ABSTRACT
The tectonic setting of the Jiamusi–Khanka Massif in the eastern sector of the Central Asian Orogenic Belt (CAOB) in NE China is still highly controversial. This review considers all existing data to evaluate the origin of the Jiamusi–Khanka Massif. The Precambrian basement in the Jiamusi–Khanka Massif is dominated by the Neoproterozoic orthogneisses (898–891 and 757–751 Ma), the Mashan Complex (peak metamorphism at 530–500 Ma), and syntectonically deformed early Palaeozoic granitoids (530–484 Ma) related to the metamorphism of the Mashan Complex. The Mashan Complex reveals a clockwise orogen-style P–T path with peak conditions of 850–870°C/8–9 kbar followed by isothermal decompression. It was intruded by syn- (530–500 Ma) and post-tectonic (490–476 Ma) granitic rocks. The Jiamusi–Khanka Massif cannot be directly derived from the North China, South China, or Tarim cratons. Instead, it may have originated in the Siberian Craton and formed once part of the Altai-Sayan and Baikal orogens at ~500 Ma. For the Jiamusi Massif, we developed a plate tectonic model, and relate the final granulite facies metamorphism to slab break-off after consumption of a branch of the Palaeo-Asian Ocean.
GRAPHICAL ABSTRACT
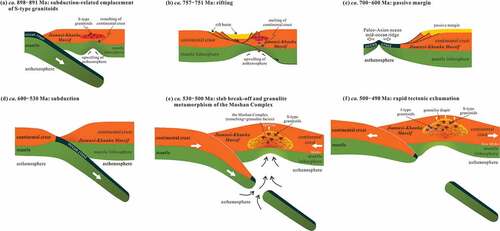
1. Introduction
The Central Asian Orogenic Belt (CAOB) comprises juvenile crust embedded by Mesoproterozoic blocks and extends from the Urals in the west to the Sea of Japan in the east (Şengör et al. Citation1993; Jahn et al. Citation2000; Xiao et al. Citation2004; Tang et al. Citation2017). CAOB is located between the East European, Siberian, North China, and Tarim cratons. Its evolution spans from the latest Mesoproterozoic to late Palaeozoic accretionary orogenies and continental growth over a period of some 800 million years (Chen et al. Citation2014, Citation2015a, Citation2015b; Eizenhöfer et al. Citation2014, Citation2015a, Citation2015b; Han et al. Citation2015, Citation2016a, Citation2016b; Kovalenko et al. Citation2004; Kröner et al. Citation2014; Safonova et al. Citation2011; Windley et al. Citation2007; Zhang et al. Citation2015a, Citation2015b, Citation2015c, Citation2016). NE China is part of easternmost CAOB and is considered to consist of a collage of micro-continental blocks (Tang, Citation1990; Tang et al. Citation1995; Li et al. Citation1999; Li, Citation2006; Wu et al. Citation2007a, Citation2007b; Zhou et al. Citation2009), which are from northwest to southeast, the Erguna, Xingan, Songnen, Jiamusi, and Khanka Massifs ()) (Şengör et al. Citation1993; Wu et al. Citation2007a; Zhou et al. Citation2010a, Citation2010b, Citation2010c, Citation2014, Citation2015a, Citation2015b) sometimes also called Manchurides (with microcontinental basement) in contrast to Altaids, which are dominated by accretionary complexes (Şengör et al. Citation1993, Citation2022). However, this interpretation is at odds as subordinate Precambrian basement units also occur within Altaids (e.g. Demoux Citation2009; Demoux et al. Citation2009; Kröner et al. Citation2017). The basement massifs in NE China are separated by the Primorie, Dunhua–Mishan, Yitong–Yilan, Hegenshan–Heihe, Xinlin–Xiguitu, and Solonker–Xar Moron–Changchun Faults ()) (Wu et al. Citation2007a; Zhou et al. Citation2010a, Citation2010b, Citation2010c).
Figure 1. Schematic diagram showing main Precambrian tectonic units of central and Eastern Asia (modified from .Zhou et al. Citation2009 and updated by Demoux Citation2009 and Degtyarev et al. Citation2017). Note: Cambrian granulite-facies rocks in the Altai-Sayan and Baikal orogens of the southern Siberia Craton with ages for the Derba Massif are after Nozhkin et al. (Citation2004), the Kitoykin Massif is after Donskaya et al. (Citation2000), the Slyudyanka Massif after Salnikova et al. (Citation1998), the Baga Bogd Massif after Demoux et al. (Citation2009) and the Olkhon Massif after Gladkochub et al. (Citation2008). Cambrian granulite-facies rocks in NE China known from the Sandaogou and Liumao areas of the Jiamusi Massif are after Wilde et al. (Citation1997, Citation2000; for locations, see ()), the Mohe Complex of the Erguna Massif from Zhou et al. (Citation2011a), and the Hutou Complex of the Khanka Massif from Zhou et al. (Citation2010b) and Wilde et al. (Citation2010)
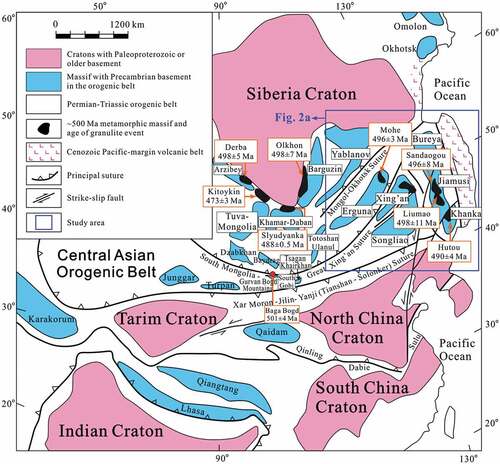
Figure 2. (a) Tectonic subdivision of NE China and Far East Russia (after Wilde et al. Citation2000; Wu et al. Citation2007b; Zhou et al. Citation2010b). The NE China orocline as demonstrated by Liu et al. (Citation2021) is also shown. (b) Simplified geological map of part of NE China, showing the distribution of Precambrian terranes with ages within the Jiamusi–Khanka Massif (modified from Wu et al. Citation2007b, Citation2011; Zhou et al. Citation2009). Note: Pink text and lines represent Neoproterozoic and early Palaeozoic granite rocks, the blue text and blue lines represent metamorphism in the Mashan Complex; the green text and green lines represent Neoproterozoic ophiolitic mélanges and bimodal intrusive rocks. (c) Schematic geological map of the Mashan Complex (modified after Wu et al. Citation2001).
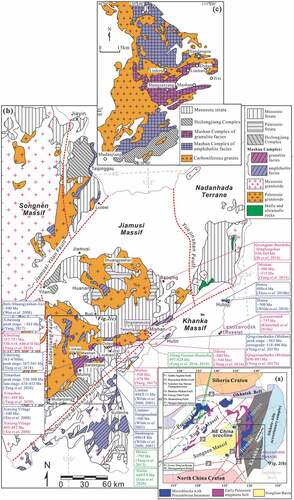
The Jiamusi and Khanka Massifs are regarded as two tectonic units (Wu et al. Citation2007a, Citation2011; Wang et al. Citation2012a, Citation2012b; Xu et al. Citation2013), separated by the Dunhu-Mishan Fault ()). In addition, Meng et al. (Citation2008) studied the Permian volcanic rocks at the southeastern margin of the Jiamusi Massif and further proposed that there was a residual Palaeo-Asian oceanic basin between the Jiamusi and Khanka Massifs, which was almost consumed prior to middle Permian times. Other scholars believe that the Jiamusi and Khanka Massifs have the same structural properties, a similar Precambrian basement composition (Cao et al. Citation1992; Zhang et al. Citation1998) and a similar geological evolution (Wilde et al. Citation2010; Zhou et al. Citation2010a, Citation2010b; Yang et al. 2014, Citation2015a; Yang Citation2017). In addition, the discovery of Triassic and Lower Cretaceous igneous rocks (227–203 and 118–110 Ma) from the Jiamusi and Khanka Massifs (Khanchuk et al. Citation2010b; Yang et al. Citation2012, Citation2015a, Citation2015b, Citation2018; Sun, Citation2013; Bi et al. Citation2015; Ma et al. Citation2016) also proves that the Jiamusi and Khanka Massifs have experienced similar magmatism and metamorphism spanning from the Neoproterozoic to Early Cretaceous (~755–115 Ma) (Wilde et al. Citation2010; Zhou et al. Citation2010a, Citation2010b; Yang et al. 2014; Yang Citation2017). More importantly, the Dunhua-Mishan Fault might have been formed during the Triassic, and its large-scale sinistral strike-slip displacement occurred during Jurassic to Cretaceous times (Zhu et al. Citation2006a, Citation2006b; Sun et al. Citation2008, Citation2010) ()). In addition, no ophiolitic mélange or accretionary complex has ever been reported from the areas around the Dunhua-Mishan Fault. To sum up, the Jiamusi and Khanka Massifs (together with the Bureya Massif in Russia in its present-day northern extension) should be regarded as a contiguous upper Neoproterozoic Jiamusi–Khanka(-Bureya) Massif.
The Jiamusi–Khanka Massif is one of the least-understood massifs in northeastern China. The age of its basement, the timing of the high-grade metamorphism, and the tectonic affinity and its possible linkage with Siberia, Gondwana, or the CAOB have all been controversial (e.g. HBGMR Citation1993; Jiang Citation1992; Lu et al. Citation1996; Lennon et al. Citation1997; Ren et al. Citation2010, Citation2012; Wilde et al. Citation2001, Citation2003; Wu et al. Citation2007a; Zhou et al. Citation2009, Citation2010a, Citation2018; Ge et al. Citation2021a, Citation2021b). Against this background, we review recent works on the composition and evolution of the Precambrian crystalline basement of the Jiamusi–Khanka Massif, aiming (1) to constrain the peak and retrograde timing of the granulite facies metamorphism, as well as the depositional age of the supracrustal rocks within the Mashan Complex; (2) to determine the tectonic setting of the early Palaeozoic magmatism and its relationship to the Cambrian high-grade metamorphism; (3) to discuss the tectonic affinity of the Jiamusi–Khanka Massif; and (4) to propose a preliminary tectono-magmatic evolutionary model for the Precambrian and Cambrian for the Jiamusi–Khanka Massif.
2. Basement properties of the Jiamusi–Khanka Massif
Tectonically, the Jiamusi–Khanka Massif is bounded by the Mongol–Okhotsk suture to the northwest, the Nadanhada Terrane and the Sikhote–Alin Terrane to the east, and the Songnen Massif to the west ()). A recent lithospheric-scale geophysical survey demonstrates the difference between microcontinental basement massifs and adjacent, more ocean-derived tectonic elements (Xin et al. Citation2021). The collisional Mongol–Okhotsk suture, which extends westward from the Okhotsk Sea to central Mongolia, is widely accepted as an important tectonic boundary between the Mongolia–North China Block and the Siberian component of a stable Eurasian continent (Zonenshain et al. Citation1990; Xu et al. Citation1997; Zorin, Citation1999; Cogné et al. Citation2005). The Nadanhada Terrane of NE China and the Sikhote–Alin Terrane of Russian Far East belong to the Palaeo–Pacific margin and are characterized by late Palaeozoic to early Mesozoic subduction accretionary complexes, and large-scale NE-trending igneous rock belts (Zhou et al. Citation2009, Citation2014; Sun et al. Citation2015a, Citation2015b; Wang et al. Citation2015b, Citation2017). The Songnen Massif includes the basement of the Songliao Basin and the Lesser Xing’an-Zhangguangcai Ranges ()). The Songliao basin developed upon a basement with weakly deformed and metamorphosed Phanerozoic granitoids and Palaeozoic units during the late Mesozoic (Wu et al. Citation2001, Citation2011; Meng et al. Citation2010). The Lesser Xing’an-Zhangguangcai Ranges are dominated by voluminous (60–70% of exposed rocks) Phanerozoic (mainly late Palaeozoic to early Mesozoic) granitoids, with rare Neoproterozoic gneissic granitoids, Palaeozoic sedimentary units as remnants surrounded by granitoids, and late Mesozoic–Cenozoic volcano-sedimentary assemblages (Meng et al. Citation2010; Wu et al. Citation2011).
The Jiamusi Massif consists of the Mashan and Heilongjiang ‘Groups’, now referred to as ‘Complexes’, together with Permian granitoids (Wu et al. Citation2011). The basement rocks of the Jiamusi Massif were traditionally thought as either Archaean (Dang and Li, Citation1993) or Proterozoic (Cao et al. Citation1992) in age. They were initially subdivided into two units, the Mashan and Heilongjiang Complexes. However, subsequent work has indicated that the sequence is not stratigraphic, consisting of a series of tectonic slivers. The Mashan Complex is characterized by khondalitic rocks (felsic granulite, marble, and graphitic schist), metamorphosed into granulite facies (Wilde et al. Citation1997). The Heilongjiang Complex is a sequence of high-pressure metamorphic rocks (mafic–ultramafic rocks, various quartzo–feldspathic schists, and radiolarian-bearing quartzite), metamorphosed up to epidote–blueschist facies during Jurassic times (the age of blueschist metamorphism is 145–185 Ma; Li et al. Citation2011; Zhou et al. Citation2009). Most geologists believe that the Mashan Complex is the basement, and the Heilongjiang Complex is the cover (Wu et al. Citation2007a; Li et al. Citation2011, Citation2014, Citation2020). The intrusive rocks of the Jiamusi Massif were mainly emplaced during three major stages: early Neoproterozoic (898–891 Ma and 757–751 Ma, respectively), Cambrian (530–484 Ma), and latest Carboniferous to earliest Triassic (302–246 Ma), the latter extending outside of the Jiamusi–Khanka Massif (Wilde et al. Citation2003, Citation2010; Khanchuk et al. Citation2010a; Zhou et al. Citation2010b; Wu et al. Citation2011; Bi et al. Citation2014, Citation2015, Citation2016; Yang et al. 2014, Citation2015a, Citation2017a, Citation2017b, Citation2018). The Phanerozoic granitoids (302–252, 110–98, and ~54 Ma), together with widespread volcanic rocks (290–267 Ma) (Meng et al. Citation2008; Bi et al. Citation2017), make up much of the massif ()).
