ABSTRACT
Sustainability is at the forefront of all research carried out at University of Leicester. There are three main groups contributing to the surface finishing effort: the Centre for Sustainable Materials Processing, the Mechanics of Materials research group and Space Park Leicester; however many collaborations occur across the institution and with industrial partners, including the Materials Innovation Centre with TWI Ltd. The ongoing projects vary across a range of themes, from forensic science to batteries and energy storage. This work aims to give a brief overview of the research groups, centres and institutes, and highlights some relevant projects that fall within the scope of Transactions of the IMF.
1. Introduction
At the University of Leicester, three research groups cover topics under the umbrella of ‘materials finishing’, spanning four Schools within the College of Science and Engineering. These are the Centre for Sustainable Materials Processing (CSMP), the Mechanics of Materials (MoM) group and Space Park Leicester (SPL) ().
Table 1. Authors’ research interests.
The Centre for Sustainable Materials Processing (CSMP) is based in the School of Chemistry and, at the time of writing, has 4 full-time academic staff, 9 postdoctoral researchers and 10 postgraduate researchers, as well as undergraduate students. There are currently 7 active themes ongoing in the group, with at least 3 new funded projects to start in the next 12 months. These projects vary in theme all the way from fingerprint development for forensic science applications, through metal recovery and recycling methods, to alternative battery and energy storage development. Sustainability is at the heart of our research focus and this has led us to develop sustainable and ‘green’ electrolytes (namely Deep Eutectic Solvents (DES), and alternative ionic liquids) for metal finishing applications, including metal electropolishing and electrochemical machining. Electrochemical etching of high-value materials from mineral deposits has become increasingly accessible and efficient due to this, allowing collaboration with the Centre for Sustainable Resource Extraction (led by Prof Gawen Jenkin) and other institutions in the Met4Tech (UKRI) project to investigate critical resource extraction and recovery from technology waste streams, including batteries and electronics such as printed circuit boards (PCBs), photovoltaics and thermoelectric generators (TEGs).Citation1–3 In collaboration with the Faraday Institution, the ReLiB project is currently developing sustainable methods for the recycling of lithium-ion batteries from electric vehicles (EVs) and this has progressed to relithiating recycled cathode material.Citation4 Novel ionic liquid-based electrolytes have allowed for new battery systems to be developed for reactive metals, including aluminium (Horizon 2020).Citation5
The Mechanics of Materials (MoM) group is one of eight research groups based in the School of Engineering and, at the time of writing, has 12 academic staff, who each supervise a number of PhD researchers covering a multitude of projects. Those of interest include the production of nanocomposite coatings to improve wear and corrosion resistance, the production of hydroxyapatite nanocomposite coatings of titanium-based bioengineered medical implants for medical application, the investigation into the effect of cryogenic-treated coatings, and metallurgical studies for the processing of novel steels and aerospace alloys.Citation6–10 There is a well-established, long-standing collaborative relationship between TWI Ltd. and University of Leicester, called the Materials Innovation Centre (MatIC), which is based in Cambridge and led by Dr Shiladitya Paul and Prof Hongbiao Dong.Citation11 This centre focuses on materials characterisation, materials modelling, materials performance evaluation, thermal and cold spray coatings, corrosion, and novel materials development.
Space Park Leicester (SPL) was established as a research institute in 2022, as a continuation of the Space Research Group founded by Prof. Ken Pounds at the university in 1960.Citation12 SPL collaborates on projects with international space agencies (including ESA and NASA) and hosts many local and industrial partners on site, including Rolls-Royce, AWE and CGI. Collaborations between SPL and CSMP include developing functional interfaces and novel nanocomposite diffusion barrier coatings for thermoelectric materials used in radioisotope power systems (RPS) in increasingly demanding environments, and the high efficiency sustainable extraction and recycling of critical resources for off-world applications.Citation13–14 These collaborations bring together all of the aforementioned research groups, centres and institutes who combine and apply their work to a common cause and application.
The following case studies will briefly outline four recent or ongoing projects which fall within the scope of Transactions of the IMF.
