ABSTRACT
The high lustre and desirable aesthetics of stainless steels have resulted in their application for decorative purposes in a variety of industries including homeware and automotive, but primarily in architecture. It is possible to further enhance the aesthetics of stainless steel by imparting a thin coloured film on the surface that does not impair any inherent desirable properties, including corrosion resistance. There are two different types of thin coloured films, iridescent and non-iridescent. Iridescent colour can visually change depending on the angle from which it is viewed and is a consequence of a phenomenon known as thin film interference. Non-iridescent colour is created by the absorbance of light waves of all but a given wavelength, the colour is intrinsic to the thin film and does not change with viewing angle. For the purpose of this review, colouring techniques have categorised into three groups, electrochemical, non-electrochemical and laser colouring. Laser colouring can be utilised as either an electrochemical or non-electrochemical process and is particularly suited to colouring small areas and intricate shapes. Non-electrochemical techniques, such as physical vapour deposition (PVD) and thermal tinting, avoid the use of toxic chemicals, however, they present other challenges, including energy consumption and scrappage rate. The use of non-electrochemical and laser techniques to colour stainless steel can negate the use of toxic hexavalent chromium; however, they are yet to be widely adopted by industry for this purpose. Therefore, it is important to consider each technique to understand why this is the case. The present Part 2 of the review discusses non-electrochemical and laser processes used to produce coloured films on stainless steel, whereas Part 1 discussed the electrochemical processes.
Introduction
Stainless steel has been, and remains, extensively used in civil engineering and architecture, one of the earliest and most famous examples being the uppermost section of the Chrysler Building in New York. Owing to stainless steel’s natural resistance to corrosion and weathering, it has remained visually untarnished for over 90 years.Citation1,Citation2
One approach to enhance the aesthetics of stainless steel is to impart colour upon it. Electrochemical techniques of imparting colour have been around for about 100 years and utilised on structures such as the Millennium Centre, Cardiff, the Ring of Remembrance, Notre Dame de Lorette.Citation3–5 Furthermore, electrochemical techniques have been developed for the inclusion of patterns and different shades of colour on a single piece of stainless steel, as seen on the Neiman Marcus Department Store, Massachusetts.Citation6 Electrochemical processes utilise an electrolyte to either modify the pre-existing chromium oxide thin film on the stainless steel surface or deposit an entirely new thin film on top of the pre-existing oxide. Non-electrochemical processes utilise alternative media to an electrolyte to achieve this, examples include the use of high temperatures in thermal tinting and vaporisation followed by condensation in physical vapour deposition (PVD).Citation7,Citation8
As will be reviewed in the current paper, non-electrochemical and laser processes have also been claimed to produce a range of long-lasting, bright colours that can be either iridescent or non-iridescent in nature. Although the electrochemical, chromic acid-based INCO process was widely adopted by industry following its development in the 1970s,Citation9 non-electrochemical processes may become more appealing following the introduction of the UK-adopted REACh (Registration, Evaluation, Authorisation and Restriction of Chemicals) regulations, which restrict the use of hexavalent chromium.Citation10,Citation11
Electrochemical processes were examined in Part 1 of this review recently in this journal (G. T. Alliott et al. Trans. IMF, 2022, 100(3), 128–137). Part 2 now reviews the non-electrochemical and laser processes by which it is possible to impart a thin coloured film on stainless steel.
Physical vapour deposition
Physical vapour deposition (PVD) is a general term used to describe a family of atomistic deposition processes by which thin films are deposited via condensation of a vaporised solid or liquid in a vacuum or low pressure environment.Citation8 The colour produced as a result of PVD will change depending on which compound is used to form the film, with the exact quantities of each element in the compound having an influence. The thickness of the film is also a determining factor. Titanium aluminium nitride (TiAlN), for example, will produce colours from gold to dark blue.Citation8,Citation12,Citation13 It is possible for the colour of bulk materials to appear different from the deposited layer due to the layer’s colour being determined by both the chemical composition and crystal structure. An example of gold stainless steel coloured by PVD in use at Vulcania amusement park, France, is shown in .Citation14
Figure 1. Installation at Vulcania amusement park, France, clad in gold stainless steel coloured using PVD.Citation14
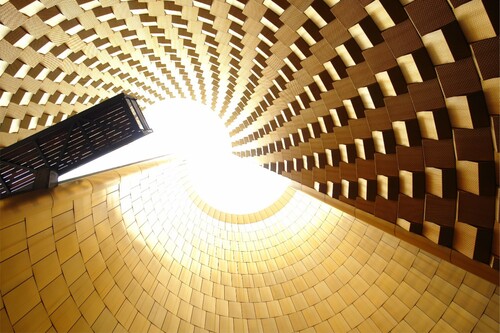
In PVD coatings, the structure can be very different to the bulk material. The substrate material can also influence the structure of the deposited film. According to KosmačCitation12 it is possible to achieve a full spectrum of colours using PVD. Kosmač also suggested that surface colour will not change with viewing angle, whereas Reiners et al.Citation13 disagreed and suggested that semi-transparent PVD coatings on a highly reflecting substrate produce colours which do differ and this is corroborated by Panjan et al.Citation15
The PVD process can be used on complex geometries as well as large sheets.Citation12 The high degree of film adhesion to the substrates allows sheet products to be shaped after they have been coloured, Hultin et al.Citation16 reported that sheets coloured using their process can be bent at angles greater than 90° without any colour change or film delamination. However, PVD films do suffer from defects meaning corrosive media could contact the substrate. This could be problematic, even for stainless steel, depending on the size of the defect and the chemistry of the environment.