The Chinese part of the Khanka Massif basement is poorly exposed, with outcrops restricted to Hulin, in the west, and Hutou, in the east (). The basement rocks were considered Neoarchaean in age during regional mapping (HBGMR, Citation1993). The rocks consist of khondalitic metasediments, marble, graphitic schist, and granitic gneiss (Mashan Complex, also called the ‘Hutou Complex’ in some literature). The granitoids in the Chinese portion of the Khanka Massif formed during a late stage of the early Palaeozoic orogeny, in the Permian active continental margin setting, and in the Late Triassic extensional environment (Yang et al. Citation2012, Citation2015a, Citation2015b; Wang et al. Citation2015a; Zhang et al. Citation2021). In that part of the Khanka Massif, detrital zircon ages extend back from Cretaceous to the earliest Neoproterozoic (Zhou et al. Citation2010a), but no Precambrian rocks have been recorded.
Following the recognition of the ca. 500 Ma metamorphic events in the Mashan Complex of the Jiamusi–Khanka Massif (Wilde et al. Citation1997, Citation2003, Citation2010; Zhou et al. Citation2010a), the tectonic nature of this massif and its initial tectonic position have attracted much attention (e.g. Wilde et al. Citation1997, Citation2000, Citation2003, Citation2010; Wu et al. Citation2007a, Citation2011; Yang et al. Citation2012, 2014, Citation2015a, Citation2015b; Zhou et al. Citation2010a, Citation2010b, Citation2011a, Citation2018). There is considerable progress in understanding the composition, structural style, metamorphism, geochemical characteristics, and geochronology of the basement rocks of the Jiamusi–Khanka Massif (Wilde et al. Citation1997, Citation2003, Citation2010; Wu et al. Citation2001; Zhou et al. Citation2010a, Citation2010b, Citation2011b; Zhou and Wilde Citation2013; Yang et al. 2014, Citation2015a, Citation2017b, Citation2018, Citation2019; Li et al. Citation2020), including the ~500 Ma peak metamorphic age of the Mashan Complex (Wilde et al. Citation1997, Citation2000), Neoproterozoic age of two groups of orthogneisses (898–891 and 757–751 Ma; Yang et al. Citation2017b, Citation2018, Citation2019, Citation2020a; Yang Citation2017), and deformed early Palaeozoic granitoids (530–484 Ma; Li et al. Citation1999; Wu et al. Citation2001, Citation2007b; Wilde et al. Citation2003; Li Citation2006).
2.1. Mashan Complex
The basement of the Jiamusi–Khanka Massif is dominated by rocks of the Mashan Complex. The Mashan Complex is composed of ‘khondalite series’ of amphibolite to granulite facies metasediments, intercalated with orthogneiss and two-pyroxene mafic granulite (); HBGMR, Citation1993; Wilde et al. Citation1999, Citation2000, Citation2001, Citation2010; Wu et al. Citation2007b, Citation2011; Zhou et al. Citation2009, Citation2010a, Citation2010b; Wilde et al. Citation2010; Khanchuk et al. Citation2010a). It was formerly defined by Cao et al. (Citation1983) as a lithostratigraphic group, consisting of four formations, named in ascending order: Ximashan (migmatite, migmatized leptynite, biotite-plagioclase gneiss, graphite schist, amphibole-plagioclase gneiss, and marble), Liumao (marble, schist, leptynite, migmatite, and metasomatite), Jiantang (schist, leptynite, hornfels, quartzite, and migmatite), and Majiajie (slate, phyllite, muscovite schist, marble, amphibolite, quartz schist, and metamorphic feldspathic quartz greywacke) Formations. The Majiajie Formation was later separated from the Mashan Complex (HBGMR, Citation1993; Wang, Citation1996). Huang et al. (Citation2009) suggested that it formed after the late Cambrian. The metamorphic degree of the Mashan Complex is not uniform in various areas (Cao et al. Citation1992; Jiang Citation1992; Lu et al. Citation1996; Ren et al. Citation2012; Geng et al. Citation2016) and can be divided into the Jixi–Mishan–Hulin granulite facies belt and Luobei–Huanan–Mudanjiang amphibolite facies belt ) (Geng et al. Citation2016). Yan et al. (Citation1989) suggested that the Mashan Complex and the Heilongjiang Complex may form a double (paired) metamorphic belt. However, Cao et al. (Citation1992) regarded the Mashan and Heilongjiang Complexes as two independent units and believed that the two protoliths formed in the Proterozoic. Wilde et al. (Citation1999, Citation2000, Citation2001) systematically analysed the rock types, metamorphism, and geochronology of the Mashan Complex, and proposed that the Mashan Complex in Jixi and Ximashan areas of the southern Jiamusi Massif has undergone granulite-facies metamorphism, accompanied by extensive migmatites. The considerable number of two types of dikes formed by distinct processes (structural emplacement or anatexis) indicates that the concept of a simple stratigraphic sequence cannot be applied to the Mashan Complex.
The Mashan Complex, a set of khondalite series dominated by metapelitic, felsic, and calc-silicate rocks, containing graphite- and phosphorus-bearing rocks, metamorphosed in amphibolite-granulite facies is characterized by a clockwise P–T path (Jiang, Citation1992; Wilde et al. Citation1997, Citation1999, Citation2000; Ren et al. Citation2012; Guo et al. Citation2014), with peak temperatures and pressures up to 850°C and 7.4 kbar, respectively (Jiang, Citation1992). Previous studies suggested that the Mashan Complex formed during the Neoarchaean (Dang and Li Citation1993; HBGMR Citation1993) or Palaeoproterozoic (Cao et al. Citation1992). According to recent precise LA-ICP-MS and SHRIMP zircon U-Pb dating, the detrital zircons of paragneisses from the Mashan Complex are not older than ca. 1050 Ma and not younger than ca. 696 Ma (Wilde et al. Citation2000; Yang et al. Citation2017b; Zhou and Wilde, Citation2013) suggesting a late Neoproterozoic depositional age (<696 Ma). Jiang (Citation1992) proposed that the primary sediments of the Mashan Complex (high-alumina metapelite, carbonaceous shale, calcareous shale, marl, and carbonate rock) are derived from erosion of K-rich granitic plutonic intrusions. These metasedimentary rocks are considered as shallow-marine shelf sediments formed at a stable passive continental margin (Jiang Citation1992; Lu et al. Citation1996). The Mashan Complex in the Jixi area contains a stable shelf sedimentary sequence of khondalitic affinity. Wilde et al. (Citation1999) considered that the metapelites and calc-silicate rocks were originally deposited in a passive continental margin setting, with graphitic sediments forming in restricted lagoons that were periodically open to the sea. The rocks were subducted, followed by a continental collision involving the crystallization of I-type granitoids and granulite-facies metamorphism (Wilde et al. Citation1999; Ren et al. Citation2010). In addition, based on geochemical constraints of immobile trace elements, Yang et al. (Citation2020b) showed that metapelites from the Mashan Complex are rapidly deposited during the early Neoproterozoic (1064–898 Ma) and originated from a continental island arc.
The Mashan Complex was intruded by early Palaeozoic granites (530–510 Ma) and underwent granulite-facies metamorphism at 510–490 Ma (Song et al. Citation1994; Wilde et al. Citation1999, Citation2000, Citation2003; Zhou et al. Citation2010b; Ren et al. Citation2012; Yang et al. 2014). Most recently, Yang et al. (Citation2017b, Citation2018, Citation2020a) discovered early Neoproterozoic magmatic zircon cores (ca. 895 and ca. 752 Ma) crystallized from granitic magma in the orthogneisses of the Mashan Complex, which were interpreted to relate to magmatism during the breakup of the Rodinia supercontinent.
2.2. Neoproterozoic orthogneiss
The ~757 Ma orthogneisses (two-pyroxene amphibole gneisses) formed in an unknown tectonic setting were found among the granulite-facies Iman Complex of the Khanka Massif in the Primorye region (Lesozavodsk; )), Russia, where metamorphism occurred at 507 Ma (Khanchuk et al. Citation2010a). The syenogranitic gneisses in the Qingxianshuiku area ()) had previously been mapped as a late Palaeozoic pluton (HBGMR, Citation1993) or was regarded as part of the ca. 513 Ma Yaoying pluton (Yang et al. 2014) based on lithological or structural relationships but not adequately constrained by precise geochronological data. Most recently, Yang et al. (Citation2017b, Citation2018) discovered two types of Neoproterozoic orthogneisses (898–891 Ma and 757–751 Ma, respectively) in the Qingxianshuiku area ()) of the central Jiamusi Massif. The enclaves of graphitic schist and marble here can be assigned to the Liumao Formation of the Mashan Complex based on field observations. The protoliths of the older type of Neoproterozoic orthogneisses are dominated by monzogranite, syenogranite, and syenite. The ca. 898–891 Ma syenogranitic magma was derived under low pressures from a crustal source that contained a high proportion of sedimentary rocks, perhaps in a subduction-related setting that was undergoing a transition from compression to extension. While the ca. 757–751 Ma granitic gneisses of the Jiamusi Massif and the ca. 757 Ma meta-basalts of the Khanka Massif (Khanchuk et al. Citation2010a) display a bimodal orthogneiss-basalt association, which has formed in a rift-related extensional environment.
2.3. Early Palaeozoic intrusive rocks
The products of early Palaeozoic magmatism are mainly granitic rocks, but there are also few reports on volcanic rocks and basic intrusive rocks. The granites occur mainly in Shuangyashan–Baoqing and Jixi–Mishan areas (Wilde et al. Citation1997, Citation2003; Li et al. Citation1999; Wu et al. Citation2001; Meng et al. Citation2008) and make up much of the Jiamusi Massif (). The major rock types are granodiorite, monzogranite, syenogranite, porphyritic granite, and garnet-granite, with massive and gneissic structures. In addition, there are similar early Palaeozoic intrusive rocks (530–450 Ma) in the Khanka Massif (Khanchuk et al. Citation2010a, Citation2010b; Wilde et al. Citation2010; Zhou et al. Citation2010b).
In recent years, high-precision geochronological studies have shown that the early Palaeozoic granites formed in the period between 530 and 484 Ma, most granites formed earlier than the age of regional granulite facies metamorphism at ~500 Ma (Wilde et al. Citation1997, Citation2000, Citation2003, Citation2010; Xie et al. Citation2008; Huang et al. Citation2009; Zhou et al. Citation2010b; Ren et al. Citation2010, Citation2012; Bi et al. Citation2014, Citation2015; Yang et al. 2014, Citation2018; Bi Citation2015; Yang Citation2017; Luan et al. Citation2017; Yang et al. Citation2020b). At present, only few studies on the petrogenesis and tectonic setting of the early Palaeozoic granites are available. Ren et al. (Citation2010) believe that they are high-K calc-alkaline I-type granites related to orogenic events. Bi (Citation2015) further proposed that they belong to post-collisional granites and originated from partial melting of the lower crust caused by the crustal collapse after crustal thickening. In addition, the rock associations of the Mishan region were emplaced in an extensional environment, including the ca. 515 Ma quartz syenites and the ca. 500 Ma bimodal gabbro-monzogranite association that were not affected by metamorphism (Yang et al. 2014). It was traditionally thought that the early Palaeozoic granitoids primarily originated from local anatexis of those Neoproterozoic rocks (Wilde et al. Citation1997, Citation2001; Ren et al. Citation2010). However, Yang et al. (Citation2018) concluded that the early Palaeozoic granitoids (490–476 Ma) formed in a post-collisional extensional setting and derived from melt injections from deep-seated sources in the crust, rather than in-situ anatexis after high-grade metamorphism.
3. Metamorphic evolution of the Mashan Complex
Recently, we have specifically studied the representative geological sections of three distinct regions, including the Liumao ()) (Yang et al. Citation2021a), Ximashan ()) (Yang et al. Citation2020b), and Shangsanyang ()) (Yang et al. Citation2021b) areas, and determined the metamorphic evolution of the Mashan Complex (Supplementary Table 1). These outcrops not only allow us to clarify the distribution characteristics of the rocks in the key study area but also provide new evidence for exploring the contact relationship between the succession of metamorphic and magmatic rocks.
Figure 3. Representative mesofabrics of the Mashan Complex in the Ximashan area (N45°12'45.2'', E130°28'21.0''). (a, b) A representative geological cross-section from the Ximashan area. (c) Geological sketch of (Figure 3(b)). Note that the ca. 500 Ma old Grt-bearing gneissic granite postdates the granulite facies metamorphism (stage M2) of the pelitic granulite. (d, e) The boundary between garnet-sillimanite gneisses and gneissic granites. (f) Field photograph of garnet-sillimanite gneisses. (g) Field photograph of the gneissic granite.
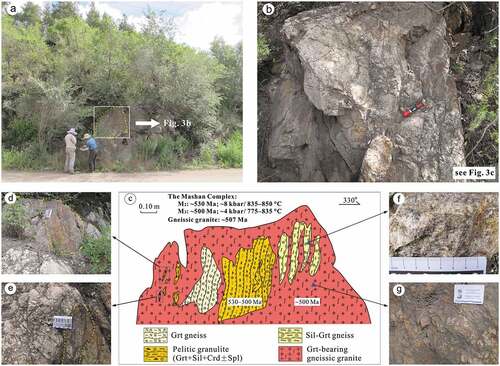
Figure 4. Photomicrographs of the Mashan Complex from the Ximashan area. (a) Garnet-sillimanite gneiss (Grt+Sil±Bi±Spl+Pl+Qz); (b) Metapelitic granulite (Grt+Sil+Crd+Bi±Spl±Kfs+Pl+Qz); (c) Garnet-sillimanite gneiss (Grt+Sil+Bi+Kfs+Pl+Qz); (d) Garnet-clinopyroxenite (Grt+Cpx+Pl+Qz); (e) Two-pyroxene granulite (Cpx+Opx+Pl+Qz); (f) Two-pyroxene garnet granulite (Grt+Cpx+Opx+Pl+Qz).
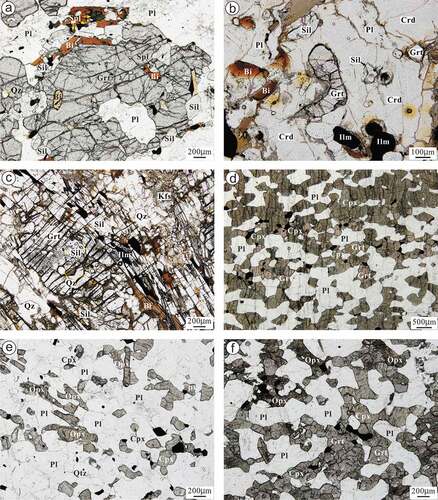
Figure 5. A representative geological section from the Liumao area (N45°13'4.06'', E130°46'5.36''). The typical macroscopic characteristics (a–i) of the studied samples are shown above the geological section.
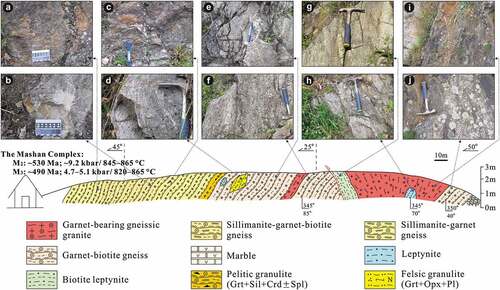
Figure 6. Photomicrographs of the Mashan Complex from the Liumao area. (a) Sillimanite-garnet gneiss (Grt+Sil±Pl+Qz); (b) Sil-Grt-Bi gneiss (Grt+Sil+Bi±Pl+Qz); (c) Garnet-biotite gneiss (Grt+Bi+Pl+Qz); (d) Felsic granulite (Grt+Opx+Pl+Bi+Qz); (e) Felsic granulite (Grt+Opx+Pl+Bi+Amp+Qz); (f) Metapelitic granulite (Grt+Sil+Crd+Bi±Spl+Kfs+Pl+Qz); (g) Biotite leptynite (Bi+Pl+Qz); (h) Leptynite (Pl+Qz).
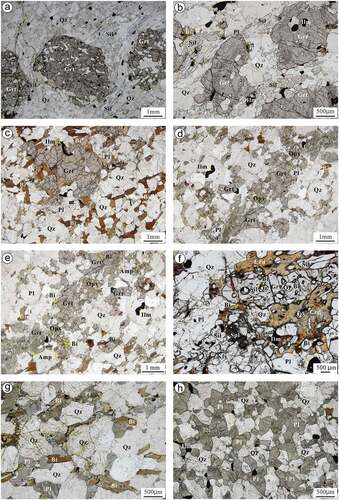
Figure 8. Schematic diagrams illustrating the tectono-magmatic evolution of the Jiamusi–Khanka Massif from early Neoproterozoic to Cambrian times.
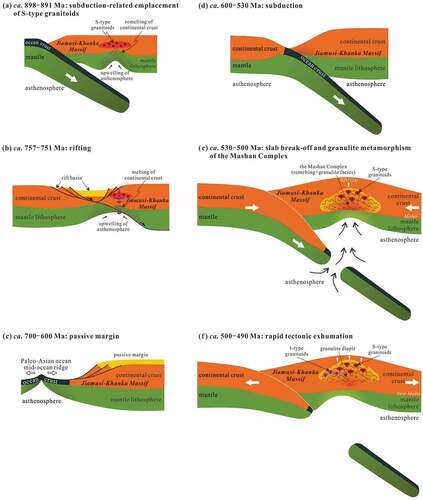
3.1. Lithologies and metamorphic overprint
In the following, we present and review exposures from two distinct areas with the aim to show lithologies, their metamorphic overprint, and the interaction between metamorphism and granite intrusions. In some metamorphic lithologies, detailed P–T conditions are known, and age data constraining the timing of metamorphic stages as well as the age of granite emplacement are available.
The exposure (), ~200 m northeast of Ximashan railway station, was selected from several outcrops near Ximashan to target the Ximashan unit of the Mashan Complex. The representative macroscopic and microscopic characteristics of the samples are shown in . The metamorphic rocks are mainly composed of three types: garnet gneiss (Grt±Bi±Kfs+Pl+Qz), garnet-sillimanite gneiss (Grt+Sil±Bi±Spl±Kfs+Pl+Qz; )), and metapelitic granulite (Grt+Sil+Crd+Bi±Spl±Kfs+Pl+Qz; )). The metapelitic granulite occurs as lenticular discrete enclaves in the gneissic garnet-bearing granites (). In addition, garnet-clinopyroxenite (Grt+Cpx+Pl+Qz; )), two-pyroxene granulite (Cpx+Opx+Pl+Qz; )), and two-pyroxene garnet granulite (Grt+Cpx+Opx+Pl+Qz; )) are also found in the Ximashan area (N45°12'29.0'', E130°27'30.9''). The foliated high-grade, granulite facies metamorphic rocks with metamorphic ages at ~530 Ma (M2 in )) represent enclaves within the schistose garnet-bearing gneissic granite, which was emplaced at ~500 Ma, and then metamorphosed within amphibolite facies conditions (M3 in ); Yang et al. Citation2020b). Consequently, two stages of metamorphism can be deduced in this outcrop from outcrop-scale fabrics.
The detailed cross-section of the Liumao area () is located ~0.4 km southeast of Fuqiang village and ~1.3 km southwest of Liumao railway station ()). Representative macroscopic and microscopic characteristics of samples are shown in ()), respectively. These metamorphic rocks comprise mainly two groups: metapelitic granulite (Grt+Sil+Crd+Bi±Spl+Kfs+Pl+Qz; )) and felsic granulite (Grt+Opx+Pl+Bi+Amp+Qz; )); amphibolite-facies sillimanite-garnet-biotite gneiss (Grt+Sil+Bi±Pl+Qz; )), sillimanite-garnet gneiss (Grt+Sil±Pl+Qz; )), and garnet-biotite gneiss (Grt+Bi+Pl+Qz; )). In addition, there is a small amount of marble, leptynite (Pl+Qz; )), and biotite leptynite (Bi+Pl+Qz; )). The minerals are aligned parallel to the general foliation of the metapelites. The felsic granulite occurs locally as lenticular lens in the garnet-biotite gneiss (). Consequently, from the relationships in this exposure, it can be deduced that granulite facies rocks are overprinted by a further stage of metamorphism within amphibolite facies conditions (see also Yang et al. Citation2021a). Again, outcrop-scale structures show two metamorphic stages and the relationships to granitoid intrusion ().
These relationships between metamorphic stages and later deformed granites are further constrained in other parts of the Mashan Complex as demonstrated by Yang et al. (Citation2021b).
From microstructures and reaction textures between mineral phases of the metapelitic and felsic granulites of rocks from the three studied locations shown above, four metamorphic stages are recognized: the early prograde (M1), peak (M2), post-peak decompression (M3), and final cooling (M4) stages (Supplementary Table 1).
The early prograde stage (M1) is characterized by mineral inclusions preserved in the core of the porphyroblastic garnet (), and include mostly quartz, with minor biotite and sillimanite, which show a preferred orientation ()).
The peak stage (M2) in the metapelitic granulites is represented by the appearance of plagioclase, K-feldspar, and further growth of garnet, biotite, and sillimanite in the matrix ()). The representative mineral assemblage of the peak stage (M2) in the felsic granulites is garnet, orthopyroxene, plagioclase, and quartz ().
The representative features of the post-peak decompression stage (M3) are represented by the formation of a cordierite corona or of a cordierite + spinel corona that appears close to the garnet in the metapelitic granulites (). The representative mineral assemblage of the post-peak stage (M3) in the felsic granulites includes amphibole, biotite, plagioclase, and quartz ()).
The final cooling stage (M4) is marked by the breakdown of minerals (such as rim of the garnet and orthopyroxene) and local recrystallization of melt close to the rim of the garnet, which consists of biotite + sillimanite + plagioclase + quartz + chlorite ()).
3.2. Formation and metamorphic ages of the Mashan Complex
Traditionally, the Mashan Complex was considered as either Archaean (Dang and Li, Citation1993; HBGMR, Citation1993; Zhao et al. Citation1995) or Neoproterozoic (Cao et al. Citation1992), based on unreliable geochronological data, such as hypersthene Ar-Ar, apatite Pb-Pb, phlogopite K-Ar, and whole-rock Rb-Sr ages (Wilde et al. Citation2001). During the last two decades, the SHRIMP U-Pb zircon dating technique has been applied to the Mashan Complex (Supplementary Table 2; Wilde et al. Citation1997, Citation2000, Citation2001, Citation2003) at Liumao, Sandaogou, and Ximashan, and a full review of the previously published dates is included in Wilde et al. (Citation2003). Studies on metasedimentary rocks yielded 1900–1050 Ma U–Pb ages from the detrital cores of the Mashan Complex (Wilde et al. Citation1997, Citation2000). Yang (Citation2017) reported the early Neoproterozoic (ca. 1064–898 Ma) LA–ICP–MS U–Pb ages on detrital zircons from the supracrustal paragneisses of the Mashan Complex in the Mashan area. These paragneisses are intruded by ~895 Ma old orthogneisses. Recently, further LA–ICP–MS U–Pb zircon dating results (Supplementary Table 2; Yang et al. Citation2020b, Citation2021a, Citation2021b) show that the deposition of the sedimentary rocks in the Liumao–Ximashan–Shangsanyang region occurred no earlier than early Neoproterozoic (ca. 898 Ma), and these rocks include Palaeoproterozoic sources (ca. 1927 Ma).
In addition, the timing of peak metamorphism is still controversial (Song et al. Citation1994; Wilde et al. Citation1997, Citation2000, Citation2003; Wen et al. Citation2008; Ren et al. Citation2010, Citation2012; Zhou et al. Citation2011a, Citation2011b, Citation2011c; Dong and Zhou, Citation2012; Zhou and Wilde, Citation2013; Yang et al. Citation2017a, Citation2017b, Citation2018). Wilde et al. (Citation1997) first proposed that the regional granulite facies metamorphism in the Jiamusi Massif took place at ca. 500 Ma based on the age of garnet-bearing granitic gneisses in the Liumao area. This conclusion has been further verified by other geochronological studies of the ortho- and paragneisses from the Mashan Complex (Supplementary Table 2; Wilde et al. Citation2000, Citation2003, Citation2010; Wen et al. Citation2008; Xie et al. Citation2008; Zhou et al. Citation2010a). For example, Wilde et al. (Citation2000) obtained 507–496 Ma ages from sillimanite gneiss and garnet-bearing granite from the Sandaogou and Ximashan areas in the Jiamusi Massif using SHRIMP U–Pb zircon method indicating granulite facies metamorphism at about 507–496 Ma. In addition, Wilde et al. (Citation2010) and Zhou et al. (Citation2010a) reported SHRIMP zircon U–Pb data for granitoids and a paragneiss in the Chinese segment of the Khanka Massif, and obtained 501 ± 8 Ma for the paragneiss and 491 ± 4 and 499 ± 10 Ma for two garnet-bearing granitoids. These data constrain the timing of the high-grade metamorphism to ca. 500 Ma. However, Ren et al. (Citation2012) questioned this viewpoint and argued that the granulite-facies metamorphism could have occurred at an earlier time than the formation of anhydrous anatectic granitoids (i.e. at ca. 530 Ma). Yang et al. (Citation2017b, Citation2018) recently proposed that the peak of the granulite-facies metamorphism in the Jiamusi Massif might have occurred at some time prior to 541–476 Ma, at around 567–561 Ma. The previous results and the latest zircon U-Pb dating of the metapelites from the Mashan area (Supplementary Table 2; Yang et al. Citation2020b) and the granulite-facies rocks from the Liumao (Supplementary Table 2; Yang et al. Citation2021a) and Shangsanyang areas (Supplementary Table 2; Yang et al. Citation2021b) indicate that the peak of the granulite-facies metamorphism of the Mashan Complex in the Jiamusi Massif might have occurred at 530–500 Ma (M2), post-peak decompression at 500–490 Ma (M3), and final cooling at 476–453 Ma (M4).
3.3. Metamorphism of the Mashan Complex
Two belts with different metamorphic grades are identified in the Mashan Complex, the Jixi–Mishan–Hulin granulite facies belt and Luobei–Huanan–Mudanjiang amphibolite facies belt (); Geng et al. Citation2016). The scholars considered that the metamorphic zonation is not related to the depth of burial but to variations in heat flow (Cao et al. Citation1992; Jiang Citation1992; Lu et al. Citation1996; Wilde et al. Citation1999; Ren et al. Citation2012; Geng et al. Citation2016). Although these two metamorphic belts reached different metamorphic grades, they are classified into one metamorphic zone because the principal-stage metamorphism occurred during the same late Cambrian orogeny (Xingkaian orogeny; Geng et al. Citation2016).
3.3.1. The granulite facies belt of the Mashan Complex
The granulite facies belt of the Mashan Complex (Cao et al. Citation1992; Jiang, Citation1992; Lu et al. Citation1996; Ren et al. Citation2012; Guo et al. Citation2014; Geng et al. Citation2016), which is dominated by khondalitic series rocks (granulite, marble, graphitic schist), is mainly distributed in the Jixi area, and a small part is also exposed in the Linkou–Boli area ()). The granulite facies belt is characterized by the presence of some high-temperature metamorphic minerals such as hypersthene, calcium-rich scapolite, wollastonite, kornerupine, and cordierite (Ren et al. Citation2012; Geng et al. Citation2016). The mineral assemblages of sillimanite–K-feldspar and cordierite–hypersthene occur in metapelites, and the development of cordierite and spinel in metapelites according to the reactions Sil + Bi → Grt + Crd, Sil + Grt → Spl + Crd, and Sil + Grt + Qz → Crd (Wilde et al. Citation1999). The subsequent widespread magmatism (510–498 Ma; Wilde et al. Citation1997, Citation1999, Citation2001, Citation2003, Citation2010; Zhou et al. Citation2010a, Citation2010b, Citation2011b; Ren et al. Citation2012; Yang et al. Citation2020b) was responsible for the intensive migmatization (~500 Ma; Xie et al. Citation2008; Ren et al. Citation2010; Yang et al. Citation2020b; Ge et al. Citation2021a, Citation2021b) and corresponding late amphibolite facies metamorphism, which eventually resulted in the partial melting of metapelitic rocks and formation of anatectic granitoids (Wilde et al. Citation1999; Ren et al. Citation2012; Geng et al. Citation2016; Yang et al. Citation2020b). In mafic rocks, the transition to granulite facies is determined by the breakdown of amphibole to form two-pyroxene–plagioclase assemblages, and the reaction is Amp + Qz → Opx + Cpx + Pl + H2O (Jiang, Citation1992; Lu et al. Citation1996; Ren et al. Citation2012). The peak conditions are estimated to be 800–850°C/7.4 kbar (Jiang, Citation1992; Lennon et al. Citation1997) by garnet-biotite and clinopyroxene-orthopyroxene thermobarometers or 820°C/8.0 kbar (Guo et al. Citation2014) by the ternary feldspar geothermometer and the garnet-sillimanite-plagioclase-quartz (GASP) geobarometer. Recently, the petrographic study and phase equilibria modelling of the granulite-facies rocks in the Ximashan–Liumao–Shangsanyang area by Yang et al. (Citation2020b, Citation2021a, Citation2021b) found peak (M2) metamorphic temperature and pressure conditions of ~8 kbar/835–850°C, 9.0–9.2 kbar/845–870°C, and 6.5–8.5 kbar/815–825°C, respectively, of the Mashan Complex in the Ximashan–Liumao–Shangsanyang areas (). However, most pressure data calculated from garnet-cordierite and GASP geobarometers concentrate at around 4.5–5.0 kbar (M3), indicating a sharp decline in pressure during cordierite crystallization (Jiang, Citation1992). The M3 metamorphism has an age of ca. 500 Ma (; Yang et al. Citation2020b, Citation2021a, Citation2021b). Retrograde metamorphism (M4) is common in the Mashan Complex, and the retrograde mineral assemblage of the amphibolite facies developed in the late stage of 476–453 Ma (Ren et al. Citation2012; Yang et al. Citation2020b).
3.3.2. The amphibolite facies belt of the Mashan Complex
In addition, the lower to higher amphibolite facies belt of the Mashan Complex (Cao et al. Citation1992; Liu, Citation1988; Dang et al. Citation1988; Ren et al. Citation2010, Citation2012) is distributed in the Linkou–Boli and Huanan–Luobei areas ()). The northern part of Jiamusi Massif is dominated by amphibolite facies metamorphism. Most areas exhibit lower amphibolite facies, while higher-amphibolite facies rocks only occur locally (Ren et al. Citation2012; Geng et al. Citation2016). The metapelites commonly contain andalusite, sillimanite, and garnet. The felsic gneiss, especially the migmatite, contains biotite + plagioclase + garnet ± amphibole. Additionally, a small amount of amphibolites occur in amphibolite facies metamorphic belt, rather than in the granulite facies metamorphic belt (Cao et al. Citation1992; Ren et al. Citation2012; Geng et al. Citation2016). The amphibolite facies belt is characterized by temperatures of ~650°C for the upper amphibolite facies and 500–550°C for the lower amphibolite facies, whereas pressures remain constant at 6.0–7.0 kbar (Jiang, Citation1992; Cao et al. Citation1992).
A few studies have focused on the metamorphic P–T path of the Mashan Complex (Jiang, Citation1992; Lu et al. Citation1996; Guo et al. Citation2014). The available data suggest that the Mashan Complex in the Jiamusi Massif underwent a regional metamorphism characterized by a clockwise P–T path (Jiang, Citation1992; Lu et al. Citation1996; Lennon et al. Citation1997). The earliest study by Jiang (Citation1992) suggests that the Mashan Complex is characterized by a clockwise P–T path from the initial increase in temperature and pressure (peak conditions of up to 850°C/7.4 kbar) to the near-isothermal decompression of the post-peak stage (), which was the same as that of typical collision orogenic belt (England and Thompson, Citation1984). Lu et al. (Citation1996) obtained a ‘hairpin-type’ clockwise P–T path for Mashan khondalite series rocks in the Liumao–Mashan area (), which is obviously different from that of a typical continental collision orogenic belt, as well as for continental extension and magmatic accretion settings (Lu et al. Citation1996). Guo et al. (Citation2014), constructed a tight clockwise P–T path with peak conditions of up to 820°C/8 kbar of the Al-rich metapelites in the Mashan Complex like that of a typical collisional orogenic belt (England and Thompson, Citation1984). Petrographic and P–T studies of the granulite-facies rocks in the Ximashan–Liumao–Shangsanyang area by Yang et al. (Citation2020b, Citation2021a, Citation2021b) show that the Mashan Complex underwent a clockwise orogen style P–T evolution of the decompression (isothermal decompression segment) and cooling from peak to retrograde stages ().
4. Petrogenesis of the Neoproterozoic–lower Palaeozoic rocks
4.1. Petrogenesis of the early Neoproterozoic orthogneiss (ca. 898–891 Ma)
Yang et al. (Citation2017b) discovered early Neoproterozoic orthogneisses in the Mishan region of the central Jiamusi–Khanka Massif. The protolith of the orthogneisses from the Mashan Complex is a ca. 898–891 Ma syenogranite (Supplementary Table 2). The syenogranitic rocks have high SiO2 (71.29–78.08 wt%) and low MgO (0.09–0.58 wt%) contents (Yang et al. Citation2017b) indicating their dominant derivation from a crustal source. Moreover, they are syn-collisional granites that were emplaced in a tectonic setting undergoing a transition from compression to extension. Yang et al. (Citation2017b) proposed that the 898–891 Ma syenogranitic magma was derived at low pressures from a crustal source with a high proportion of sedimentary rocks, perhaps in a subduction-related setting that was changing from compression to extension ()).
4.2. Petrogenesis of the middle Neoproterozoic orthogneiss (ca. 757–751 Ma)
The protoliths of the ca. 757–751 Ma orthogneisses from the Mashan Complex are dominated by monzogranite, syenogranite, and granite (Supplementary Table 2). All three types of granitic rocks exhibit similar geochemical characteristics: high SiO2 (>71.5 wt%), and K2O (>3.9 wt%), and low MgO (<0.8 wt%) and low Cr and Ni, suggesting possible crust-derived differentiated magmas or highly evolved magmas (Yang et al. Citation2018). Moreover, the εHf(t) values (+0.1 to +8.5) of the orthogneiss zircons, suggesting that the primary magmas could be derived at low pressures from a juvenile crust accompanied by a certain degree of involvement of ancient crustal rocks in their petrogenesis (Yang et al. Citation2018). This is further supported by the identification of the ca. 895 Ma syenogranitic gneisses with negative εHf(t) values from the surrounding Mishan region (Yang et al. Citation2017b). Notably, there was a depleted asthenosphere mantle within their source region that contributed both the heat and juvenile materials. Yang et al. (Citation2018) inferred that the middle Neoproterozoic orthogneiss (757–751 Ma) have formed in a rift-related extensional setting ()).
Detrital zircon ages from the Mashan Complex (called the ‘Hutou Complex’ in some literature) extend back to the earliest Neoproterozoic (Supplementary Table 2; Zhou et al. Citation2010a), but no Precambrian rocks have been discovered in the Chinese portion of the Khanka Massif. However, a ~ 757 Ma old orthogneiss formed in an undetermined tectonic setting was discovered in the Russian part of the Khanka Massif (Khanchuk et al. Citation2010a).
4.3. Petrogenesis of the early Palaeozoic intrusive rocks (ca. 530–484 Ma)
The early Palaeozoic intrusive rocks are widely distributed in the Jiamusi–Khanka Massif (Wilde et al. Citation2003, Citation2010; Xie et al. Citation2008; Huang et al. Citation2009; Zhou et al. Citation2010a; Ren et al. Citation2010, Citation2012; Bi et al. Citation2014, Citation2015; Yang et al. 2014, Citation2018; Yang Citation2017; Yang et al. Citation2020b), mainly including (all ages refer to and Supplementary Table 2): (1) the 530–484 Ma syenogranite, monzogranite, and granodiorite intrusions in the Baoqing-Shuangyashan region (Supplementary Table 2; Bi et al. Citation2014); (2) the 541–498 Ma gabbro, syenogranite, monzogranite, and quartz syenite intrusions in the Mishan region (Supplementary Table 2; Yang et al. 2014); (3) the 504 ± 2 Ma gneissic granites in the Huanan region (Supplementary Table 2; Huang et al. Citation2009); (4) the 530–476 Ma granitoids in the Xibeileng region (Supplementary Table 2; Yang et al. Citation2018); and (5) the 530–450 Ma granitic rocks in the Hutou region (Supplementary Table 2; Wilde et al. Citation2010; Zhou et al. Citation2010a). In all cases, the intrusive rocks postdate granulite facies conditions of country rocks, and some published ages older than ~510 Ma of intrusive rocks must be treated with caution, e.g. the 530 ± 5 Ma age is only the mean value of widely scattered zircon ages from a sample, in which the younger concordant ages are at 488 Ma (Bi et al. Citation2014). An unclear exception is the emplacement age of ca. 540 Ma of a syenogranite (Yang H. et al. 2014), which is younger than the amphibolite-grade metamorphic overprint of country rocks. For final clarification of this peculiar case, further work is needed. The 510–476 Ma granitoids in the Jiamusi Massif all belong to high-K calc-alkaline to the shoshonitic series and show a transitional feature from normal I-type granites to highly fractionated I-type granites (Ren et al. Citation2010, Citation2012; Bi et al. Citation2014, Citation2015; Yang et al. 2014, Citation2018). Based on the rock associations from the Mishan region emplaced in an extensional environment recognized by Yang et al. (2014), Yang et al. (Citation2018) proposed that the ca. 490–476 Ma granitoids were derived from the magmatic injection of melts from deep-seated sources in the crust, rather than from in-situ anatexis after high-grade metamorphism (Ren et al. Citation2010, Citation2012). Their primary magmas could have originated from a dominantly ancient crustal source with an average Palaeoproterozoic age and subsequently experienced significant crystal fractionation (Bi et al. Citation2014, Citation2015; Yang et al. 2014, Citation2018). Based on the geochemical and zircon U–Pb–Hf isotopic constraints from the Mashan Complex, the granites formed in a post-collisional extensional setting that resulted from the collapse of a Neoproterozoic to Cambrian global orogen (Ren et al. Citation2010, Citation2012; Bi et al. Citation2014, Citation2015; Yang et al. 2014, Citation2018; Yang et al. Citation2020b).
5. Tectonic evolution of the Jiamusi–Khanka Massif
In the following, specific questions are discussed first, and then plate tectonic models are proposed explaining the succession of geological events known in the Jiamusi–Khanka Massif.
5.1. Supracrustal rocks and early Neoproterozoic magmatism of the Mashan Complex
The protoliths of the khondalite series of the Mashan Complex are pelites, pelitic quartz sandstones, and feldspathic quartz sandstones. The potential sources of detritus of the khondalite series are weathered and erosive K-rich granitic rocks (Jiang, Citation1993). Yang (Citation2017) suggests that the protolith of some supracrustal rocks within the Mashan Complex was deposited during the early Neoproterozoic (ca. 1064–898 Ma), according to the detrital zircon age spectra of 2548–1064 Ma from the paragneisses in Mishan area, combined with the intrusion of ~895 Ma old orthogneisses. Therefore, we infer that the thinning of the continental crust formed an early Neoproterozoic continental rift basin, and granitic sources provided sediments for the khondalite series protoliths of the Mashan Complex (Yang et al. Citation2020b, Citation2021a). Yang H. (Citation202Citation0a) found two magmatic suites, a gabbro-granodiorite-monzogranite suite at 937–933 Ma and syenogranite at 896–891 Ma. These authors interpreted the magmatic suites as formed in an andino-type continental margin setting. This suggests that the rift was probably a back-arc rift.
5.2. Cambrian metamorphism and P–T path
As mentioned above, all scholars obtained the clockwise P–T paths (; Jiang Citation1992; Lu et al. Citation1996; Guo et al. Citation2014; Yang et al. Citation2020b, Citation2021a, Citation2021b), with peak temperatures and pressures up to 850–870°C and 8–9 kbar, respectively, (Yang et al. Citation2020b, Citation2021a, Citation2021b). The P–T path of granulite-facies metamorphism of the Mashan Complex was the same as that of a typical collisional orogenic belt and reveals that the dynamic process of the collapse of the orogenic belt is due to the uneven gravity distribution caused by the thickening of the lower crust during the collisional orogeny (England and Thompson, Citation1984; Condie et al. Citation1992; Brown, Citation1993). Significantly, the peak-metamorphic pressure of the Mashan Complex is at ~8 kbar (locally up to 9 kbar), indicating that the high-temperature metamorphism of the peak stage depends not only on crustal thickening but also on an additional heat source at depth. In addition, the representative features of the post-peak decompression stage, such as cordierite coronas or cordierite + spinel coronas, appear close to the rim of the garnet, demonstrating that the decompression process is not simply the result of crustal denudation, but more importantly, the result of rapid tectonic exhumation (Cawood et al. Citation1995).
Figure 9. (a, b) Precambrian microcontinents and basement massifs and early Palaeozoic metamorphic terranes of Central Asia (modified from Zhou et al. Citation2009 and updated by Demoux et al., Citation2009 and Degtyarev et al. Citation2017), and (c–e) relative probability plots of data from granulite-facies rocks and (metamorphic) magmatic rocks in NE China (Jiamusi and Khanka Massifs) and the southern Siberia Craton (Altai-Sayang and Baikal orogens). (c) Zircons from granulite facies rocks of the Mashan Complex and Neoproterozoic orthogneiss in the Jiamusi Massif. Data are from Wilde et al. (Citation1997, Citation1999, Citation2000, Citation2003) and Yang et al. (Citation2017b, Citation2018, Citation2020b, Citation2021a, Citation2021b); (d) zircons from granulite-facies rocks of the Hutou Complex and Neoproterozoic magmatic rocks in the Khanka Massif; data are from Khanchuk et al. (Citation2010a), Zhou et al. (Citation2010b) and Xiong et al. (Citation2020); and (e) zircons from granulite-facies rocks in the Derba, Kitoykin, Olkhon and Slyudyanka Massifs and (metamorphic) magmatic rocks in the Altai-Sayan and Baikal orogens at the southern margin of the Siberian Craton; data from Salnikova et al. (Citation1998), Donskaya et al. (Citation2000), Nozhkin et al. (Citation2004), Gladkochub et al. (Citation2008) and Kuzmichev and Larionov (Citation2011, Citation2013).
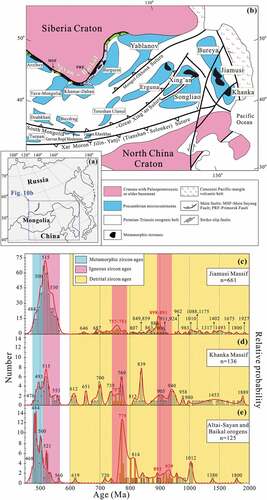
According to all the information mentioned above, the peak of the granulite-facies metamorphism in the Jiamusi Massif might have occurred at 530–500 Ma. The early Palaeozoic magmatism formed between 510 and 484 Ma, combined with the tectonic setting of the post-collisional extensional setting, suggesting that the magmatism was formed in a collapsing orogen after the near-isothermal decompressional metamorphic stage M3.
5.3. Tectonic evolutionary model of the Jiamusi–Khanka Massif
Based on the arguments presented above, the magmatism in the Jiamusi Massif can be divided into three stages:
Before the early Neoproterozoic (>898 Ma): the thinning of continental crust resulted in the formation of the continental rift basin in the early Neoproterozoic with the sedimentation of the khondalite series (Jiang, Citation1992; Lu et al. Citation1996). The protolith of the supracrustal rocks within the Mashan Complex was deposited during the early Neoproterozoic (ca. 1064–898 Ma; Yang Citation2017) and includes Palaeoproterozoic detritus (ca.1927 Ma; Yang et al. Citation2021b).
If the interpretation of the middle Neoproterozoic rifting is correct and the formation of the khondalite series on a passive margin (), the question arises whether the rift developed to a passive margin and the margin of an oceanic basin. Rifting is supported when the widespread 780–740 Ma mafic dike swarms along the southern margin of the Siberian Craton (Sklyarov et al. Citation2003; Gladkochub et al. Citation2007) are considered (see following chapter) as well as similar successions in the Ereendavaa Terrane close to the Siberian craton margin (Miao et al. Citation2020). There is no Neoproterozoic ophiolite between 750 and 530 Ma adjacent to the Jiamusi Massif except such in late Neoproterozoic ophiolitic mélanges (Mongush et al. Citation2011; Furnes and Safanova, Citation2019). A rift-related extensional environment can be favoured by the 697–628 Ma ophiolitic mélanges from the northern Great Xing’an Range (Feng et al. Citation2016, Citation2018; Gou et al. Citation2020), ca. 792 Ma bimodal intrusive rocks from the Erguna Massif of NE China (Tang et al. Citation2013). Then, during ca. 600–530 Ma, the palaeo-oceanic crust between the Jiamusi–Khanka Massif and the adjacent continental plate continued to subduct and demise (). Late Neoproterozoic (578 Ma) ophiolitic elements were incorporated in ophiolitic mélange systems (Mongush et al. Citation2011; Furnes and Safinova, Citation2019; Pfänder et al. Citation2021) are in support of the oceanic subduction ().
Figure 10. A speculative model showing the possible source region and subsequent drift history of the combined Khanka–Jiamusi–Bureya Massif from the Neoproterozoic to the early Palaeozoic (modified from Salnikova et al. Citation1998; Gladkochub et al. Citation2008; Zhou et al. Citation2010a).
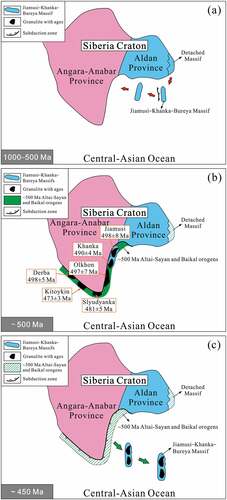
During the initial peak stage of orogeny (~530–500 Ma), interpreted continental subduction and subsequent collision, which resulted in thickening. Subsequent break-off of the subducted oceanic lithosphere, uprise of asthenospheric melts strongly heated the overlying mantle lithosphere, increased the temperature and heating started to remelt the lower crust, which caused rocks of the Mashan Complex to undergo progressive metamorphism at lower amphibolite facies to granulite facies conditions at the initial stage of the intraplate collisional orogeny (); Jiang, Citation1992; Lu et al. Citation1996; Yang et al. Citation2020b). However, due to the uneven distribution of the regional heat flow, the spatial intensity of metamorphism varies. In addition, it is accompanied by the formation of a small number of collisional S-type granites at 530–500 Ma (); Wilde et al. Citation1997, Citation1999, Citation2001, Citation2003, Citation2010; Zhou et al. Citation2010a).
A rapid tectonic exhumation stage (500–490 Ma) after the peak stage of metamorphism rapidly exhumed granulite-facies rocks to a shallow level of the crust. The temperature remained almost unchanged, and the pressure reduced obviously, accompanied by regional decompressional migmatization, which indicates the process of rapid crustal exhumation. The underlying process was the thermal thinning of the mantle lithosphere, which was responsible for unloading and exhumation of the granulite facies rocks of the Mashan Complex, also driven by the low density of granulite facies rocks and intruding magmatites ()). The study area reveals characteristics of an extensional tectonic environment, which led to voluminous K-rich granites (I-type) emplacement at ~500 Ma (; Ren et al. Citation2010, Citation2012; Yang Citation2017; Yang et al. Citation2020b). In the adjacent Bureya Massif, S- and A2-type granites intruded between 502 and 492 Ma (Ovchinnikov et al. Citation2020, Citation2021) being part of this late-stage magmatic event related to slab break-off.
The extensional collapse stage of the orogen (476–453 Ma) took place when the granulite-facies rocks were exhumed to shallow levels of the crust, resulting in the extensional collapse of the orogenic belt due to the uneven gravity (Dewey et al. Citation1993; Platt and England, Citation1994). The asthenospheric mantle upwelling caused partial melting of the lithospheric mantle and of the lower and middle crust, resulting in the emplacement of large post-orogenic intrusive bodies (490–476 Ma) after the early Palaeozoic in the extensional tectonic setting (Yang Citation2017; Bi et al. Citation2015). At the same time, the granulites were transformed into amphibolite-facies rocks by the retrograde metamorphic stage M4, which also affected and deformed the ~500 granitoids.
5.4. Tectonic affinity of the Jiamusi–Khanka Massif
Critical data of Neoproterozoic to Cambrian tectonic events in microcontinental blocks of eastern CAOB are summarized in (see ) for locations). All these blocks show evidence for the earliest Ordovician magmatism, but only the Erguna Block and the Olkhon terrane rocks within the Barguzin Block show evidence for Cambrian granulite or amphibolite facies metamorphism. At the present stage of knowledge, other microcontinental blocks, like Xing’an,Songliao, and Yablanov, lack evidence for Neoproterozoic magmatic and metamorphic events.
Table 1. Major Precambrian–Cambrian events in the microcontinents of the eastern CAOB.
As mentioned before, the Jiamusi and Khanka Massifs (together with the Bureya Massif in Russia) should be regarded as a contiguous massif during late Neoproterozoic-Cambrian times. Together with the early Palaeozoic granite belts, these form the NE China orocline surrounding the Songliao basin as recently demonstrated by Liu et al. (Citation2021) (). The orocline formed during the late Palaeozoic formation of the CAOB and includes the Jiamusi-Khanka-Bureya and Erguna Massifs.
In the following, details of some mentioned microcontinental blocks are discussed. The Erguna Massif is characterized by Meso-Neoproterozoic khondalitic rocks, which underwent a ~ 500 Ma metamorphic event (peak conditions of 710–740°C/5–6 kbar) (Zhou et al. Citation2011b, Xu et al. Citation2018), and the oldest granitoids (1800–700 Ma) reported in the NE China segment of the CAOB (Sun et al. Citation2012, Citation2013; Tang et al. Citation2013; Ge et al. Citation2015). The Palaeozoic and Mesozoic granites construct the main body of the Erguna Massif and are covered by Mesozoic sediments and volcanic rocks (Wu et al. Citation2011; Han et al. Citation2015). The Erguna Massif has a Neoproterozoic metamorphic basement although not as much widespread as traditionally reported, which makes the Erguna Massif different from the Xing’an, Songnen, and Jiamusi–Khanka Massifs that are lack or are poor of late Mesoproterozoic igneous rocks (Liu et al. Citation2017). The Xing’an Massif consists mainly of Palaeozoic granitoids, and sedimentary strata covered by voluminous Mesozoic volcanic rocks and granitoids (Wu et al. Citation2011). The previously thought Precambrian metasedimentary complexes within the Xing’an Massif are much younger in age. Detrital zircon U–Pb ages of 506–410 Ma indicate formation in late early Palaeozoic times. This fact makes the Xing’an Massif different from Jiamusi–Khanka Massifs (Liu et al. Citation2017). The basement of Songnen Massif consists of the late Palaeozoic-early Mesozoic crystalline rocks and some Precambrian metamorphic rocks (greenschist facies, detrital zircon U–Pb ages of 751–855 Ma) (Wu et al. Citation2000; Quan et al. Citation2013; Wang et al. Citation2013). Most of the granitoids in the area were emplaced during the Late Triassic-Middle Jurassic, with a limited number emplaced during the early Palaeozoic (Wu et al. Citation2011).
Other microcontinental blocks like the South Gobi also consist of Neoproterozoic ortho- and paragneiss, biotite- and hornblende-bearing schist with minor quartzites, amphibolites, and marbles, which are intruded by granites of 950 and 916 Ma (Şengör et al. Citation2022 and references therein). Another element is the Tuva-Mongol magmatic arc, which also comprises early Precambrian high-grade metamorphic rocks (Dzabkhan/Baidrag; ), Neoproterozoic to early Cambrian ophiolites and early Proterozoic island arc volcanics, overlain by late Neoproterozoic-Cambrian shelf carbonates and by early to late Palaeozoic granite intrusions. We exclude, therefore, a primary relationship of these two microcontinental blocks to the Jiamusi-Khanka-Bureya Massif. However, leucogranitic gneisses with an age of 501 ± 4 Ma are known from Gorvan Bogd in the central part of CAOB (Demoux et al. Citation2009) and Kröner et al. (Citation2010) report granite intrusion, metamorphism, and thrusting.
As a consequence, we discuss the arguments constraining the origin of the Jiamusi-Khanka-Bureya Massif. Firstly, the geological events recorded within the Jiamusi-Khanka-Bureya Massif, especially the granulite-facies metamorphism, were quite unique and different from those of other microcontinents like Erguna, Xing’an, and Songnen Massifs within the eastern CAOB, suggesting that the Jiamusi–Khanka Massif is probably an exotic fragment rather than a locally derived block in NE China (Wilde et al. Citation2010; Wu et al. Citation2011).
Secondly, the Neoproterozoic sedimentary and igneous rocks of the South China and Tarim cratons were only metamorphosed at greenschist and blueschist facies (ca. 780–825 Ma) conditions (Zheng et al. Citation2013; Zhao, Citation2014; Li et al. Citation2016), and high-grade (up to granulite-facies) rocks with ages of ca. 530 Ma like in the Jiamusi–Khanka Massif are absent within these two cratons.
Thirdly, ca. 895 and ca. 752 Ma granitic gneisses and large amounts of ca. 1100–700 Ma xenocrystic zircons have already been found from various units of the Jiamusi–Khanka Massif (Zheng et al. Citation2013; Li et al. Citation2016), but both the Siberian and North China cratons are characterized by a lack of late Mesoproterozoic to Neoproterozoic magmatism formed between 1100 and 700 Ma (Gladkochub et al. Citation2010; Levashova et al. Citation2010, Citation2011; Zhao et al. Citation2004; Zheng et al. Citation2013). These characteristics imply that the Jiamusi–Khanka Massif was not directly derived from the surroundings of the Siberian and North China cratons before the early Neoproterozoic. However, Gladkochub et al. (Citation2008) have recently determined that the granulite-facies metamorphism (ca. 500 Ma) of the Olkhon terrane of Lake Baikal in Siberia is characterized by a clockwise P–T path with a near-isothermal process, and their temperatures and pressures vary from 643°C to 877°C/4.5–10.0 kbar, showing a marked similarity to P–T conditions defined from the Mashan Complex of the Jiamusi–Khanka Massif (Yang et al. Citation2020b, Citation2021a, Citation2021b). Furthermore, the protoliths of the granulites within the Baikal orogen were mainly island arc mafic volcanic rocks and back-arc basin sediments, which is consistent with the results of recent studies in the Jiamusi–Khanka Massif (Zhou et al. Citation2010a; Yang et al. Citation2020b).
Salnikova et al. (Citation1998) dated the metamorphic episode of the Slyudyanka Complex in the southwestern Baikal region at the southern margin of the Siberian Craton lasting for ca. 20 million years (between 490 and 470 Ma), which is only slightly younger than the main principal metamorphic events in the Jiamusi–Khanka Massif, suggesting that they may be related to the same overall event (Zhou et al. Citation2010a, Citation2018). Indeed, there are marked similarities when the data of the time of peak granulite-facies metamorphism for the Baikal belt are plotted against the Jiamusi and Khanka data (Fig. 11), which includes 498 ± 5 Ma ages from the Derba Massif (Nozhkin et al. Citation2004), 473 ± 3 Ma data from the Kioykin Massif (Donskaya et al. Citation2000), and 507 ± 8 to 498 ± 7 Ma from the Olhkon Massif (Gladkochub et al. Citation2008).
Recent investigations show growing evidence for widespread magmatism in the early Neoproterozoic at ca. 775 Ma (the Sarkhoi volcanics of the southeastern East Sayan; Gordienko et al. Citation2009) and the early Neoproterozoic magmatism at ca. 892–939 Ma (the Shaman metabasalts of the Baikal-Muya belt; Kuzmichev and Larionov, Citation2011, Citation2013) in the southwestern Altai-Sayan and Baikal regions (Fig. 11). Although ca. 895 Ma magmatic rocks have not been identified in the Khanka Massif, the results mentioned above clearly demonstrate that the Jiamusi and Khanka Massifs underwent an identical tectonic and magmatic evolution at least since ca. 755 Ma. In addition, palaeomagnetic data can also prove the reliability of the above conclusions. Yang et al. (Citation1998) suggested that several major micro-continental blocks in Northeast China were in southern latitudes <20° in early Palaeozoic times, like Siberia, North China, Tarim, Congo, and central Gondwana cratons (see also Neoproterozoic reconstructions based also on palaeomagnetism in Şengör et al. Citation2022). According to evidence from reliable geological, palaeomagnetic and palaeontological data, Zhao et al. (Citation2018) reconstructed the palaeogeographic location of the East Asian blocks after the break-up of the Rodinia and considered that the micro-continental blocks (including the Erguna, Xing’an, Songnen, and Jiamusi Massifs) in Northeast China are held between the Siberian Craton and Gondwana, and closer to the Siberian Craton. The discovery of the Lenan trilobite fossils (Proerbia sinensis sp.) from the Cambrian fauna of Siberia in the Songnen Massif also confirmed the affinity of the northern part of NE China microcontinental pieces with the Siberian Craton (Duan and An, Citation2001). Based on the palaeontological and palaeomagnetic data, the ca. 898–891 and 757–751 Ma magmatic periods and the ca. 500 Ma high-grade metamorphism in the Jiamusi–Khanka Massif provide constraints on the possible linkages between the Jiamusi–Khanka-Bureya Massif and the Siberian Craton during Neoproterozoic to early Palaeozoic times. The linkage could also apply for the Erguna Massif in the westward extension of the Jiamusi–Khanka Massif prior to the late Palaeozoic orocline formation. Furthermore, the evidence for magmatism and metamorphism at ca. 500 Ma in the Gorvan Bogd area could potentially be considered to constrain as further westward extension of this 500 Ma orogenic belt. In summary, the Jiamusi–Khanka-Bureya Massif cannot be directly derived from the North China, South China, and Tarim cratons. Instead, based on the arguments mentioned above, most of the study results reported from the Jiamusi–Khanka Massif (Wilde et al. Citation1997, Citation1999, Citation2000, Citation2003, Citation2010; Zhou et al. Citation2009, Citation2010a, Citation2018; Yang et al. Citation2020b, Citation2021a, Citation2021b) are like those in the CAOB and the Baikal belt along the southern margin of the Siberian Craton. In accordance with previous suggestions by Zhou et al. (Citation2011a, Citation2011b, Citation2018), we propose that the Jiamusi–Khanka Massif might be a rifted portion of the Baikal orogen and could be derived from the Siberian Craton. The detailed review confirms the current model as proposed before (Salnikova et al. Citation1998; Gladkochub et al. Citation2008; Zhou et al. Citation2010a). Based on the tectonic model of Gladkochub et al. (Citation2008) and Zhou et al. (Citation2011b), we propose a model whereby the Jiamusi/Khanka/Bureya massifs originated as part of the late Cambrian (~500 Ma) orogenic belt developed around the southern margin of the Siberian Craton (). The precursor rocks of the Olkhon, Kitoykin, Derba, and Slyudyanka massifs have a Siberian Craton (Aldan Domain) affinity (Salnikova et al. Citation1998; Gladkochub et al. Citation2008) and may have been detached from there in the latest Mesoproterozoic (Khain et al. Citation2002; Kuzmichev et al. Citation2005; Gladkochub et al. Citation2008). These detached fragments, possibly including the Jiamusi/Khanka/Bureya massifs, would have drifted to the south and west (in present-day coordinates) across the Palaeo-Asian Ocean to the southern part of the Siberian Craton (Angara–Anabar Province), where Neoproterozoic magmatism was the result of subduction (); Khain et al. Citation2003; Kuzmichev et al. Citation2005; Gladkochub et al. Citation2008). During the oblique collision with the SE part of the Angara–Anabar Province at about 500 Ma, the rocks were deformed and metamorphosed into granulite facies ()). The collision was accompanied by the emplacement of mantle-derived magmas with ages at 475–450 Ma (Gladkochub et al. Citation2008), which caused melting of the thickened lithosphere and intrusion of syn-tectonic granitoids. A > 1300 km long Cambrian khondalite belt in northern China located along the southern margin of the CAOB established by Zhou et al. (Citation2011b) further substantiate this speculative model that other massifs in the eastern CAOB (e.g. Songnen, Xing’an, Erguna massifs in NE China; see ) have had a similar history since they appear to record the same Cambrian events (e.g. Wilde et al. Citation1997, Citation1999, Citation2000, Citation2003, Citation2010; Zhou et al. Citation2009, Citation2010a, Citation2010b, Citation2010c, Citation2011a, Citation2011b).
However, the presence of relatively abundant inherited zircons (>1000 Ma) in rocks of the Heilongjiang Complex, when coupled with the E-MORB and OIB signature of the basalt protoliths from the Heilongjiang Complex (Zhou et al. Citation2009), proves that the combined Jiamusi/Khanka/Bureya massifs rifted away from Siberia at some time between 450 and 300 Ma, and moved farther south into what is now NE China/Far East Russia (); Zhou et al. Citation2010a; Li et al. Citation2020).
6. Conclusions
(1) The Jiamusi, Khanka, and Bureya Massifs should be regarded as parts of a contiguous late Neoproterozoic block. The basement of the Jiamusi–Khanka Massif consists of the Mashan Complex (with peak metamorphic ages of 530–500 Ma), comprising the Neoproterozoic orthogneisses (898–891 and 757–751 Ma) and deformed early Palaeozoic granitoids (530–484 Ma).
(2) The khondalite series in the Mashan Complex is characterized by graphite-bearing, Al-rich, and phosphorus-bearing rocks, and is mainly composed of Al-rich metapelitic, felsic, and calc-silicate rocks. These rocks of a Neoproterozoic passive continental margin have been intruded by syn- (530–500 Ma) and post-tectonic (490–476 Ma) granitic rocks. The sedimentary protoliths were deposited during the early Neoproterozoic and contain Palaeoproterozoic detrital sources.
(3) Two metamorphic zones are recognized in the Mashan Complex: the granulite and amphibolite facies belts. The available data suggest that the Mashan Complex is characterized by a clockwise orogen style P–T path with peak conditions of 850–870°C/8–9 kbar. It reveals vital information related to collisional orogeny, subsequent exhumation, and post-collision processes. Peak metamorphic conditions are explained by slab break-off during plate collision.
(4) The Jiamusi–Khanka-Bureya Massif cannot be directly derived from the North China, South China, and Tarim cratons. Instead, it might be a rifted portion of the Altai-Sayan and Baikal orogens and are derived from the outboard part of the Siberian Craton.
Highlights
Mashan Complex (Jiamusi–Khanka Massif) includes Neoproterozoic and early Palaeozoic granitoids.
The protolith of the Mashan Complex was deposited during the early Neoproterozoic.
The Mashan Complex is characterized by a clockwise orogen-style P–T path at 850–870°C/8–9 kbar.
The Jiamusi–Khanka Massif might be rifted from the Sayan–Baikal orogen of the Siberian Craton.
Mineral abbreviations
Amp-amphibole, Bi-biotite, Cpx-clinopyroxene, Crd-cordierite, Grt-garnet, Ilm-ilmenite, Kfs-K-feldspar, Opx-orthopyroxene, Pl-plagioclase, Qz-quartz, Sil-sillimanite, Spl-spinel.
Supplemental Material
Download Zip (52.1 KB)Acknowledgments
We gratefully acknowledge critical reviews and thoughtful suggestions by Editor in Chief Prof. Robert J. Stern and two anonymous reviewers to earlier versions of the manuscript. This study was financially supported by the National Natural Science Foundation of China (No. 41872192, 42172213, 41730210, and 41972215), Graduate Innovation Fund of Jilin University (No. 101832020CX208), and the China Scholarship Council (No. 202006170184). We are grateful to Xiao Zhou, Erlin Zhu, Xiaolei Sun, Dongxue Li, Shiju Jia, Zhiwei Song, and Shuyue Qu of College of Earth Sciences, Jilin University, for their advice and assistance that helped to improve the manuscript.
Disclosure statement
We declare that we do not have any commercial or associative interest that represents a conflict of interest in connection with the manuscript submitted.
Supplementary material
Supplemental data for this article can be accessed online at https://doi.org/10.1080/00206814.2022.2084646
Additional information
Funding
References
- Bi, J.H., Ge, W.C., Yang, H., Zhao, G.C., Yu, J.J., Zhang, Y.L., Wang, Z.H., and Tian, D.X., 2014, Petrogenesis and tectonic implications of early Paleozoic granitic magmatism in the Jiamusi Massif, NE China: Geochronological, geochemical and Hf isotopic evidence: Journal of Asian Earth Sciences, v. 96, p. 308–331. doi: 10.1016/j.jseaes.2014.09.013
- Bi, J.H., 2015, Petrogenesis and tectonic implication of early Paleozoic granites in the eastern Jiamusi Massif, [ Master Thesis]: Changchun, Jilin University. [in Chinese with English abstract.]
- Bi, J.H., Ge, W.C., Yang, H., Zhao, G.C., Xu, W.L., and Wang, Z.H., 2015, Geochronology, geochemistry and zircon Hf isotopes of the Dongfanghong gabbroic complex at the eastern margin of the Jiamusi Massif, NE China: Petrogenesis and Tectonic Implications: Lithos, v. 234–235, p. 27–46. doi: 10.1016/j.lithos.2015.07.015
- Bi, J.H., Ge, W.C., Yang, H., Wang, Z.H., Xu, W.L., Yang, J.H., Xing, D.H., and Chen, H.J., 2016, Geochronology and geochemistry of late Carboniferous-middle Permian I- and A-type granites and gabbro-diorites in the eastern Jiamusi Massif, NE China: Implications for petrogenesis and tectonic setting: Lithos, v. 266–267, p. 213–232. doi: 10.1016/j.lithos.2016.10.001
- Bi, J.H., Ge, W.C., Yang, H., Wang, Z.H., Dong, Y., Liu, X.W., and Ji, Z., 2017, Age, petrogenesis, and tectonic setting of the Permian bimodal volcanic rocks in the eastern Jiamusi Massif, NE China: Journal of Asian Earth Sciences, v. 134, p. 160–175. doi: 10.1016/j.jseaes.2016.09.022
- Brown, M., 1993, P–T–t evolution of orogenic belts and the causes of regional metamorphism: Journal of the Geological Society of London, v. 150, no. 2, p. 227–241. doi: 10.1144/gsjgs.150.2.0227.
- Cao, R., Zhao, W., and Xiao, Z., 1983, Precambrian classification and correlation in China: Beijing, Science Press, p. 421–451.
- Cao, X., Dang, Z.X., Zhang, X.Z., Jiang, J.S., and Wang, H.D., 1992, Jiamusi Composite Terranes: Changchun, Jilin Publishing House of Science and Technology, p. 224. [in Chinese with English and Russian abstracts.]
- Cawood, P.A., Van Gool, J.A.M., and Dunning, G.R., 1995, Collisional tectonics along the Laurentian margin of the Newfoundland Appalachians: Geological association of Canada: Special Paper, p. 41, 283–301.
- Chen, M., Sun, M., Cai, K.D., Buslov, M.M., Zhao, G.C., and Rubanova, E.S., 2014, Geochemical study of the Cambrian-Ordovician meta-sedimentary rocks from the northern Altai-Mongolian terrane, northwestern Central Asian orogenic belt: Implications on the provenance and tectonic setting: Journal of Asian Earth Sciences, v. 96, p. 69–83. doi: 10.1016/j.jseaes.2014.08.028
- Chen, M., Sun, M., Buslov, M.M., Cai, K.D., Zhao, G.C., Zheng, J., Rubanova, E.S., and Voytishek, E.E., 2015a, Neoproterozoic-middle Paleozoic tectono-magmatic evolution of the Gorny Altai terrane, northwest of the Central Asian orogenic belt: Constraints from detrital zircon U–Pb and Hf-isotope studies: Lithos, v. 233, p. 223–236. doi: 10.1016/j.lithos.2015.03.020
- Chen, M., Sun, M., Cai, K.D., Buslov, M.M., Zhao, G.C., Rubanova, E.S., and Voytishek, E.E., 2015b, Detrital zircon record of the early Paleozoic meta-sedimentary rocks in Russian Altai: Implications on their provenance and the tectonic nature of the Altai–Mongolian terrane: Lithos, v. 233, p. 209–222. doi: 10.1016/j.lithos.2014.11.023
- Cogné, J.P., Kravchinsky, V.A., Halim, N., and Hankard, F., 2005, Late Jurassic-Early Cretaceous closure of the Mongol-Okhotsk Ocean demonstrated by new Mesozoic palaeomagnetic results from the Trans-Baikal area (SE Siberia): Geophysical Journal International, v. 163, no. 2, p. 813–832. doi: 10.1111/j.1365-246X.2005.02782.x
- Condie, K.C., Boryta, M.D., Liu, J.Z., and Qian, X.L., 1992, The origin of khondalites: Geochemical evidence from the Archean to early Proterozoic granulite belt in the North China craton: Precambrian Research, v. 59, no. 3–4, p. 207–223. doi: 10.1016/0301-9268(92)90057-u
- Dang, Z.X., Wang, D.H., and Cui, G., 1988, The metamorphic zone, metamorphic facies and metamorphic series of the Heilongjiang and mashan groups: Beijing, Geological Publishing House, 23–31 p. [in Chinese with English abstract].
- Dang, Y.S., and Li, D.R., 1993, Discussion on isotope geochronology of Precambrian Jiamusi block: Journal of Changchun University Earth Sciences, v. 23, p. 312–318. [in Chinese with English abstract.]
- Degtyarev, K., Yakubchuk, A., Tretyakov, A., Kotov, A., and Kovach, V., 2017, Precambrian geology of the Kazakh Uplands and Tien Shan: An overview: Gondwana Research, v. 47, p. 44–75. doi: 10.1016/j.gr.2016.12.014
- Demoux, A., 2009, Neoproterozoic to Middle Palaeozoic Evolution of the Central Asian Orogenic Belt in South-Central Mongolia: Chronological and Geochemical Perspectives, [ PhD thesis]: Johannes Gutenberg-Universität Mainz, 162 p. doi: 10.25358/openscience-4514.
- Demoux, A., Kröner, A., Liu, D.Y., Badarch, G., 2009, Precambrian crystalline basement in southern Mongolia as revealed by SHRIMP zircon dating. International Journal of Earth Sciences, v. 98, no. 6, p. 1365–1380. doi: 10.1007/s00531-008-0321-4
- Dewey, J.F., Ryan, P.D., and Anderson, T.B., 1993, Orogenic uplift and collapse, crustal thickness, fabrics and metamorphic phase changes: The role of eclogites: London, Geological Society of London Special Publication, p. 325–343. doi: 10.1144/gsl.sp.1993.076.01.16
- Dong, C., and Zhou, J.B., 2012, Geochemistry of ~500Ma Pan-African khondalite series in west of NE China and its tectonic implications: Acta Petrologica Sinica, v. 28, p. 2866–2877. [in Chinese with English abstract.]
- Donskaya, T.V., Sklyarov, E.V., and Gladkochub, D.P., 2000, The Baikal collisional metamorphic belt: Doklady Earth Sciences, v. 374, p. 1075–1079.
- Duan, J.Y., and An, S.L., 2001, Early Cambrian Siberian fauna from Yichun of Heilongjiang province: Acta Palaeontologica Sinica, v. 40, p. 362–370. [in Chinese with English abstract.]
- Eizenhöfer, P.R., Zhao, G.C., Zhang, J., and Sun, M., 2014, Final closure of the Paleo-Asian Ocean along the Solonker suture zone: Constraints from geochronological and geochemical data of Permian volcanic and sedimentary rocks: Tectonics, v. 33, no. 4, p. 441–463. doi: 10.1002/2013TC003357
- Eizenhöfer, P.R., Zhao, G.C., Zhang, J., Han, Y.G., Hou, W.Z., Liu, D.X., and Wang, B., 2015a, Geochemical characteristics of the Permian basins and their provenances across the Solonker suture zone: Assessment of net crustal growth during the closure of the Palaeo-Asian Ocean: Lithos, v. 224, p. 240–255. doi: 10.1016/j.lithos.2015.03.012
- Eizenhöfer, P.R., Zhao, G.C., Sun, M., Zhang, J., Han, Y.G., and Hou, H., 2015b, Geochronological and Hf isotopic variability of detrital zircons in Palaeozoic sedimentary strata across the accretionary collision zone between the North China Craton and the Mongolian arcs, and its tectonic implications: Geological Society of America Bulletin. v. 127, no. 9–10, p. 1422–1436, doi:10.1130/B31175.1.
- England, P.C., and Thompson, A.B., 1984, Pressure-temperature-time paths of regional metamorphism I. heat transfer during the evolution of regions of thickened continental crust: Journal of Petrology, v. 25, p. 894–928. doi: 10.1093/petrology/25.4.894.
- Feng, Z.Q., Liu, Y.J., Liu, B.Q., Wen, Q.B., Li, W.M., and Liu, Q., 2016, Timing and nature of the Xinlin–Xiguitu ocean: Constraints from ophiolitic gabbros in the northern great Xing’an Range, eastern Central Asian orogenic belt: International Journal of Earth Sciences, v. 105, p. 491–505. doi: 10.1007/s00531-015-1185-z 2
- Feng, Z.Q., Liu, Y.J., Li, L., She, H.Q., Jiang, L.W., Du, B.Y., Liu, Y.W., Li, W.M., Wen, Q.B., and Liang, C.Y., 2018, Subduction, accretion, and collision during the neoproterozoic-Cambrian orogeny in the great Xing’an Range, NE China: Insights from geochemistry and geochronology of the Ali River ophiolitic mélange and arc-type granodiorites: Precambrian Research, v. 311, p. 117–135. doi: 10.1016/j.precamres.2018.04.013
- Furnes, H., and Safonova, I., 2019, Ophiolites of the central Asian orogenic belt: Geochemical and petrological characterization and tectonic settings: Geoscience Frontiers, v. 10, p. 1255–1284. doi: 10.1016/j.gsf.2018.12.007.
- Ge, W.C., Wu, F.Y., Zhou, C.Y., Abdel Rahman, A.A., 2005, Emplacement age of the Tahe granite and its constraints on the tectonic nature of the Ergun block in the northern part of the Da Hinggan Range: Chinese Science Bulletin, v. 50 , p. 1239–1247. [in Chinese with English abstract.]
- Ge, W.C., Chen, J.S., Yang, H., Zhao, G.C., Zhang, Y.L., and Tian, D.X., 2015, Tectonic implications of new zircon U–Pb ages for the Xinghuadukou Complex, Erguna Massif, northern great Xing’an range, NE China: Journal of Asian Earth Sciences, v. 106, p. 169–185. doi: 10.1016/j.jseaes.2015.03.011.
- Ge, M.H., Li, Z., Li, L., Zhang, J.J., and Liu, K., 2021a, Zircon and monazite U-Pb ages of the Mashan complex of the Jiamusi block of NE China: A link to Gondwana?: International Geology Review, v. 2021, p. 1–16. doi: 10.1080/00206814.2021.1940321
- Ge, M.H., Li, L., Wang, T., Zhang, J.J., Tong, Y., Guo, L., Liu, K., Feng, L., Song, P., and Yuan, J.G., 2021b, Hf isotopic mapping of the Paleozoic-Mesozoic granitoids from the Jiamusi and Songnen blocks, NE China: Implications for their tectonic division and juvenile continental crustal growth: Lithos, v. 386. doi: 10.1016/j.lithos.2021.106048
- Geng, Y. S., Shen, Q.H., Liu, F.L., Zhang, L.F., Dong, Y.S., Wang, H.C., Yang, C.H., Zhang, J.X., Wei, C.J., Xue, H.M., Zhou, X.W., and Ren, L.D., 2016, Regional metamorphic rocks and metamorphic evolution in China: Beijing, Geological Publishing House, p. 84–90. [in Chinese with English abstract.]
- Gladkochub, D.P., Donskaya, T.V., Mazukabzov, A.M., Stanevich, A.M., Sklyarov, E.V., and Ponomarchuk, V.A., 2007, Signature of Precambrian extension events in the southern Siberian craton: Russian Geology and Geophysics, v. 48, p. 17–31. doi: 10.1016/j.rgg.2006.12.001.
- Gladkochub, D.P., Donskaya, T.V., Wingate, M.T.D., Poller, U., Kröner, A., Fedorovsky, V.S., Mazukabov, A.M., Todt, W., and Pisarevsky, S.A., 2008, Petrology, geochronology, and tectonic implications of c . 500 Ma metamorphic and igneous rocks along the northern margin of the Central Asian Orogen (Olkhon terrane, Lake Baikal, Siberia): Journal of the Geological Society of London, v. 165, p. 235–246. doi:10.1144/0016-76492006-125
- Gladkochub, D.P., Donskaya, T.V., Wingate, M.T.D., Mazukabzov, A.M., Pisarevsky, S.A., Sklyarov, E.V., and Stanevich, A.M., 2010, A one-billion-year gap in the Precambrian history of the southern Siberian Craton and the problem of the Transproterozoic supercontinent: American Journal of Science, v. 310, p. 812–825. doi:10.2475/09.2010.03.
- Gordienko, I.V., Bulgatov, A.N., Lastochkin, N.I., and Sitnikova, V.S., 2009, Composition and U-Pb isotopic age determinations (SHRIMP II) of the ophiolitic assemblage from the Shaman paleospreading zone and the conditions of its formation (North Transbaikalia): Doklady Earth Sciences, v. 429, p. 1420–1425. doi:10.1134/S1028334X09090025
- Gou, J.,Sun, D.Y., Ren, Y.S., Liu, Y.J., Zhang, S.Y., Fu, C.L., Wang, T.H., Wu, P.F. and Liu, X.M. 2013, Petrogenesis and geodynamic setting of Neoproterozoic and Late Paleozoic magmatism in the Manzhouli–Erguna area of Inner Mongolia, China: Geochronological, geochemical and Hf isotopic evidence: Journal of Asian Earth Sciences, v. 67-68, p. 114–137. doi: 10.1016/j.jseaes.2013.02.016.
- Gou, J., Sun, D.Y., Deng, C.Z., Feng, Z., and Tang, Z.Y., 2020, Petrogenesis of the Neoproterozoic Xinlin ophiolite, northern great Xing’an Range, northeastern China: Implications for the evolution of the northeastern branch of the Paleo-Asian ocean: Precambrian Research, v. 350. doi:10.1016/j.precamres.2020.105925.
- Guo, X.Z., Takasu, A., Liu, Y. J., and Li, W.M., 2014, Zn-rich spinel in association with quartz in the Al-rich metapelites from the mashan khondalite series, NE China: Journal of Earth Science, v. 25, p. 207–223. doi:10.1007/s12583-014-0428-4.
- Han, G.Q., Liu, Y.J., Neubauer, F., Bartel, E., Genser, J., Feng, Z.Q., Zhang, L., and Yang, M.C., 2015, U–Pb age and Hf isotopic data of detrital zircons from the Devonian and Carboniferous sandstones in Yimin area, NE China: New evidence to the collision timing between the Xing’an and Erguna blocks in eastern segment of Central Asian orogenic belt: Journal of Asian Earth Sciences, v. 97, p. 211–228. doi:10.1016/j.jseaes.2014.08.006.
- Han, Y.G., Zhao, G.C., Sun, M., Eizenhöfer, P.R., Hou, W.Z., Zhang, X.R., Liu, D.X., Wang, B., and Zhang, G.W., 2015, Paleozoic accretionary orogenesis in the Paleo-Asian ocean: Insights from detrital zircons from Silurian to Carboniferous strata at the northwestern margin of the Tarim Craton: Tectonics, v. 34, p. 334–351. doi:10.1002/2014TC003668.
- Han, Y.G., Zhao, G.C., Sun, M., Eizenhöfer, P.R., Hou, W.Z., Zhang, X.R., Liu, Q., Wang, B., Liu, D.X., and Xu, B., 2016a, Late Paleozoic subduction and collision processes during the amalgamation of the central Asian orogenic belt along the South Tianshan suture zone: Lithos, v. 246–247, p. 1–12. doi: 10.1016/j.lithos.2015.12.016
- Han, Y.G., Zhao, G.C., Cawood, P.A., Sun, M., Eizenhöfer, P.R., Hou, W.Z., Zhang, X.R., and Liu, Q., 2016b, Tarim and North China cratons linked to northern Gondwana through switching accretionary tectonics and collisional orogenesis: Geology, v. 44, p. 95–98. doi:10.1130/G37399.1.
- HBGMR (Heilongjiang Bureau of Geology and Mineral Resources), 1993, Regional geology of Heilongjiang province: Beijing, Geological Publishing House, p. 1–734. [in Chinese with English abstract.]
- Hou, W.Z., Zhao, G.C., Han, Y.G., Eizenhoefer, P.R., Zhang, X.R., and Liu, Q., 2020, A ~2.5 Ga magmatic arc in NE China: New geochronological and geochemical evidence from the Xinghuadukou Complex: Geological Journal, v. 55, p. 2550–2571. doi:10.1002/gj.3513.
- Huang, Y.C., Zhang, X.Z., Zhang, H.B., Xiong, X.S., Liu, C.L., and Zhao, L.L., 2009, Geochemical characteristics and sedimentation age of the Majiajie Group in eastern Heilongjiang Province, China: Acta Geologica Sinica, v. 83, p. 295–303. [in Chinese with English abstract.]
- Jahn, B.M., Wu, F.Y., and Chen, B., 2000 Massive granitoid generation in Central Asia: Nd isotopic evidence and implication for continental growth in the Phanerozoic: Episodes, v. 23, p. 82–92. doi:10.18814/epiiugs/2000/v23i2/001.
- Jiang, J.S., 1992, Peak regional metamorphism of the khondalite series of Mashan group and its evolution: Acta Petrologica et Mineralogica, v. 11, p. 97–108. [in Chinese with English abstract.]
- Jiang, J.S., 1993, Geochemistry of Mashan-Group khondalite series: Geochimica, v. 12, p. 363–372. [in Chinese with English abstract.]
- Khain, E.V., Bibikova, E.V., Kröner, A., Zhuravlev, D.Z., Sklyarov, E.V., Fedotova, A.A., and Kravchenko-Berezhnoy, I.R., 2002, The most ancient ophiolite of the central asian fold belt: U–Pb and Pb–Pb zircon ages for the Dunzhugur Complex, Eastern Sayan, Siberia, and geodynamic implications: Earth and Planetary Science Letters, v. 199, p. 311–325. doi: 10.1016/S0012-821X(02)00587-3.
- Khain, E.V., Bibikova, E.V., Salnikova, E.B., Kröner, A., Gibsher, A.S., Didenko, A.N., Degtyarev, K.E., and Fedotova, A.A., 2003, The Palaeo-Asian Ocean in the Neoproterozoic and early Palaeozoic: New geochronologic data and palaeotectonic reconstructions: Precambrian Research, v. 122, p. 329–358. doi: 10.1016/S0301-9268(02)00218-8.
- Khanchuk, A.I., Vovna, G.M., Kiselev, V.I., Mishkin, M.A., and Lavrik, S.N., 2010a, First results of zircon LA-ICP-MC U-Pb dating of the rocks from the granulite complex of Khanka Massif in the Primorye region: Doklady Earth Sciences, v. 434, no. 1, p. 1164–1167. doi: 10.1134/S1028334X10090059.
- Khanchuk, A.I., Sakhno, V.G., and Alenicheva, A.A., 2010b, First SHRIMP U–Pb zircon dating of magmatic complexes in the southwestern Primor’e region: Doklady Earth Sciences, v. 431, no. 2, p. 424–428. doi: 10.1134/S1028334X10040033.
- Kovalenko, V.I., Yarmolyuk, V.V., Kovach, V.P., Kotov, A.B., Kozakov, I.K., Salnikova, E.B., and Larin, A.M., 2004, Isotope provinces, mechanisms of generation and sources of the continental crust in the Central Asian mobile belt: Geological and isotopic evidence: Journal of Asian Earth Science, v. 23, p. 605–627. doi: 10.1016/S1367-9120(03)00130-5.
- Kröner, A., Lehmann, J., Schulmann, K., Demoux, A., Lexa, O., Tomurhuu, D., Stípská, P., Liu, D., and Wingate, M.T.D., 2010, Lithostratigraphic and geochronological constraints on the evolution of the Central Asian Orogenic Belt in SW Mongolia: Early Paleozoic rifting followed by late Paleozoic accretion. American Journal of Science 310, 523–574. doi: 10.2475/07.2010.01.
- Kröner, A., Kovach, V., Belousova, E., Hegner, E., Armstrong, R., Dolgopolova, A., Seltmann, R., Alexeiev, D.V., Hoffmann, J.E., Wong, J., Sun, M., Cai, K., Wang, T., Tong, Y., Wilde, S.A., Degtyarev, K.E., and Rytsk, E.Y., 2014, Reassessment of continental growth during the accretionary history of the central asian orogenic belt: Gondwana Research, v. 25, p. 103–125. doi: 10.1016/j.gr.2012.12.023.
- Kröner, A., Kovach, V., Kozakov, I. K., Kirnozova, T., Azimov, P., Wong, J., and Geng, H.Y., 2015, Zircon ages and Nd–Hf isotopes in UHT granulites of the Ider complex: A cratonic terrane within the central Asian orogenic belt in NW Mongolia. Gondwana Research, v. 27, p. 1392–1406. doi: 10.1016/j.gr.2014.03.005.
- Kröner, A., Kovach, V., Alexeiev, D., Wang, K.-L., Wong, J., Degtyarev, K., and Kozakov, I., 2017, No excessive crustal growth in the central asian orogenic belt: Further evidence from field relationships and isotopic data: Gondwana Research, v., 50, p. 135–166. doi: 10.1016/j.gr.2017.04.006.
- Kuzmichev, A., Kröner, A., Hegner, E., Liu, D.Y., and Wan, Y.S., 2005, The Shishkhid ophiolite, northern Mongolia: A key to the reconstruction of a Neoproterozoic island-arc system in Central Asia: Precambrian Research, v. 138, p. 125–150. doi: 10.1016/j.precamres.2005.04.002.
- Kuzmichev, A.B., and Larionov, A.N., 2011, The Sarkhoi group in east Sayan: Neoproterozoic (~770–800 Ma) volcanic belt of the Andean type: Russian Geology and Geophysics, v. 52, p. 685–700. doi: 10.1016/j.rgg.2011.06.001 7
- Kuzmichev, A.B., and Larionov, A.N., 2013, Neoproterozoic island arcs in East Sayan: Duration of magmatism (from U–Pb zircon dating of volcanic clastics): Russian Geology and Geophysics, v. 54, p. 34–43. doi: 10.1016/j.rgg.2012.12.003.
- Lennon, R.G., 1997, The metamorphic conditions, tectonic setting and evolution of the Mashan Group, Heilongjiang Province, Northeastern China: Perth, Curtin University of Technology, 150 p. [ Honours Thesis (unpublished)]
- Levashova, N.M., Kalugin, V.M., Gibsher, A.S., Yff, J., Ryabinin, A.B., Meert, J.G., and Malone, S.J., 2010, The origin of the Baydaric Microcontinent, Mongolia: Constraints from paleomagnetism and geochronology: Tectonophysics, v. 485, p. 306–320. doi: 10.1016/j.tecto.2010.01.012.
- Levashova, N.M., Meert, J.G., Gibsher, A.S., Grice, W.C., and Bazhenov, M.L., 2011, The origin of microcontinents in the central asian orogenic belt: Constraints from paleomagnetism and geochronology: Precambrian Research, v. 185, p. 37–54. doi: 10.1016/j.precamres.2010.12.001.
- Li, J.Y., Niu, B.G., Song, B., Xu, W.X., Zhang, Y.H., and Zhao, Z.R., 1999, Crustal Formation and Evolution of Northern Changbai Mountains: Northeast China: Beijing, Geological Publishing House, p. 32–50. [in Chinese with English abstract.]
- Li, J.Y., 2006, Permian geodynamic setting of northeast China and adjacent regions: Closure of the Paleo-Asian Ocean and subduction of the Paleo-Pacific plate: Journal of Asian Earth Sciences, v. 26, p. 207–224. doi: 10.1016/j.jseaes.2005.09.001.
- Li, W.M., Takasu, A., Liu, Y.J., Genser, J., Neubauer, F., and Guo, X.Z., 2011, U-Pb and 40Ar/39Ar age constrains on protolith and high-P/T type metamorphism of the Heilongjiang Complex in the Jiamusi Massif, NE China: Journal of Mineralogical and Petrological Sciences, v. 106, p. 326–331. doi: 10.2465/jmps.110621e
- Li, W.M., Liu, Y.J., Takasu, A., Zhao, Y.L., Wen, Q.B., Guo, X.Z., and Zhang, L., 2014, Pressure (P)-temperature (T)-time (t) paths of the blueschists from the Yilan area, Heilongjiang province: Acta Petrologica Sinica, v. 30, p. 3085–3099. [in Chinese with English abstract.]
- Li, S.Z., Yang, Z., Zhao, S.J., Li, X.Y., Guo, L.L., Yu, S., Liu, X., Suo, Y.H., and Lan, H.Y., 2016, Global Early Paleozoic orogens (I): Collision-type orogeny: Journal of Jilin University (Earth Science Edition), v. 46, no. 4, p. 1–24. [in Chinese with English abstract.]
- Li, W.M., Liu, Y.J., Zhao, Y.L., Feng, Z.Q., Zhou, J.P., Wen, Q.B., Liang, C.Y., and Zhang, D., 2020, Tectonic evolution of the Jiamusi block, NE China: Acta Petrologica Sinica, v. 36, p. 665–684. doi: 10.18654/1000-0569/2020.03.03.
- Liu, J.L., 1988, Study on the Precambrian of the Jiamusi block: Journal of the Changchun College of Geology, v. 18, no. 2, p. 147–156. [in Chinese with English abstract.]
- Liu, Y.J., Li, W.M., Feng, Z.Q., Wen, Q.B., Neubauer, F., and Liang, C.Y., 2017, A review of the Paleozoic tectonics in the eastern part of central asian orogenic belt: Gondwana Research, v. 43, p. 123–148. doi: 10.1016/j.gr.2016.03.013.
- Liu, Y.J., Li, W.M., Ma, Y.F., Feng, Z.Q., Guan, Q.B., Li, S.Z., Chen, Z.X., Liang, C.Y., and Wen, Q.B., 2021, An orocline in the eastern Central Asian Orogenic Belt: Earth-Science Reviews, v. 221. doi: 10.1016/j.earscirev.2021.103808.
- Lu, L.Z., Xu, X.C., and Liu, F.L., 1996, The Precambrian khondalite series of northern: China: Changchun, Changchun Publishing House. [in Chinese.]
- Luan, J.P., Wang, F., Xu, W.L., Ge, W.C., Sorokin, A.A., Wang, Z.W., and Guo, P., 2017, Provenance, age, and tectonic implications of Neoproterozoic strata in the Jiamusi Massif: Evidence from U–Pb ages and Hf isotope compositions of detrital and magmatic zircons: Precambrian Research, v. 297, p. 19–32. doi: 10.1016/j.precamres.2017.05.012.
- Ma XH, Zhu WP, Zhou ZH, and Qiao SL (2016) Transformation from Paleo-Asian Ocean closure to Paleo-Pacific subduction: New constraints from granitoids in the eastern Jilin-Heilongjiang Belt, NE China. Journal of Asian Earth Sciences 144: 261–286. doi: 10.1016/j.jseaes.2016.11.003.
- Meng, E., Xu, W.L., Pei, F.P., Yang, D.B., Ji, W.Q., Yu, Y., and Zhang, X.Z., 2008, Chronology of Late Paleozoic volcanism in eastern and southeastern margin of Jiamusi Massif and its tectonic implications: Chinese Science Bulletin, v. 53, p. 1231–1245. [in Chinese with English abstract.]
- Meng, E., Xu, W.L., Pei, F.P., Yang, D.B., Yu, Y., and Zhang, X.Z., 2010, Detrital zircon geochronology of Late Paleozoic sedimentary rocks in eastern Heilongjiang province, NE China: Implications for the tectonic evolution of the eastern segment of the central asian orogenic belt: Tectonophysics, v. 485, p. 42–51. doi: 10.1016/j.tecto.2009.11.015.
- Miao, L.C., Zhu, M.S., Liu, C.H., Baatar, M.S., Anaad, C.S., Yang, S.H., and Li, X.B., 2020, Detrital-Zircon Age spectra of neoproterozoic-Paleozoic sedimentary rocks from the Ereendavaa terrane in NE Mongolia: Implications for the early-stage evolution of the Ereendavaa terrane and the Mongol-Okhotsk Ocean: Minerals, v. 10, p. 742. doi: 10.3390/min10090742.
- Mongush, A.A., Lebedev, V.I., Travin, A.V., and Yarmolyuk, V.V., 2011, Ophiolites of western Tyva as fragments of a late vendian island arc of the Paleo-Asian Ocean: Doklady Earth Sciences, v. 438, p. 866–872. doi: 10.1134/S1028334X11060328.
- Nozhkin, A.D., Turkina, O.M., Bayanova, T.B., and Travin, A.V., 2004, The granitoids of the south-western frame of the Siberian craton as indicators of the Riphean juvenile crust forming and following accretion–collisional processes: Irkutsk, IGSBRAS, p. 49–52.
- Ovchinnikov, R.O., Sorokin, A.A., and Kudryashov, N.M., 2020, the first evidence of Cambrian granitoid magmatism during the formation history of the Bureya Continental Massif of the Central Asian fold belt: Doklady Earth Sciences, v. 493, p. 490–494. doi: 10.1134/s1028334x20070120.
- Ovchinnikov, R.O., Sorokin, A.A., and Kydryashov, N.M., 2021, Early Paleozoic magmatic events in the Bureya Continental Massif, central asian orogenic belt: Timing and tectonic significance: Lithos, v. 396. doi: 10.1016/j.lithos.2021.106237.
- Pfänder, J.A., Jochum, K.P., Galer, S.J.G., Hellebrand, E.W.G., Jung, S., and Kröner, A., 2021, Geochemistry of ultramafic and mafic rocks from the northern central asian orogenic belt (Tuva, Central Asia) - constraints on lower and middle arc crust formation linked to late Proterozoic intra-oceanic subduction: Precambrian Research, v. 356. doi: 10.1016/j.precamres.2020.106061.
- Platt, J.R., and England, P.C., 1994, Convective removal of lithosphere beneath mountain belts: Thermal and mechanical consequences: American Journal of Science, v. 294, p. 307–336. doi: 10.2475/ajs.294.3.307.
- Quan, J.Y., Chi, X.G., Zhang, R., Sun, W., Fan, L.F., and Hu, Z.C., 2013, LA-ICP-MS U–Pb geochronology of detrital zircon from the Neoproterozoic dongfengshan group in Songnen massif and its geological significance: Geological Bulletin of China, v. 32, p. 353–364. [in Chinese with English abstract.]
- Ren, L.D., Wang, Y.B., Yang, C.H., Han, J., Xie, H.Q., and Li, L.S., 2010, Metamorphism, migmatization and granites of the Mashan Complex in Heilongjiang Province, Northeast China: Acta Petrologica Sinica, v. 26, p. 2005–2014. [in Chinese with English abstract.]
- Ren, L.D., Wang, Y.B., Yang, C.H., Zhao, Z.R., Guo, J.J., and Gao, H.L., 2012, Two types of metamorphism and their relationships with granites in the Mashan complex: Acta Petrologica Sinica, v. 28, p. 2855–2865. [in Chinese with English abstract.]
- Rytsk, E.Y., Kovach, V.P., Yarmolyuk, V.V., Kovalenko, V.I., Bogomolov, E.S., and Kotov, A.B., 2011, Isotopic structure and evolution of the continental crust in the east transbaikalian segment of the central asian foldbelt: Geotektonika, v. 45, p. 17–51. doi: 10.1134/S0016852111050037.
- Rytsk, E.Y., Andreev, A.A., Tolmacheva, E.V., Kuznetsov, A.B., Velikoslavinsky, S.D., and Fedoseenko, A.M., 2021, Paleoproterozoic age of gneiss-granites of the Mamskaya zone (Baikal–Patom belt): Geological implications: Doklady Earth Sciences, v. 498, p. 367–371. doi: 10.1134/S1028334X21050159.
- Safonova, I.Y., Seltmann, R., Kroener, A., Gladkochub, D., Schulmann, K., Xiao, W., Kim, T., Komiya, T., and Sun, M., 2011, A new concept of continental construction in the central asian orogenic belt (compared to actualistic examples from the Western Pacific): Episodes, v. 34, p. 186–194. doi: 10.18814/epiiugs/2011/v34i3/005.
- Salnikova, E.B., Sergeev, S.A., Kotov, A.B., Yakovleva, S.Z., Reznitskii, L.Z., and Vasil’ev, E.P., 1998, U–Pb zircon dating of granulite metamorphism in the Slyudyanskiy complex, eastern Siberia: Gondwana Research, v. 1, p. 195–205. doi: 10.1016/S1342-937X(05)70830-3.
- Şengör, A.M.C., Natal’in, B.A., and Burtman, V.S., 1993, Evolution of the Altaid tectonic collage and Palaeozoic crustal growth in Eurasia: Nature, v. 364, p. 299–307. doi: 10.1038/364299a0.
- Şengör, A.M.C., and Natal’in, B.A., 1996, Palaeotectonics of Asia: Fragments of a synthesis. In Yin, A., Harrison, M. (Eds.), The Tectonic Evolution of Asia, Rubey Colloquium. Cambridge University Press, Cambridge, pp. 486–640.
- Şengör, A.M.C., Sunal, G., Natal’in, Boris A., and van der Voo, R., 2022, The altaids: A review of twenty-five years of knowledge accumulation: Earth-Science Reviews, v. 228, 104013. doi: 10.1016/j.earscirev.2022.104013.
- Sklyarov, E.V., Gladkochub, D.P., Mazukabzov, A.M., Menshagin, Y.V., Watanabe, T., and Pisarevsky, S.A., 2003, Neoproterozoic mafic dike swarms of the Sharyzhalgai metamorphic massif, southern Siberian craton: Precambrian Research, v. 122, p. 359–376. doi: 10.1016/S0301-9268(02)00219-X.
- Song, B., Niu, B.G., Li, J.Y., and Xu, W.X., 1994, Isotope geochronology of granitoids in Mudanjiang-Jixi area: Acta Petrologica et Mineralogica, v. 13, no. 3, p. 204–213. [in Chinese with English Abstract.]
- Sorokin, A.A., Smirnov, Y.V., Kotov, A.B., Sal’nikova, E.B., Sorokin, A.P., Kovach, V.P., Yakovleva, S.Z., Anisimova, I.V., 2014, Early Paleozoic age of the Isagachi sequence (Chalaya Group, Gonzha Terrane) in the eastern part of the Central Asian Fold Belt: Doklady Akademii Nauk, v. 457, p. 323–326.
- Sun, X.M., Liu, Y.J., Sun, Q.C., Han, G.Q., Wang, S.Q., and Wang, Y.D., 2008, 40Ar/39Ar geochronology evidence of strike-slip movement in Dunhua-Mishan fault zone: Journal of Jilin University (Earth Science Edition), v. 38, p. 965–972. [in Chinese with English abstract.]
- Sun, X.M., Wang, S.Q., Wang, Y.D., Du, J.Y., and Xu, Q.W., 2010, The structural feature and evolutionary series in the northern segment of Tancheng-Lujiang fault zone: Acta Petrologica Sinica, v. 26, p. 165–176. [in Chinese with English abstract.]
- Sun, L.X., Ren, B.F., Zhao, F.Q., and Peng, L.N., 2012, Zircon U–Pb ages and Hf isotope characteristics of Taipingchuan large porphyritic granite pluton of Erguna Massif in the Great Xing’an range: Earth Science Frontiers, v. 19, p. 114–122. [in Chinese with English abstract.]
- Sun, L.X., Ren, B.F., Zhao, F.Q., Ji, S.P., and Geng, J.Z., 2013, