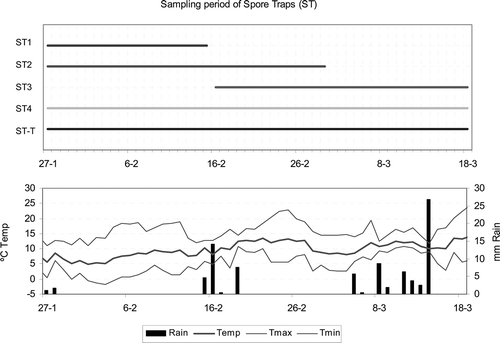
Weather data was provided by an automatic meteorological station located at ground level, 5.5 m from the spore traps. Petrolatum white (CAS number 8009-03-8) was used as adhesive. Four longitudinal scans taken in the centre of the microscope slide to minimise errors (Tormo et al., Citation1996) were counted at 400× magnification under light microscopy. The spore traps were checked using a flow meter at the beginning of the sampling period. Comparisons of the traps' daily and hourly pollen concentration data were made.
Since not all the spore traps were available all the time, three periods of time were analysed separately (see ) to compare the data provided by the different traps. In particular, four spore traps were compared for 20 days (27 January–15 February), another four for 14 days (16 February–1 March) and three other for 17 days (2 March–18 March).
The data were normalised by log transformation. Paired t-tests were performed to compare daily and hourly data for the different spore traps. Pearson correlation coefficients were calculated to study the relationships between the daily and hourly data of the traps. They were also calculated for the hourly data between the pollen concentrations (total, Cupressaceae and Ulmus) and the meteorological parameters (rainfall, temperature, relative humidity, wind speed and wind direction).
For the study of microscope slide surfaces, eight slides corresponding to the four samplers on the two days with maximum total pollen concentrations (12 February and 20 February) were selected. Pollen was counted under a Zeiss microscope at 100× magnification with an optical field diameter of 1.43 mm. The entire plastic strip surface with 13 longitudinal scans was counted. An ANOVA was applied to compare the longitudinal scan data.
Results
There were 14 days of rainfall during the study period (). Predominant wind direction was southwest with speed of less than 1 m/s, except for one rainy day (). The average hourly wind speeds and directions differed from night to day. Daytime wind speeds were greater and wind directions changed from predominantly southwest during the night to southeast during the day.
A total of 14 pollen types and Alternaria spores were registered. Cupressaceae and Ulmus pollen types accounted for at least 80% of the total pollen counts (; ). The paired daily data comparisons indicated no statistically significant differences among the ground level spore traps, but significant differences between the data for four of these traps and those for the trap on the terrace (; ). Because rainfall was mainly restricted to two wet spells, three periods of total and Cupressaceae type pollen concentrations are observed, although for Ulmus, the third period is less evident. The daily data of all the spore traps were strongly correlated (p < 0.001). Paired t-test comparisons were made for the hourly data of different intervals of the study period (). Significant differences between the terrace spore trap data and each of the ground level spore traps are evident as there are also significant differences between ground level spore traps (in particular, ST2-ST4 and ST3-ST4).
Table III. Average pollen concentrations (grains/m3) and Alternaria spores (spores/m3) in descending order in the study period for the five spore traps tested
Table IV. Statistically significant results (p = 0.05) of the paired t-test comparing daily pollen data
Table V. Results of the paired test between hourly data from spore traps. Only comparisons with at least one result statistically significant are included
Figure 4. Average concentrations of total, Cupressaceae and Ulmus pollen in three intervals of the study period for each spore trap (ST1–ST4, spore traps on ground level; STT, spore trap on the terrace).
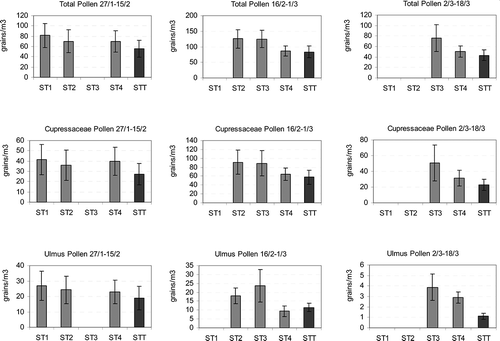
Lags reaching up to seven hours were observed in the appearance of the hourly pollen concentration peaks between the different ground level spore traps (). In Cupressaceae (), these lags were of one hour (days 10 February, 21 February), two hours (12 February), three hours (20 February, 7 March) and seven hours (8 March); in Ulmus (), they were of two hours (11 February, 23 February), three hours (7 February, 12 February), four hours (8 February) and five hours (26 February). Distinct differences were also recorded in the total amounts of pollen at hourly peaks (). Considering only the ground level spore trap data, these differences were doubled (Cupressaceae: 10 February and 8 March, Ulmus: 23 February) or tripled (Cupressaceae: 7 March); when the spore trap on the terrace was included, they were even larger. The correlation analysis applied to the hourly data revealed statistically significant positive correlations with temperature and wind speed, and was negatively correlated with relative humidity and wind direction, but no significant correlation with rainfall.
Table VI. Hourly concentrations of Cupressaceae and Ulmus pollen type on different days with the interval of hours that includes the hourly peak (in bold) for each spore trap
Figure 6. Hourly Cupressaceae pollen concentrations and wind speeds in the periods with maximum pollen concentrations.
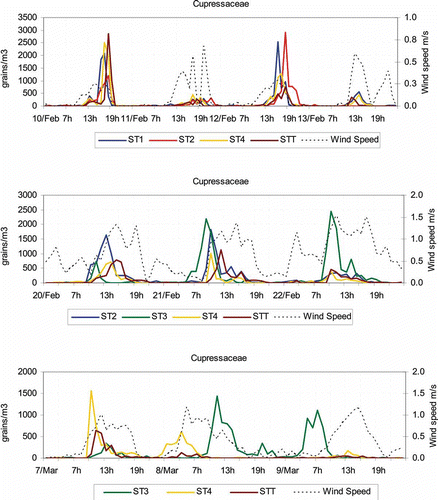
Figure 7. Hourly Ulmus pollen concentrations and wind speeds in the periods with maximum pollen concentrations.
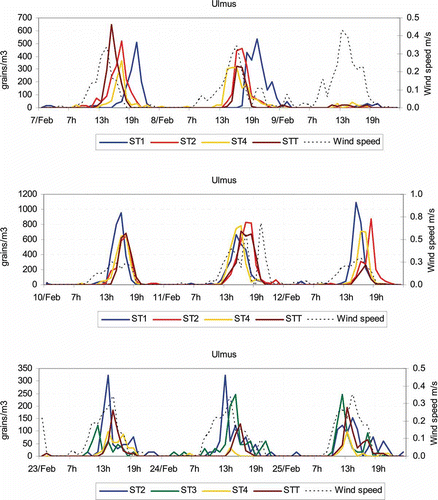
A total of 23 361 pollen grains were counted in the pollen distribution analysis over the surface of the eight slides corresponding to two days (). An ANOVA revealed no statistically differences between the slides (F = 1.505, p = 0.175). Nevertheless, comparisons between the 13 scans showed statistically significant differences (F = 9.173, p < 0.001). Tukey's post-hoc HSD test evidenced that the inner scans three to ten formed a homogeneous set statistically different from the outermost scans (1–2 and 11–13).
Discussion
Differences in data provided by different spore traps can be explained by variations in environmental conditions. Spatial separation may imply differences in sources and meteorological conditions that are reflected in phenological behaviour and pollen seasons (Piotrowska, Citation2004; Alcázar et al., Citation2009). Such spatial variations are more marked with latitudinal than with longitudinal differences because there are generally greater changes in weather from north to south than from east to west. Nevertheless, other geographical factors that can affect weather conditions and consequently pollen seasons must also be taken into account. Another potential source of variability is the land use (Corden et al., Citation2002). When more than two stations are compared, it is more difficult to draw any firm conclusions because more environmental factors may be involved, although it may be possible to identify certain trends if the study runs over several years (Leuschner & Boehm, Citation1981; Jäger et al., Citation1996).
There is an extensively confirmed general pattern of decreasing particle concentration with height (Rantio-Lehtimäki et al., Citation1991; Galán et al., Citation1995a ; Bergamini et al., Citation2004). However, differences can be observed in this pattern depending on the hour of the day. This has been explained by different distribution of respective sources (Alcázar & Comtois, Citation2000) or by, e.g. the proximity of buildings (Bryant et al., Citation1989). A particle-size dependence has been observed as well, smaller spores being dominant at larger heights, and larger spores and conidia more prevalent nearer to the ground (Chakraborty et al., Citation2001). In the present work, the counts on a terrace at a height of 16 m were on average 27% smaller than those at ground level, although it needs to be stressed that only one spore trap was used on the terrace.
When spore traps are operating together side by side, one would assume that the environmental conditions are the same and that differences between the spore traps are due to the accuracy of the traps themselves. There have been various statistical analyses comparing pollen data from two or more traps. A simple regression analysis (Giorato et al., Citation2003) only revealed the data to be correlated, but the differences in the amounts of pollen were not evaluated so that evidence for major variations between the traps could have been missed. A one-way ANOVA applied to normalised data only assessed differences in the means between populations, taking into account within-group and between-group variance (Irdi et al., Citation2002). However, data recorded sequentially over time with two or more spore traps are paired, because on the same day or at the same hour, two or more values are recorded at the same time, and these paired or multiple data should always be taken together in a statistical analysis. Trigo et al. (Citation2000) discussed in some depth the statistical methods useful in comparing data from different stations, considering both normal and non-normal statistics. The present results include both correlation analyses and paired comparisons. The daily data given by the four ground level spore traps were statistically equivalent, a conclusion consistent with the findings of the cited literature despite of the different statistics applied.
The hourly data exhibited statistically significant differences between spore traps placed side by side with difference up to seven hours for peaks of concentration. There was even an up to three-fold difference in the total amount of pollen recorded at those peaks. The peak pollen concentration hour depends on the source phenology and the wind, which distributes the grains. Wind speed seemed to strongly influence the pollen peaks (, ) and is significantly correlated with the counts of both pollen types studied. However, this relationship was not always clear. For example, the pollen counts were markedly reduced on 11 February and 13 February for hourly Cupressaceae pollen () and on 9 February for hourly Ulmus pollen () even though wind speeds were similar to the previous days.
In general, another potential cause of hourly variations is the distribution of the pollen sources in the area affecting the traps. In the present case Cupressaceae and Ulmus trees are fairly uniformly distributed in the university campus around the spore trap locations (), implying that the distribution of the pollen sources apparently did not affect the hourly peaks.
The distribution of the grains on the surface of the slides showed that much pollen is captured outside the 14 mm inlet width. Since the plastic tape is 19 mm wide and the microscopic field is only 1.43 mm wide, one can estimate that all the pollen in the 1st and 13th scans and about three-quarters of the 2nd and 12th scans came to lie outside the inlet width. This represents more than 12% of all pollen collected, much more than found in previous studies (Tormo et al., Citation1996), so that a definitive figure would probably call for further analysis. Although the ANOVA revealed scans three to ten to form a statistically homogeneous set, it also indicated the within-scan variance to be greater than the between-scan variance. A decrease of pollen from the centre to the edges of the slide was also observed (). In order to estimate this decrease, the average of the three scans in the centre of the slide (6–8) was calculated and compared with the average of the remaining ten scans on the outsides (1–5, 9–13): More than 50% of the pollen that should have been recorded was lost on these outer scans. This pattern of reduction in counts towards the edges of the slide has been noted previously (Tormo et al., Citation1996), but has been higher because all longitudinal scans were taken into account instead of only the four central scans. Moreover, the reduction would be the origin of the differences between transverse traverse counts and total slide counts found by Sterling et al. (Citation1999). These authors counted 82 longitudinal scans because they used 1000× instead of 100× magnifications. While Irdi et al. (Citation2002) expressed some doubt about this pattern, they analysed only one strip of 4.6 mm width for pollen and one of 1.87 mm width for spores from two slides. The ANOVA resulted in statistically significant differences for spores but not for pollen. However, these widths are insufficient to reveal the pattern with which the pollen is distributed over the slide. The decrease in pollen counts towards the edges of the slides observed in this and the previous work seems to reduce the efficiency of the trap at these sides of the slide.
Conclusions
There were no statistically significant differences observed among spore traps placed at ground level in terms of daily data, but some differences, however, with hourly data. At 16 m height, statistically significant differences in pollen counts occurred relative to the ground level traps, with a decrease of about 30%. Peak hourly pollen concentrations have seldom been recorded at the same time in various spore traps. In the present study, lags of up to seven hours were observed, and the peak hourly concentrations differed by factors of about two or three between traps. The spatial distribution of pollen and spores is far from homogeneous even within a separation distance as small as 2 m. Therefore, spore trap hourly data should be interpreted with caution, and hourly peak concentrations should be regarded only as no more than a rough approximation or estimation, which involves a major degree of uncertainty. We have confirmed previous observations of a decrease in pollen capture from the centre to the edge of the slide. Consequently, we would strongly recommend counting aerobiological particles captured in spore traps only in the middle of the slide to obtain comparable results.
Acknowledgements
This work was supported by the Regional Government, Junta de Extremadura (Spain), and by the European Regional Development through research projects PRI06A190 and PRI BS10008. The authors are grateful to Mari Cruz Lozano for her collaboration.
References
- Alcázar , P. and Comtois , P. 2000 . The influence of sampler height and orientation on airborne Ambrosia pollen counts in Montreal . Grana , 39 : 303 – 307 .
- Alcázar , P. , Stach , A. , Nowak , M. and Galán , C. 2009 . Comparison of airborne herb pollen types in Córdoba (southwestern Spain) and Poznan (western Poland) . Aerobiologia , 25 : 55 – 63 .
- Arobba , D. , Guido , M. A. , Minale , P. , Montanari , C. , Placereani , S. , Pracilio , S. , Troise , C. , Voltolini , S. and Negrini , A. C. 2000 . Airborne pollen in Genoa (NW-Italy): A comparison between two pollen-sampling stations . Aerobiologia , 16 : 233 – 243 .
- Bergamini , B. M. , Grillenzoni , S. , Andreoni , A. D. , Natali , P. , Ranzi , A. and Bertolani , M. F. 2004 . Alternaria spores at different heights from the ground . Allergy , 59 : 746 – 752 .
- Bryant , R. H. , Emberlin , J. C. and Norris-Hill , J. 1989 . Vertical variation in pollen abundance in north-central London . Aerobiologia , 5 : 123 – 137 .
- Caramiello , R. , Polini , V. , Siniscalco , C. , Mincigrucci , G. , Romano , B. , Frenguelli , G. and Bricchi , E. 1985 . Comparison between airborne pollens in Torino and Perugia (Italy) 1982-83-84 . Aerobiologia , 1 : 39 – 45 .
- Cariñanos , P. , Emberlin , J. , Galán , C. and Domínguez-Vilches , E. 2000 . Comparison of two pollen counting methods of slides from a Hirst type volumetric trap . Aerobiologia , 16 : 339 – 346 .
- Chakraborty , P. , Gupta-Bhattacharya , S. , Chowdhury , I. , Majumdar , M. R. and Chanda , S. 2001 . Differences in concentrations of allergenic pollens and spores at different heights on an agricultural farm in West Bengal, India . Annals of Agricultural and Environmental Medicine , 8 : 123 – 130 .
- Comtois , P. , Alcázar , P. and Néron , D. 1999 . Pollen counts statistics and its relevance to precision . Aerobiologia , 15 : 19 – 28 .
- Corden , J. M. , Stach , A. and Millington , W. M. 2002 . A comparison of Betula pollen seasons at two European sites; Derby, United Kingdom and Poznan, Poland (1995–1999) . Aerobiologia , 18 : 45 – 53 .
- El-Ghazaly , G. , El-Ghazaly , P.-K. , Larsson , K. A. and Nilsson , S. 1993 . Comparison of airborne pollen grains in Huddinge and Stockholm, Sweden . Aerobiologia , 9 : 53 – 67 .
- Ekebom , A. , Nilsson , S. , Saar , M. and Van Hage-Hamsten , M. 1997 . A comparative study of airborne pollen concentrations of three allergenic types in Tartu (Estonia), Roma/Gotland and Stockholm (Sweden) 1990–1996 . Grana , 36 : 366 – 372 .
- Eversmeyer , M. G. and Kramer , C. L. 1987 . Single versus multiple sampler comparisons . Grana , 26 : 109 – 112 .
- Fiorina , A. , Mincarini , M. , Sivori , M. , Scordamaglia , A. , Canonica , G. W. and Brichetto , L. 1999 . Aeropollinic sampling at three different heights by personal volumetric collector (Partrap FA 52) . Allergy , 54 : 1309 – 1315 .
- Frenz , D. A. , Melcher , S. E. , Murray , L. W. and Sand , R. E. 1997 . A comparison of total pollen counts obtained 5.6 km apart . Aerobiologia , 13 : 205 – 208 .
- Galán , C. , Alcázar-Teno , P. , Domínguez-Vilches , E. , Villamandos , F. and Infante , F. 1995a . Airborne pollen grain concentrations at two different heights . Aerobiologia , 11 : 105 – 109 .
- Galán , C. , Emberlin , J. , Domínguez , E. , Bryant , R. H. and Villamandos , F. 1995b . A comparative analysis of daily variations in the Gramineae pollen counts at Córdoba, Spain and London, UK . Grana , 34 : 189 – 198 .
- Giorato , M. , Bordin , A. , Gemignani , C. , Turatello , F. and Marcer , G. 2003 . Airborne pollen in Padua (NE-Italy): A comparison between two pollen samplers . Aerobiologia , 19 : 129 – 131 .
- Gottardini , E. and Cristofolini , F. 1997 . Spring airborne pollen data in two sites in Trentino (northern Italy): A comparison with meteorological data . Aerobiologia , 13 : 199 – 204 .
- Hart , M. L. , Wentworth , J. E. and Bailey , J. P. 1994 . The effects of trap height and weather variables on recorded pollen concentration at Leicester . Grana , 33 : 100 – 103 .
- Hirst , J. M. 1952 . An automatic volumetric spore trap . Annals of Applied Biology , 39 : 257 – 265 .
- Irdi , G. , Jones , J. R. and White , C. M. 2002 . Pollen and fungal spore sampling and analysis. Statistical evaluations . Grana , 41 : 44 – 47 .
- Jäger , J. , Nilsson , S. , Berggren , B. , Pessi , A.-M. , Helander , M. and Ramfjord , H. 1996 . Trends of some airborne tree pollen in the Nordic countries and Austria, 1980–1993. A comparison between Stockholm, Trondheim, Turku and Vienna . Grana , 35 : 171 – 178 .
- Jones , G. D. , Vaughn , M. and Bryant , V. M. Jr. 2007 . A comparison of pollen counts: Light versus scanning electron microscopy . Grana , 46 : 20 – 33 .
- Khattab , A. and Levetin , E. 2008 . Effect of sampling height on the concentration of airborne next term fungal spores . Annals of Allergy, Asthma & Immunology , 101 : 529 – 534 .
- Koch , A. , Heilemann , K.-J. , Bischof , W. , Heinrich , J. and Wichmann , H. E. 2000 . Indoor viable mould spores – a comparison between two cities, Erfurt (eastern Germany) and Hamburg (western Germany) . Allergy , 55 : 176 – 180 .
- Kramer , C. L. and Eversmeyer , M. G. 1984 . Comparisons of airspora concentrations at various sites within a ten kilometer radius of Manhattan, Kansas, USA . Grana , 23 : 117 – 122 .
- Kupias , R. , Helander , M. L. , Saar , M. and Mäkinen , Y. 1989 . Comparison of some pollen concentrations in Finland and the Estonian SSR . Aerobiologia , 5 : 94 – 103 .
- Lejoly-Gabriel , G. and Leuschner , R. M. 1983 . Comparison of air-borne pollen at Louvain-la-Neuve (Belgium) and Basel (Switzerland) during 1979 and 1980 . Grana , 22 : 59 – 64 .
- Leuschner , R. M. 1999 . Comparison between pollen counts at ground and at roof level in Basel (Switzerland) . Aerobiologia , 15 : 143 – 147 .
- Leuschner , R. M. and Boehm , G. 1981 . Pollen and inorganic particles in the air of climatically very different places in Switzerland . Grana , 20 : 161 – 167 .
- Meiffren , I. 1988 . Airborne pollen of Toulouse, southern France. Comparison with Bordeaux and Montpellier . Grana , 27 : 183 – 201 .
- Palmas , F. and Cosentino , S. 1990 . Comparison between fungal air spore concentrations at two different sites in the south of Sardinia . Grana , 29 : 87 – 95 .
- Pedersen , B. V. and Moseholm , L. 1993 . Precision of the daily pollen count: Identifying sources and variation using variance component models . Aerobiologia , 9 : 15 – 26 .
- Piotrowska , K. 2004 . Comparison of Alnus, Corylus, and Betula pollen counts in Loubling (Poland) and Skien (Norway) . Annals of Agricultural and Environmental Medicine , 11 : 205 – 208 .
- Rantio-Lehtimäki , A. , Helander , M. L. and Pessi , A. M. 1991 . Circadian periodicity of airborne pollen and spores; significance of sampling height . Aerobiologia , 7 : 129 – 135 .
- Rizzi , L. , Pizzulin , M. and Larese , F. 1992 . Comparison between the allergenic airborne pollen in Trieste and at Lozzo di Cadore (Italy) in 1989 . Aerobiologia , 8 : 385 – 391 .
- Sterling , M. , Rogers , C. and Levetin , E. 1999 . An evaluation of two methods used for microscopic analysis of airborne fungal spore concentrations from the Burkard Spore Trap . Aerobiologia , 15 : 9 – 18 .
- Strandhede , S.-O. and Wihl , J.-A. 1981 . Comparison of pollen counts in Copenhagen and Malmö . Grana , 20 : 187 – 189 .
- Tormo , R. , Muñoz , A. F. and Silva , I. 1996 . Sampling in aerobiology: Differences between traverses along the length of the slide in Hirst sporetraps . Aerobiologia , 12 : 161 – 166 .
- Trigo , M. M. , Toro , F. J. , Recio , M. and Cabezudo , B. 2000 . A statistical approach to comparing the results from different aerobiological stations . Grana , 39 : 252 – 258 .
- Welam-Henningson , E. and Ahlberg , M. S. 1994 . Evaluation of microbial aerosol samplers: A review . Journal of Aerosol Science , 25 : 1459 – 1492 .