KosmačCitation12 quotes PVD coating thickness as approximately 0.3 µm, whereas Reiners et al.Citation13 provide a range of 1-5 µm. Panjan et al.Citation14 provide an example of a nano-layered AlTiN/TiN coating with a total thickness of approximately 0.3 µm, similar to the value provided by Kosmač.Citation12 Examples of the colours produced by Panjan et al. in stainless steel are shown in .Citation15 In their patent for depositing a PVD coating on stainless steel strip, Hultin et al.Citation16 state a preferable coating thickness of 2-3 µm. Therefore, there appears to be some disagreement amongst authors as to the degree of film thickness that is required for coloured PVD films. However, this could be a result of differing coating chemistries and crystal structures resulting in varying film thickness requirements. The authors also appear to disagree on the type of colour produced, Reiners et al.Citation13 and Panjan et al.Citation5 both claim to produce interference colours, despite the significant difference in film thickness, whereas KosmačCitation12 states that the colour is not dependant on viewing angle, despite quoting a similar film thickness to Reiners et al.Citation13 It is therefore unclear as to whether or not it is possible to produce both interference and intrinsic colour using PVD.
Figure 2. Stainless steel samples coated, by Panjan et al.,Citation15 with different thickness of AlTiN using PVD.
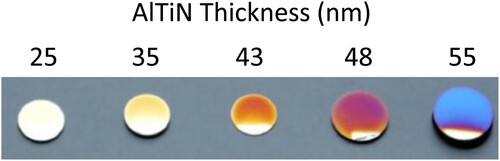
To colour large products, a vacuum chamber several square metres in size may be required.Citation17 A larger vacuum chamber would increase time and energy to vacuum and vent and require large amounts of energy to power the coating process itself. The possibility for a high scrappage rate has also been reported, owing to the inability to rework any products where the finish is imperfect.Citation17
To negate possible issues with coating large sheets, Hultin et al.Citation16 have developed a continuous roll to roll PVD process for colouring stainless steel. The process can colour stainless steel coils preferably between 100 m and 20,000 m long at a minimum coating speed of 3 m min−1, using electron beam evaporation or sputtering on the target material. Hultin et al.Citation16 state that a large variety of metal oxides can be deposited, however, preference is for the use of titanium dioxide (TiO2), alumina (Al2O3) or chromia (Cr2O3), from which a large range of colours can be achieved. The coatings produced using the process are said to be hard wearing and corrosion resistant and suitable for sports, marine applications, household appliances and fashion.
Thermal tinting
It has been reported that when stainless steel is exposed to high temperatures, such as the heat of a furnace or welding equipment, colour appears on the surface of the steel.Citation7,Citation18–22 The colour is formed by the progressive thickening of the surface oxide layer. The thickening of the oxide layer by exposure to heat to produce colour is called thermal tinting or temper colouring.Citation7,Citation23 The colour produced is a result of thin film interference. ,Citation24 shows a thermal tinted stainless steel motorbike exhaust manifold following exposure to hot exhaust gases.
Figure 3. Motorcycle exhaust manifold appears coloured resulting from a thermally grown interference film after exposure to hot exhaust gasses.Citation24
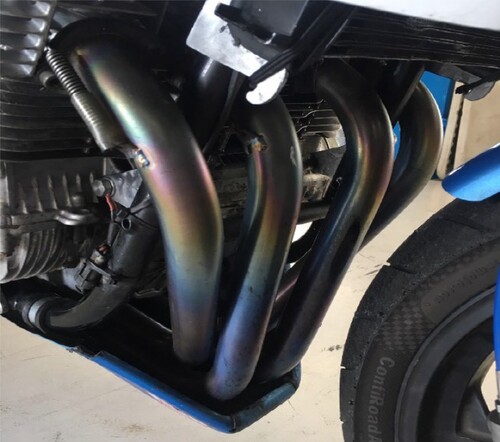
Labanowski and Glowacka,Citation20 who observed thermal tinting on stainless steel welds, postulate that the properties of the oxide are determined by five factors:
Time and temperature of heat exposure
Composition of the atmosphere during the heating and cooling process
Chemical composition of the basic alloy
Surface physical condition
Binding of the oxide to the native metal
The colour produced depends on several factors; temperature (usually between 300 and 850°CCitation7), time (at maximum temperature as well as heating and cooling rates), atmosphere (in ambient conditions or controlled atmosphere), surface roughness and chromium content.Citation7,Citation18–22 Higher temperatures and longer exposure times will lead to a thicker oxide film being produced. As the thickness of the film increases, light interference will cause the film to appear different colours. The thickest films have been reported as blues and purples, whereas thinner films are yellow, gold, bronze and copper; other colours reported include grey, black, brown and red.Citation7,Citation21 Takahashi et al.Citation19 have reported issues with colouring above 450°C, citing that chromium-carbides precipitate in stainless steel between 450°C and 750°C. Precipitation of these carbides could lead to the stainless steel being susceptible to pitting corrosion, they therefore recommend processing temperatures are kept below 450°C.
The most sizeable influence on colour is reported by the British Stainless Steel Association (BSSA) as the chromium content of the steel; the higher the chromium content the higher is the heat resistance.Citation23 Higher temperatures and longer heat exposure times are therefore needed to colour the stainless steel. However, reducing the chromium content to speed up colouring times and reduce colouring temperatures will sacrifice the corrosion resistance of the stainless steel. Surface finish also helps to determine the rate of oxidation.Citation18,Citation22 Rougher surfaces can oxidise at a higher rate and also affect viewing angle, thus altering the perceived colour.Citation23 The difference in perceived colour as a consequence of surface roughness is caused by the light waves reflecting at different angles compared to a smooth surface. Takahashi et al.Citation19 identified the variation between colours produced from unpolished and electropolished stainless steel. On unpolished stainless steel, gold and blue were produced at 400 and 800°C respectively, whereas on electropolished stainless steel gold and light blue were produced at 600 and 800°C, respectively. The causes of the differences in colour were reported as variations in the surface chemistry caused by the electropolishing. Localised changes in colour across the surface were also reported.Citation7,Citation19 Providing a solution for this inconsistent colouration was the focus of a patent by Takahashi et al.Citation19 Microparticles of titanium dioxide (TiO2) and silica (SiO2) were added to water to create a slip (a solution of water containing the microparticles in suspension) which was added to the surface of the stainless steel prior to heat treatment. The thermally grown oxide is then said to grow uniformly beneath the slip, before the latter is washed off at the end of the process.
PreddyCitation22 has patented a method of producing various colours on stainless steel utilising the effect that surface roughness has during thermal tinting. In the process the use of various grades of silicon carbide paper to impart differing surface conditions is described, yielding different colours when exposed to high temperatures. The lowest grit number, 36 grit, produced blue and purple colours when used to abrade the surface of the stainless steel prior to heat treatment for 30 min at 500 °C. The higher grit numbers of 80 and 120 produced gold and bronze respectively. Therefore, samples abraded with the coarsest surfaces must yield the thickest oxide films. Observations by Takahashi et al.Citation19 however, suggest the thermal tinted samples, polished using mechanical methods as opposed to electropolishing, have a lower quality surface finish and lustre.
Higginson et al.Citation7 carried out analysis of thermally grown, interference oxides using X-ray photoelectron spectroscopy (XPS); the depth profile from one such sample is shown in .Citation7 It was found that the oxides consisted of two layers, the outer layer being formed predominantly of iron oxide of the form Fe2O3 (haematite), covered with small oxide nodules also formed primarily of haematite. Oxide nodules are not a feature which has been mentioned by other authors. The inner layer was found to be predominantly a spinel oxide of the form AB2O4, where AB was chromium and iron. Similar structures have also been proposed by other authors.Citation19–21 Labanowski and GlowackaCitation20 suggest the dual layer structure is a result of chromium’s tendency to preferentially oxidise over iron. Several authors have reported that the tendency of chromium to oxidise leaves the surface of the metallic substrate lacking chromium and thus its corrosion resistance compromised.Citation19–21 Coupled with the cracked and brittle oxide described by Labanowski and GlowackaCitation20 and Brajkovic et al.Citation21 thermally coloured stainless steel could be susceptible to localised attack by corrosive media in the cracks in the form of pitting corrosion. Both Labanowski and GlowackaCitation20 and Brajkovic et al.Citation21 observed thermally grown oxides on welds in stainless steel and both expressed similar concerns on the vulnerability of the coloured areas to corrosion. Both publications propose methods of chemically removing the interference oxide to restore the corrosion resistance of the stainless steel substrate.
Figure 4. XPS depth profile, produced by Higginson et al.,Citation7 of a Type 304 stainless steel sample following oxidation at 650°C for 8 minutes.
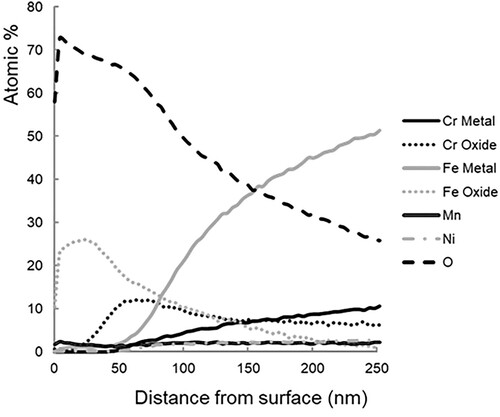
Further concerns include the reproducibility of the colour. Different heating environments (chemical species present in air, humidity etc.) will result in colours being produced at different times and temperatures.
Heat tinting avoids the use of potentially environmentally problematic chemicals, however, there are other issues related to the process. Due to the high temperatures and large furnace which may be required, depending on product size, the process could be energy intensive.Citation23 Additionally, a large range of colouring times have also been suggested. Higginson et al.Citation7 report little increase in film thickness upwards of 7 min for samples coloured at 650°C, whereas Takahashi et al.Citation19 report times of 30 min across a range of temperatures used to produce different colours. This time is also reported by Preddy,Citation20 however, if the cooling time is also considered, a single colouring cycle takes up to 53 min. Production efficiency could be maximised by a continuous process, such as the one patented by Underwood,Citation18 where a metal sheet is passed across a heating element at a predetermined speed which raises the temperature of the metal surface to the desired level. The stainless steel is continually exposed to a cooling atmosphere of the desired composition to impart a uniform, preselected, stable interference colour.
Although other issues such as processing times and non-uniform colouring have been proposed, it does appear that solutions do exist. Proposed uses for thermally tinted stainless steel include ornamental construction, counter tops, baths and sinks.Citation19,Citation22 All these uses would be subjected to wear, moisture and other corrosive media, therefore, although concerns have been expressed with regards to the corrosion resistance of thermally grown oxides, it has not deterred authors from recommending them for use.
Sol gel coatings
Sol–gel is a chemical synthesis method of producing metastable solids from molecular precursors in a liquid medium via hydrolysis and condensation reactions.Citation25,Citation26 A sol is a liquid containing colloidal particles in suspension. A gel consists of a continuous liquid phase supported by a porous, solid network that is usually the consequence of covalent bonds forming between sol particles.Citation26
One application of the sol–gel process is to deposit ceramic or ceramic hybrid coatings onto the surface of metals to protect the substrate against forms of corrosion. Hybrid sol–gel coatings consist of an organic and an inorganic component whereas ceramic sol–gel coatings consist of only an inorganic component. Research has been conducted in the past on how to produce coloured sol–gel coatings and some processes have even been patented.Citation27–30
Sol–gel coatings are versatile due to the variety of film compositions and the control over the structure by changing process parameters and the incorporation of nano-particles. The most frequently used coating compositions are based on silica (SiO2), alumina (Al2O3) and zirconia (ZrO2).Citation30 Parameters such as ageing time and baking temperature can be altered to influence film properties such as porosity, ductility and hardness.Citation25,Citation32,Citation33
Although there are several advantages to using the sol–gel process over other colouring alternatives, there are still some drawbacks that need to be considered when deciding upon a coating process to use. The most frequently discussed issue is the shrinkage of the coatings during the baking phase. Internal tensile stresses resulting from the loss of moisture, coupled with a ceramic’s poor performance in tension, result in the fracture of the film. Cracking can also occur during service due to differences in thermal expansion coefficient between the coating and substrate. Cracking can result in impaired aesthetics and localised aggressive corrosion of the substrate if corrosive media penetrates the fractures. Other drawbacks include the cost of precursors, difficulty in avoiding residual porosity and preferential precipitation of a particular oxide during sol formation due to different reactivities of precursors.Citation33
Sol gel coatings could be used to provide protection to a coloured oxide already applied to a stainless steel surface, such as in the process described by Piesslinger-Schriber,Citation34 or inorganic pigment particles can be added to the coating to provide non-iridescent colour. Two patents, by Boehome and Piesslinger-SchweigerCitation30 and Piero,Citation29 have been identified that describe processes which apply colour to metallic surfaces by the inclusion of nano-sized inorganic pigments particles in the coating.Citation29,Citation30 The colour selection is described by Boehme and Piesslinger-SweigerCitation30 as ‘freely selectable’, however, the colour would be non-iridescent and not produced via thin film interference, unlike the electrochemical processes previously discussed in Part 1 of this review. If the sol–gel coating produces the colour and is not used to protect a coloured oxide it can be in direct contact with the stainless steel substrate. It is known that sol–gel coatings in direct contact with some metallic substrates form strong Van der Waals forces and during curing can form stable covalent bonds between the coating and substrate.Citation35
PieroCitation29 describes a process that can be used with ‘all the ceramic oxides’ to produce a sol–gel coating, however, it is stated that the best results are achieved using alumina. Boehme and Piesslinger-Schweiger,Citation30 however, opted for a silica based sol–gel. Both PieroCitation29 and Boehme and Piesslinger-SchweigerCitation30 suggest a spray coating method, however, the latter state application by spreading or rolling is also possible. Spraying is known to produce thinner coatings with higher accuracy than the most commonly used sol–gel application process of dipping.Citation36 Following application, PieroCitation29 suggests 1 h at 140–150°C is sufficient to fully cure the coating, whereas Boehme and Piesslinger-SchweigerCitation30 suggest a higher curing temperature range of preferably between 200–250 °C, however, for a lower optimum time of 30 min. Both patents claim their respective processes result in films which are UV and corrosion resistant, have good adherence to the substrate and produce aesthetically pleasing colours.Citation19,Citation30 PieroCitation29 quotes a coating thickness of 50–70 µm, however, it would appear this is prior to curing, where densification and thinning of the sol–gel will occur, a porosity of 5–10% is also given. Boehme and Piesslinger-SchweigerCitation30 quote a post curing thickness range of 0.5–5 µm.
Corrosion inhibitors can be added to protective sol–gel films to produce a self-healing effect similar to that of the native oxide on stainless steel, however, if the sol–gel coating also contains pigment particles it is not known whether the addition of corrosion inhibitors as well would impair the integrity of the film.Citation25,Citation31,Citation32,Citation35 Zheludkevich et al.Citation35 reported that the critical concentration of cerium oxide inhibitors is in the range of 0.2–0.6 wt%, concentrations above this could lead to the formation of defects in the coating. A critical wt % for inorganic pigments in the coating was not reported in either of the patents, so remains an unknown. As an alternative to adding inorganic particles, organic inhibitors can be incorporated into the matrix. Localised changes in pH, resulting from corrosion, would trigger the release of the organic molecular inhibitor. The incorporation of an organic species creates a hybrid coating. Ideally it would be possible to add corrosion inhibitors to coatings both providing colour or protecting a coloured film to produce the self-healing effect. Cerium salts have been reported as the most effective inhibitor to include in the sol–gel matrix.Citation25,Citation32
Laser colouring of stainless steel
Maeda et al.Citation37 were first granted a patent for improving the surface functions of steel alloys using a laser in 1987. The patented process included the colouring of stainless steel, the same process would provide good wear resistance, film adhesion to the substrate and corrosion resistance. In the process steel alloys were placed into an oxidising solution of acid or metal salt and a high powered, CO2 laser shone through the solution to be focussed on the steel alloy surface. Maeda et al.Citation37 state that the solution must contain at least one of the following, nitric acid or a nitrate, chromic acid or a chromate, permanganic acid or a permanganate. The laser was used as a localised heating source. It is said that the laser would ‘instantaneously’ break down the native oxide on the surface of the steel and a new film would be formed, by the steel reacting with the electrolyte, in 0.1 s or less.Citation37 It was suggested that the different interference colours are achieved by a variation in chromium concentration in the surface layers of the steel alloy. If interference colours are achieved using this process, the lack of any mention in the patent of thin film interference and the influence of film thickness on colour is surprising.
Common traits across published processes in the literature for the use of a laser to colour stainless steel are that the laser is used to heat the surface of the stainless steel and colours are produced by way of an interference oxide.Citation37–44 The exception is Ahsan et al.,Citation45 who utilise a titanium-sapphire, femtosecond laser, to produce micro-holes and micro/nano-gratings on the surface of the stainless steel. The colour is produced by the holes or gratings trapping the incident light, and light waves of a particular wavelength within the visible light spectrum are released via Fresnel reflections. Photographs of treated surfaces, shown in ,Citation45 demonstrate that poor quality colours were produced using this process.Citation45
Figure 5. Micro/nano-gratings induced colourised stainless steel surfaces produced by Ahsan et al.Citation45

Authors utilising lasers as a heating source have been seen to utilise oxidising solutionsCitation37,Citation42 or gaseous environments Citation38–41,Citation43,Citation44 to contribute to the formation of an interference film. As well as Maeda et al.,Citation37 Shin et al.Citation42 describe immersing the stainless steel in an oxidising solution, however, their processes differ. Shin et al.Citation42 produced a laser mask on the surface of the stainless steel before immersing it in a 2 M sodium nitrate solution. The area of the surface which interacts with the laser will temporarily not react with the oxidising solution, allowing an interference film to be produced on unaffected areas. Longer exposure times to the laser will produce a longer lasting barrier layer, therefore multi-coloured designs can be precisely produced on the surface of the stainless steel. Several authors have described methods of producing interference films in different gaseous environments.Citation38–41,Citation43,Citation44 Li et al.,Citation41 Chang et al.Citation43 and Veiko et al.Citation44 all colour in air whereas Zheng et al.Citation40 use a pure O2 or N2 atmosphere and Sugioka et al.Citation38,Citation39 use silane (SiH4) gas. Heating the stainless steel using a laser in silane allows Si to be implanted into the surface layers of the metal substrate by reacting with the iron to form iron disilicide (FeSi2). Using this process, they describe achieving gold, red and blue colours.
Zheng et al.Citation40 describe film formation in their process as taking place in four stages:
Transport of oxygen from the surrounding atmosphere to the heated metal surface.
Adsorption of the oxygen.
A transfer of electrons from the metal substrate to the adsorbed oxygen molecules.
Diffusion of species through the oxide layer.
Zheng et al.Citation40 report that film growth continues by electron tunnelling and diffusion of species through the growing interference oxide until such time that the film is too thick to allow diffusion to continue, at which point the growth is self-terminating. Whilst briefly reviewing other processes, Zheng et al.Citation40 state that printed or emulsion colours on metallic substrates have issues with scratch and wear resistance and electrochemical processes have limited flexibility in producing colours and patterns, problems which are apparently negated by the use of laser processing. Whilst discussing their laser process, Maeda et al.Citation37 reported good wear resistance of the interference film. Zheng et al.,Citation40 however, describe electrochemically grown colours as being varied by changing the metal ions in the electrolyte. This is unlike other electrochemical processes, which are known to produce a range of aesthetically pleasing colours, which are typically dictated largely by film thickness and thus processing time.Citation9,Citation46–54 Zheng et al.Citation40 on the other hand only report brown, yellow, green and blue colours being produced.
Variations on the type of laser used exist between authors. Earlier investigations by Sugioka et al.Citation38,Citation39 and Maeda et al.Citation37 use krypton fluoride (KrF) and high-powered CO2 lasers respectively. KrF lasers are a type of excimer laser which are made up of a noble gas and a halogen, in this case krypton and fluorine.Citation55 More recent studies by Shin et al.,Citation42 Chang et al.Citation43 and Veiko et al.Citation44 have utilised ytterbium fibre lasers, a type of solid state laser. Solid state lasers are known to exhibit high beam quality and require little maintenance.Citation56
Lasers are a method of producing interference films, with promising mechanical properties, on metallic substrates, however, the size of their focal point on the stainless steel means that colouring, although precise, is very localised.Citation37,Citation41–45
Conclusions
For PVD colouring processes the colour is typically determined by the metal compound depositedCitation8,Citation12,Citation13 however, authors disagree as to whether the colour is intrinsic to the compound and particular film structure or whether the colour is created by thin film interference.Citation12,Citation13,Citation15 Films have been reported as yielding extremely good mechanical properties and desirable and reproducible colour. However, concerns remain with regards to energy consumption for chambers coating large parts and the potential scrappage rate of the process.Citation17
For thermal tinting the range of colours achieved by authors is comparable to that of chromic-sulphuric acid based electrochemical processes.Citation7,Citation21 Higginson et al.Citation7 found the oxide to consist of a two layer structure, the outer being predominantly iron oxide and the inner a spinel oxide of iron and chromium.
Laser colouring processes primarily utilise the laser to raise the surface temperature of stainless steel in localised areas whilst in a controlled environment resulting in the growth of an oxide film. Several bases for a colouring solution were suggested by Maeda et al.Citation37 Earlier processes utilised KrF or CO2 lasers,Citation37–39 however, ytterbium fibre lasers were later used.Citation42–44 Laser colouring is well suited to producing small intricate designs due to the low focal area of lasers but would not be able to match other processes when colouring large articles.
Sol–gel is a method of producing hard ceramic and hybrid coatings on a variety of substrates including stainless steels. Sol–gel can be conducted at ambient or relatively low temperatures, however, heat is often applied to reduce the curing time of the coating. They are typically silica, zirconia or alumina based depending on desired properties and offer good wear, scratch and corrosion resistance. Nanoparticles can be contained within the sol–gel matrix to produce self-healing qualities or impart intrinsic colour by the addition of inorganic dye particles. Patented processes have been developed using sol–gel coatings to protect interference films or impart colour through the inclusion of dye particles, however, there is a relatively small amount of literature that discusses sol-gels as colouring agents.Citation29,Citation30,Citation34
Considering the pertinent literature discussed in Part 2 of this review, laser and non-electrochemical techniques have yielded promising results. However, there is no clear technique that matches or exceeds each of the desirable properties of hexavalent chromium processes without posing new concerns, such as scrappage rate, energy consumption, colouring time and cost of equipment.
Part 1 reviews the electrochemical processes for producing coloured films on stainless steel.
Disclosure statement
No potential conflict of interest was reported by the author(s).
References
- A. Harrison: ‘Understanding stainless steel’; 2013, Sheffield, British Stainless Steel Association.
- E. P. Nash: ‘Manhattan Skyscrapers’, 2nd edn; 2005, New York, Princeton Architectural Press. DOI:10.1007/1-56898-652-1
- H. Jermain. ‘Process of coloring iron or steel articles’, US 1342910, United States Patent Office, 1920.
- J. Glancey. ‘The ring of remembrance, Notre Dame de Lorette’. http://www.telegraph.co.uk/culture/art/architecture/11220393/The-Ring-of-Remembrance-Notre-Dame-de-Lorette.html (accessed Nov 8, 2016).
- Wales Millenium Centre. https://www.wmc.org.uk/en/ (accessed Mar 17, 2019).
- Rimex Metals Ltd. Projects. https://www.rimexmetals.com/ (accessed Jun 3, 2020).
- R. L. Higginson, C. P. Jackson, E. L. Murrell, P. A. Z. Exworthy, R. J. Mortimer, D. R. Worrall and G. D. Wilcox: ‘Effect of thermally grown oxides on colour development of stainless steel’, Mater. High Temp., 2015, 32(1–2), 113–117. DOI:10.1179/0960340914Z.00000000083.
- D. M. Mattox: ‘Handbook of physical vapor deposition (PVD) processing’, 2nd edn; 2010, Oxford, Elsevier. DOI:10.1016/B978-0-8155-2037-5.00025-3.
- T. E. Evans, A. C. Hart, H. James and V. A. Smith: ‘A new process for colouring stainless steel’, Trans. IMF, 1972, 50(1), 77–79. DOI:10.1080/00202967.1972.11870224.
- European Union. ‘Regulation (EC) No 1907/2006 of the European Parliament and of the Council of 18 December 2006 Concerning the Registration, Evaluation, Authorisation and Restriction of Chemicals (REACH), Establishing a European Chemicals Agency, Amending Directive 1999/4’; 2006, Brussels, European Union. DOI:2004R0726-v.7 of 05.06.2013.
- UK REACH explained. https://www.hse.gov.uk/reach/about.htm (accessed Aug 21, 2022).
- A. Kosmač: ‘Colouring stainless steel’, Mater. Appl. Ser., 2011, 16, 1–22.
- G. Reiners, U. Beck and H. A. Jehn: ‘Decorative optical coatings’, Thin Solid Films, 1994, 253(1–2), 33–40. DOI:10.1016/0040-6090(94)90290-9.
- G. Churchard. ‘Vulcania’. https://www.flickr.com/photos/graeme/2575704098 (accessed Apr 11, 2021).
- M. Panjan, M. Klanjšek Gunde, P. Panjan and M. Čekada: ‘Designing the color of AlTiN Hard coating through interference effect’, Surf. Coatings Technol., 2014, 254, 65–72. DOI:10.1016/j.surfcoat.2014.05.065.
- A. S. Hultin, M. Schuisky, U. Isaksson and M. Ahlgren. ‘Stainless steel strip coated with a decorative layer’, US 2007/0275264, United States Patent Office, 2007. DOI:10.1037/t24245-000.
- ‘Rimex Metals Ltd.: Analysis of alternatives’; 2015, London, Rimex Metals Ltd.
- L. D. Underwood. ‘Method of coloring metals by the application of heat’, US 3664884, United States Patent Office, 1972.
- H. Takahashi, S. Goto, S. Takata, M. Shibata and T. Hata. ‘Method for treating the surface of stainless steel by high temperature oxidation’, US 4776897, United States Patent Office, 1988.
- J. Łabanowski and M. Głowacka: ‘Heat tint colours on stainless steel and welded joints’, Weld. Int., 2011, 25(7), 509–512. DOI:10.1080/09507116.2010.540837.
- T. Brajkovic, I. Juraga and V. Simunovic: ‘Influence of surface treatment on corrosion resistance of Cr-Ni steel’, Eng. Rev., 2013, 33(2), 129–134.
- R. L. Preddy. ‘Method for coloring stainless steel’, US 2019/0017130, United States Patent Office, 2019.
- British Stainless Steel Association. ‘Heat tint (temper) colours on stainless steel surfaces heated in air’. http://www.bssa.org.uk/topics.php?article=140 (accessed Mar 29, 2020).
- G. Alliott: ‘The electrochemical colouring of austenitic stainless steel in sodium molybdate and other environmenteally benign solutions’, Doctoral Thesis, Loughborough University, 2020.
- R. Figueira, I. Fontinha, C. Silva and E. Pereira: ‘Hybrid sol-gel coatings: smart and green materials for corrosion mitigation’, Coatings, 2016, 6(1), 12. DOI:10.3390/coatings6010012.
- ‘The sol-gel handbook’, (eds. D. Levy and M. Zayat); 2015, Weinheim, Wiley-VCH Verlag GmbH & Co. DOI:10.1002/9783527670819
- T. Y. Lim, Y. M. Park, J. H. Hwang, C. Y. Kim and C. Y. Kim: ‘Properties of transparent color coating glass fabricated by sol-gel technology’, Mater. Sci. Forum, 2007, 544–545, 817–820. DOI:10.4028/www.scientific.net/MSF.544-545.817.
- F. Orgaz and H. Rawson: Coloured Coatings Prepared by the Sol-Gel Process. J. Non. Cryst. Solids, 1986, 82(1–3), 378–390. doi:10.1016/0022-3093(86)90155-9.
- R. Piero. ‘Procedure for the surface colouring of objects, particularly on stainless steel and on metallic materials in general’, 01247114, The Ministry of the Industry of Commerce and Craftsmanship Central Office, Italy, 1994.
- O. Boehme and S. Pießlinger-schweiger. ‘Method for the production of colored stainless steel surfaces. 2016/0310984’, United States Patent Office, 2016.
- M. Guglielmi: ‘Sol-gel coatings on metals’, J. Sol-Gel Sci. Technol., 1997, 8(1–3), 443–449. DOI:10.1023/A:1018373404815.
- D. Wang and G. P. Bierwagen: ‘Sol–gel coatings on metals for corrosion protection’, Prog. Org. Coatings, 2009, 64(4), 327–338. DOI:10.1016/j.porgcoat.2008.08.010.
- A. C. Marques. ‘Sol-gel process: an overview’, https://www.lehigh.edu/imi/teched/LecBasic/Marques_Sol_gel.pdf (accessed Mar 31, 2020).
- S. Piesslinger-Schweiger. ‘Coloured stainless steel surface and method for colouring stainless steel. EP2145980A1’, European Patent Office, 2010.
- M. L. Zheludkevich, I. M. Salvado and M. G. S. Ferreira: ‘Sol–gel coatings for corrosion protection of metals’, J. Mater. Chem., 2005, 15(48), 5099. DOI:10.1039/b419153f.
- R. B. R. Garcia, F. S. Silva and E. Y. Kawachi. ‘Evaluation of dip and spray coating techniques in corrosion inhibition of AA2024 alloy using a silicon/zirconium sol-gel film as coating’, In AIP Conference Proceedings; 2017; 1809, 020017. DOI:10.1063/1.4975432.
- S. Maeda, M. Yamamoto, H. Omata and H. Okada. ‘Method of improving functions of surface of alloy steel by means of irradiation of laser beam, and alloy steel and structure made by the method’, US 4692191, United States Patent Office, 1987.
- K. Sugioka, H. Tashiro, K. Toyoda, E. Tamura and K. Nagasaka: ‘Surface hardening of SUS 304 by irradiation with a KrF Excimer laser in SiH4 gas ambient’, J. Mater. Res, 1990, 5(2), 265–270. DOI:10.1557/JMR.1990.0265.
- K. Sugioka, H. Tashiro, H. Murakami, H. Takai and K. Toyoda: ‘Control of surface color of stainless steel 304 by KrF Excimer laser implant deposition’, Jpn. J. Appl. Phys., 1990, 29(7), L1185–L1187. DOI:10.1143/JJAP.29.L1185.
- H. Y. Zheng, G. C. Lim, X. C. Wang, J. L. Tan and J. Hilfiker: ‘Process study for laser-induced surface coloration’, J. Laser Appl., 2002, 14(4), 215–220. DOI:10.2351/1.1514222.
- Z. L. Li, H. Y. Zheng, K. M. The, Y. C. Liu, G. C. Lim, H. L. Seng and N. L. Yakovlev: ‘Analysis of oxide formation induced by UV laser coloration of stainless steel’, Appl. Surf. Sci., 2009, 256(5), 1582–1588. DOI:10.1016/j.apsusc.2009.09.025.
- H. S. Shin, D. K. Chung, M. S. Park, B. H. Kim and C. N. Chu: ‘Electrochemical etching of stainless steel through laser masking’, J. Micromechanics Microengineering, 2010, 20(5), 055030. DOI:10.1088/0960-1317/20/5/055030.
- C. L. Chang, J. W. Wu and C. Y. Lee: ‘Coloration of stainless steel utilizing fiber laser oxidation’, Appl. Mech. Mater., 2011, 121–126, 70–74. DOI:10.4028/www.scientific.net/AMM.121-126.70.
- V. Veiko, G. Odintsova, E. Ageev, Y. Karlagina, A. Loginov, A. Skuratova and E. Gorbunova: ‘Controlled oxide films formation by nanosecond laser pulses for color marking’, Opt. Express, 2014, 22(20), 24342. DOI:10.1364/OE.22.024342.
- M. S. Ahsan, F. Ahmed, Y. G. Kim, M. S. Lee and M. B. G. Jun: ‘Colorizing stainless steel surface by femtosecond laser induced micro/nano-structures’, Appl. Surf. Sci., 2011, 257(17), 7771–7777. DOI:10.1016/j.apsusc.2011.04.027.
- H. James, C. G. Smith and L. G. Tottle. ‘Colouring stainless steel’, 1122172, The United Kingdom Patent Office, 1968.
- K. Ogura, K. Sakurai and S. Uehara: ‘Room temperature-coloration of stainless steel by alternating potential pulse method’, J. Electrochem. Soc., 1994, 141(3), 648–651. DOI:10.1149/1.2054785.
- C. J. Lin and J. G. Duh: ‘The predominant operation parameters and alternative controllability in the square-wave current pulse process for coloring SUS 304 stainless steel’, Surf. Coatings Technol., 1994, 70(1), 79–85. DOI:10.1016/0257-8972(94)90078-7.
- J. H. Wang and J. G. Duh: ‘Colour tone and chromaticity in a coloured film on stainless steel by alternating current electrolysis method’, Surf. Coatings Technol., 1995, 73(1–2), 46–51. DOI:10.1016/0257-8972(94)02359-X.
- R. Conrrado, N. Bocchi, R. C. Rocha-Filho and S. R. Biaggio: ‘Corrosion resistance of colored films grown on stainless steel by the alternating potential pulse method’, Electrochim. Acta, 2003, 48(17), 2417–2424. DOI:10.1016/S0013-4686(03)00264-0.
- E. Kikuti, R. Conrrado, N. Bocchi, S. R. Biaggio and R. C. Rocha-Filho: ‘Chemical and electrochemical coloration of stainless steel and pitting corrosion resistance studies’, J. Braz. Chem. Soc., 2004, 15(4), 472–480. DOI:10.1590/S0103-50532004000400005.
- L. D. Vazquez-Santoyo, J. J. Perez-Bueno, A. Manzano-Ramirez, J. Gonzalez-Hernandez, J. F. Perez-Robles and Y. V. Vorobiev: ‘Origin of interference colors on austenitic stainless steel’, Inorg. Mater., 2005, 41(9), 955–960. DOI:10.1007/s10789-005-0245-3.
- R. M. R. Junqueira, M. S. Andrade, C. R. O. Loureiro and V. T. L. Buono: Mechanical Properties of Interference Thin Films on Colored Stainless Steel Evaluated by Depth-Sensing Nanoindentation. Surf. Coatings Technol, 2006, 201(6), 2431–2437. doi:10.1016/j.surfcoat.2006.04.023.
- J. Corredor, C. P. Bergmann, M. Pereira and L. F. P. Dick: ‘Coloring ferritic stainless steel by an electrochemical–photochemical process under visible light illumination’, Surf. Coatings Technol., 2014, 245, 125–132. DOI:10.1016/j.surfcoat.2014.02.051.
- H. Abramczyk: ‘Introduction to laser spectroscopy’; 2005, Amsterdam, Elsevier. DOI:10.1016/B978-044451662-6/50005-8.
- R. Paetzel. ‘Comparison excimer laser – solid state laser’, In International Congress on Applications of Lasers & Electro-Optics; Laser Institute of America, 2002; 163015. DOI:10.2351/1.5066151.