2. Case studies of research at University of Leicester
2.1. Project 1: electropolishing of nickel-based HIP consolidated and cast aerospace forms using deep eutectic solvents as a replacement for conventional aqueous electrolytes
CSMP has a long-standing collaborative relationship with Rolls-Royce, with projects focussed on finding improvements to processing aerospace materials for jet engines. The extreme temperatures that can be found inside the gas turbine of the engine has resulted in the development of nickel-based superalloys.Citation15 These alloys have a finely tuned elemental composition and microstructure to make the material capable of operating much closer to its melting point. These parts are cast in moulds made of different materials dependent on the properties required of the component. Hot isostatic press (HIP) consolidation is carried out in steel moulds to refine the microstructure and reduce porosity in the material, however an iron-rich layer forms on the surface of the part. This is detrimental to the performance of the alloy, and so must be removed. This is typically done by chemical etching in nitric acid and then by electropolishing in a proprietary aqueous electrolyte composed of alkyl sulphonic acids and glycolic acids, which have a high cost and low efficiency leading to high energy costs. Researchers from CSMP developed a deep eutectic solvent (DES)-based electrolyte which was shown to be effective at removing the Fe-rich layer and was of low toxicity, sustainable and relatively low cost ().
Figure 1. Secondary electron SEM images of RR1000 HIP alloy electropolished for 120 min at 10 V in 2Eg:ChCl, (a) image showing the boundary region between unpolished (top) and polished (bottom) surfaces, (b) SEM in profile showing the etch-step edge (taken with permission from ref. Citation15).
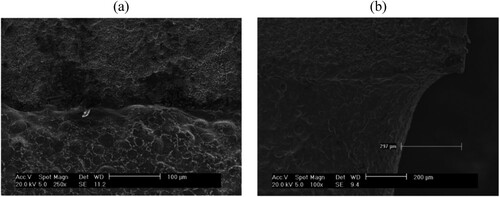
Ceramic moulds are used in single crystal casting, such as for turbine blades.Citation16 During the solidification and cooling process, the mould and metal component contract and separate slightly and, in the exposed areas, a mixed metal oxide deposit forms. This must be removed before further processing of the component as the deposit masks the metal below, preventing grain structure assessment. As with the nickel-based HIP consolidated components, a DES-based electrolyte and electropolishing process were developed to effectively remove surface oxide scale from the turbine blades, providing a more sustainable, less toxic and cheaper alternative than the strong inorganic acid commercial etchant previously used ().
Figure 2. Rolls-Royce Trent IP turbine blade before (top) and after (bottom) electropolishing in 2Eg:ChCl for 30 min in a six-blade batch trial process (taken with permission from ref. Citation16).
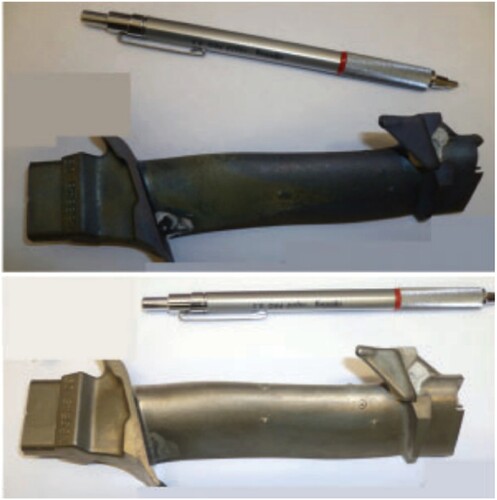
2.2. Project 2: production of nanocomposite coatings via a novel pulse reverse plating technique
Metal Matrix Nanocomposite (MMNC) coatings provide the ability to produce materials with enhanced properties, which can be tailored to suit a variety of applications based on the nanoparticle and bulk metal matrix chosen.Citation17 For example, silicon carbide (SiC) can be put into a copper matrix to produce a coating with a hardness greater than that of the pure metal, whilst maintaining high electrical and thermal conductivities. Typically, these nanocomposites are produced by electrochemical (co-deposition) or mechanical (milling and mechanical alloying) methods, however, neither of these methods are ideal. Co-deposition has little control of nanoparticle concentration, therefore is uncontrolled and not reproduceable. Milling takes many hours to form a sufficient dispersion, and this is followed by a sintering process which is extremely energy intensive, requiring high temperatures and pressures. Pulse reverse plating (PRP), combined with the use of an anionic surfactant, offers a controlled mechanism for delivering a high concentration of nanoparticles to the coating during the anodic pulse, which are subsequently captured and encapsulated in the cathodic pulse. This technique is controlled by adjusting the charge quotient of the process and the surfactant to particle ratio, allowing for a tailorable coating composition. To date, this method has been successfully applied to cobalt-MMNC coatings containing SiC () and Al2O3, and work is ongoing to apply it to copper and nickel-MMNC coatings containing a variety of nanoparticles.
Figure 3. Back-scattered electron SEM images of cobalt-SiC nanocomposite, produced by pulse-reverse plating (PRP) technique (taken with permission from ref. Citation18).
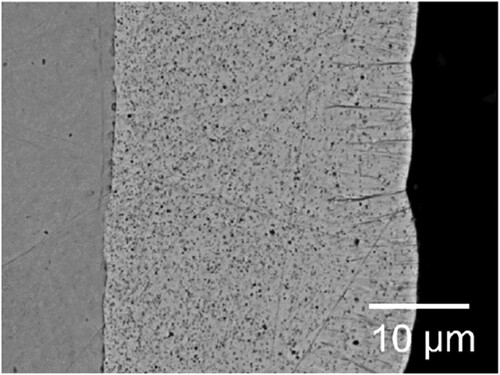
2.3. Project 3: recycling and recovery of critical resource materials
As previously mentioned, there are multiple projects ongoing at CSMP, in collaboration with other institutions, research centres at University of Leicester, and industry partners. Researchers on the UKRI-funded Met4Tech project developed a toolbox () for improved recycling of critical metals and materials in low-carbon technologies, to replace current pyrometallurgical and hydrometallurgical methods.Citation19 These tools can be implemented as best needed based on the material that needs recycling, and include dissolution of metals with DESs and brines, dissolution and removal of polymers from mixed phase components to aid the recovery of metals, high-intensity ultrasonication to generate local mechanical separation, electrocatalytic dissolution of metals using Earth-abundant oxidising agents such as iodine or iron chloride and designing technology to aid end-of-life recycling.
Figure 4. Toolbox of techniques to process a range of metallic and semiconductor structures in organic and inorganic phases (adapted from ref. Citation20).
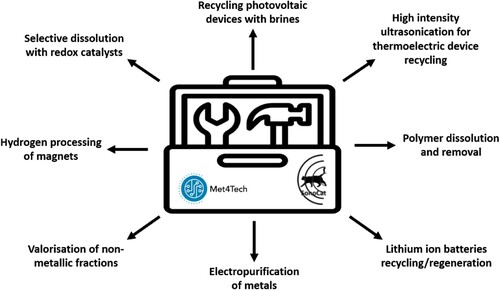
The ReLiB project, funded by the Faraday Institute, is centred around the recycling of Li-ion batteries. Harper et al. predicted that 250,000 tonnes of battery waste would be produced from the one million electric vehicles sold in 2017 alone once they reach end of life (EOL).Citation21 In 2020, three million electric vehicles were registered, making the global stock around 10 million. These figures have only grown exponentially in the last 3 years, meaning the amount of battery waste produced when vehicles reach EOL is going to be on the order of millions of tonnes. Researchers at CSMP in collaboration with other institutions on the project, proposed a method to use high-intensity ultrasound to remove the active electrode material from the metal foil.Citation22 This is effective but the ‘black mass’ of active material recovered is still very difficult to separate into its constituent parts. Work was subsequently done to investigate the use of water-soluble binders to replace the commonly used polyvinylidene fluoride (PVDF) binder which holds the active material together.Citation23 PVDF also has very poor solubility in N-methyl-2-pyrrolidone (NMP) which has high cost and toxicity. Further to this, the decomposition of PVDF, under the high temperatures it requires, forms by-products including HF and CO. Scott et al.Citation23 proposed the use of gelatin and alginate-based binders, which are water soluble and showed comparable cycling behaviours against conventional materials. Most recently, the relithiation of cathode material has been investigated, to allow circularity and complete reuse of the materials, and is showing very promising results ().Citation4
Figure 5. Images showing a battery anode before and after processing with different ultrasonication techniques. An ultrasonic bath (power intensity ∼ 0.02 W cm−2) at room temperature for 5 mins was used in images (a–d). (a) and (b) show electrode material made with gelatin binder and (c) and (d) show electrode material made with sodium alginate binder. Images (e) and (f) show the effect of a high-powered ultrasonic horn (power intensity ∼ 398 W cm−2) on the gelatin electrodes at 10% power for 5 s (taken with permission from ref. Citation23).
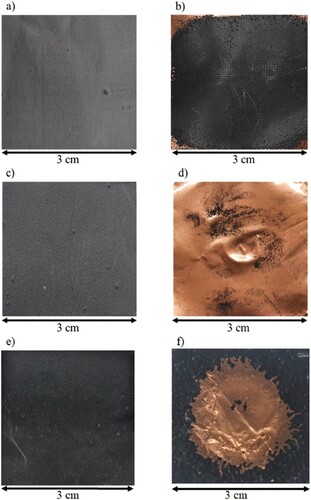
University of Leicester are research and development partners with deep tech company, DEScycle.Citation24 DEScycle were established in 2018, building on the DES technology discovered and patented at Leicester in 2003 by Prof Andy Abbott, and filed their maiden patent in 2021.Citation25–26 They customise DES formulations allowing them to tailor solutions to recover and recycle valuable metals from minerals and a variety of waste streams, including e-waste, fossil fuels and electricity production. Dr Rob Harris, former research fellow at the University, is the Chief Technology Officer of DEScycle and was awarded the Future Leaders Fellows award from UKRI in 2022.Citation27 In the last couple of years, their e-waste recycling lab pilot has since achieved technology readiness level (TRL) 6, and they have been named among the UK’s Most Innovative New Businesses, Bloomberg UK’s Top 25 Startups to Watch and CIRCULAZE’s Top 21 Circular Economy Start-Ups.
2.4. Project 4: Materials Innovation Centre (MatIC)
Materials Innovation Centre (MatIC) was set up by the University of Leicester in 2016 as a strategic partnership between themselves and TWI Ltd., focusing on materials characterisation and novel materials development.Citation11 The centre brings together the expertise of the two organisations to focus on the fundamental understanding of metallic materials, and how the properties and performance are related to processing-depending microstructures. They also work with industrial partners to secure a portfolio of funding from external sponsors, allowing for joint research programmes and ventures. Recent work by TWI Ltd. and University of Leicester in the Materials Innovation Centre (MatIC) collaboration has focused on corrosion, coatings for energy-intensive industries, high entropy alloys (HEAs) for aerospace and multi-layer materials recycling strategies.
Sol-Rec2, funded by the EU’s Horizon 2020 grant, investigates reducing pharmaceutical multi-layer material packaging waste by developing and implementing innovative strategies to improve the sorting, separation and recycling of blister packs and laminated packaging. CSMP researchers carry out life cycle assessments (LCA) for these processes and these assessments help researchers to determine the route of investigation and which environmental impacts need to be reduced the most.
Recent publications from MatIC include in-situ imaging and electrochemical monitoring of damaged thermal spray aluminium coatings in synthetic seawater, the use of electrodeposited copper catalysts for CO2 conversion to valuable products, the effect of corrosion products and deposits on the damage tolerance of TSA-coated steel in artificial seawater and the corrosion performance of electrodeposited zinc and zinc-alloy coatings in marine environments ().Citation28–31
Figure 6. Optical-electrochemical monitoring of thermal sprayed aluminium coating with 30% of exposed steel after removing the calcareous deposits formed on the top of the steel (C-30R). Top: open circuit potential (OCP) measurement over 30 s. Bottom: Original and processed sequential images (taken with permission from ref. Citation28).
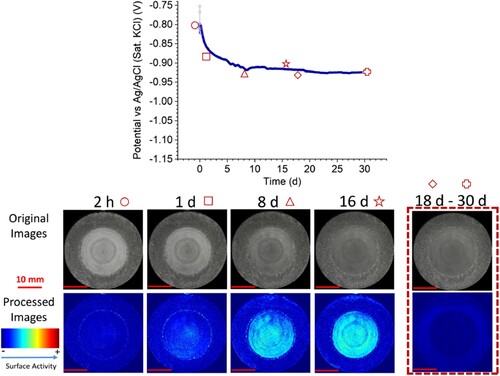
3. Vision for the future
The future of surface finishing at University of Leicester must have sustainability at its heart. With the current environmental and climate issues, reuse and recycling of materials must be more efficient, accessible and financially viable for industrial scale-up and implementation. Working with the local community is important to us: educating in schools and providing accessible information to bring awareness to what sustainability actually means and how to truly be ‘sustainable’. We want to continue to help industrial partners become more energy and resource efficient and continue to build our portfolio of collaborators and partners with an ultimate goal of achieving a circular economy.
Our long-term goal within the University of Leicester, is to draw together these efforts from each individual research group and centre into a singular resourced entity, sharing knowledge, expertise and equipment, whilst working with the community towards the common goal of developing resource efficiency and sustainability in the sector.
Disclosure statement
No potential conflict of interest was reported by the author(s).
References
- Met4Tech: 2021, Met4Tech home page [online]. Available at: https://www.met4tech.org.
- R. M. Rivera, C. E. Elgar, B. Jacobson, A. Feeney, P. Prentice, K. Ryder and A. P. Abbott: RSC Sustain., 2024, 2, 403–415. doi:10.1039/d3su00147d.
- G. Zante, E. Daskalopoulou, C. E. Elgar, R. M. Rivera, J. M. Hartley, K. Simpson, R. Tuley, J. Kettle and A. P. Abbott: RSC Sustain., 2023, 1, 1025–1034. doi:10.1039/d3su00087g.
- T. Yingnakorn, J. Hartley, J. S. Terreblanche, C. Lei, W. M. Dose and A. P. Abbott: RSC Sustain., 2023, 1, 2341–2349. doi:10.1039/d3su00237c.
- A. J. Lucio, I. Sumarlan, E. Bulmer, I. Efimov, S. Viles, A. R. Hillman, C. J. Zaleski and K. S. Ryder: J. Phys. Chem. C, 2023, 127(28), 13866–13876. doi:10.1021/acs.jpcc.3c02302.
- D. Albusalih, S. Gill, D. Weston and F. Altmann: Trans. IMF, 2019, 97(4), 203–216. doi:10.1080/00202967.2019.1634883.
- J. H. Shepherd, D. V. Shepherd and S. M. Best: J. Mater. Sci.: Mater. Med., 2012, 23, 2335–2347. doi:10.1007/s10856-012-4598-2.
- R. Thornton, T. Slatter and H. Ghadbeigi: Wear, 2013, 305(1-2), 177–191. doi:10.1016/j.wear.2013.06.005.
- J. Wang, G. M. A. M. El-Fallah, Z. Wang, H. Li, H. Dong and Q. Tao: Mat. Sci. Eng.: A,, 2023, 884, 145538. doi:10.1016/j.msea.2023.145538.
- Z. N. Yang, C. B. Liu, C. Y. Zhang, Q. W. Fang, Y. G. Li, G. M. A. M. El-Fallah, S. W. Ooi and F. C. Zhang: Mat. Sci. Eng.: A, 2021, 825, 141848. doi:10.1016/j.msea.2021.141848.
- TWI Ltd. Materials Innovation Centre [Online]. Available at: https://www.twi-global.com/innovation-network/centres/innovation-centres/materials-innovation-centre.
- Space Park Leicester. About us [Online]. Available at: https://www.space-park.co.uk/about/.
- H. M. Sargeant, R. Mesalam, E. J. Watkinson, A. Barco, R. M. Ambrosi, L. Gard, L. Trager, B. Lara, E. Scougal and T. Tinsley. ‘Ice-mining on the moon with radioisotope power systems’, NETS 2023 Conference Proceedings, 494–498, May 2023, doi:10.13182/NETS23-41840
- E. J. Watkinson, R. Mesalam, J.-F. Vigier, O. Beneš, J.-C. Griveau, E. Colineau, M. Sierig, D. Freis, R. M. Ambrosi, D. Staicu and R. J. M. Konings: Thermo, 2021, 1(3), 297–331. doi:10.3390/thermo1030020.
- A. J. Goddard, R. C. Harris, S. Saleem, M. Azam, C. Hood, D. Clark, J. Satchwell and K. S. Ryder: Trans IMF, 2017, 95(3), 137–146. doi:10.1080/00202967.2016.1270616.
- A. P. Abbott, N. Dsouza, P. Withey and K. S. Ryder: Trans. IMF, 2013, 90(1), 9–14. doi:10.1179/174591912(13228247936644.
- H. Hilton-Tapp, J. Kelly and D. Weston: Trans. IMF, 2023, 101(4), 179–188. doi:10.1080/00202967.2023.2207900.
- D. P. Weston, D. Albusalih, H. Hilton-Tapp, D. Statharas, S. P. Gill, J. Navajas, J. Cornec and N. J. Weston: Mat. Chem. Phys., 2023, 305, 127943. doi:10.1016/j.matchemphys.2023.127943.
- G. Zante, C. E. Elgar, J. M. Hartley, R. Mukherjee, J. Kettle, L. E. Horsfall, A. Walton, G. D. J. Harper and A. P. Abbott: RSC Sustain., 2024, 2, 320–347. doi:10.1039/d3su00390f.
- C. Pettit, S. King, A. P. Abbott, C. E. Elgar and G. Zante. ‘A toolbox for sustainable metal recycling’, Met4Tech Press Release [Online], Sept 2023, Available at: https://met4tech.org/about/.
- G. Harper, R. Sommerville, E. Kendrick, L. Driscoll, P. Slater, R. Stolkin, A. Walton, P. Christensen, O. Heidrich, S. Lambert, A. P. Abbott, K. S. Ryder, L. Gaines and P. Anderson: Nature, 2019, 575, 75–86. doi:10.1038/s41586-019-1682-5.
- C. Lei, I. Aldous, J. M. Hartley, D. L. Thompson, S. Scott, R. Hanson, P. A. Anderson, E. Kendrick, R. Sommerville, K. S. Ryder and A. P. Abbott: Green Chem., 2021, 23, 4710–4715. doi:10.1039/d1gc01623g.
- S. Scott, J. Terreblanche, D. L. Thompson, C. Lei, J. M. Hartley, A. P. Abbott and K. S. Ryder: J. Phys. Chem. C, 2022, 126(19), 8489–8498. doi:10.1021/acs.jpcc.2c01282.
- Argo Natural Resources Limited (Trading as Descycle). ‘Expansion of collaboration with the University of Leicester’, Descycle Press Release [Online], Feb 2021, Available at: https://www.descycle.com/news/r%26d-at-the-uol.
- A. P. Abbott, D. L. Davies, G. Capper, R. K. Rasheed and V. Tam-Byrajah. ‘Ionic liquids and their use as solvents’, International Patent, WO2002026701A2, Apr.4 2002.
- Argo Natural Resources Limited (Trading as Descycle). ‘Filing of maiden patent’, Descycle Press Release [Online], Sept 2021, Available at: https://www.descycle.com/news/patent-filing.
- Argo Natural Resources Limited (Trading as Descycle). ‘Dr Rob Harris awarded £1.2 m under future leaders fellowship from UKRI’, Descycle Press Release [Online], Jun. 2022, Available at: https://www.descycle.com/news/uk-grant-awarded.
- A. Castro-Vargas and S. Paul: Electrochim. Acta, 2023, 464, 142847. doi:10.1016/j.electacta.2023.142847.
- K. K. Maniam, M. Maniam, L. A. Diaz, H. K. Kukreja, A. I. Papadopoulos, V. Kumar, P. Seferlis and S. Paul: Processes, 2023, 11(4), 1148), doi:10.3390/pr11041148.
- A. Castro-Vargas, S. Gill and S. Paul: Surfaces, 2022, 5(1), 113–126. doi:10.3390/surfaces5010005.
- K. K. Maniam and S. Paul: Corros. Mater. Degrad., 2021, 2(2), 163–189. doi:10.3390/cmd2020010.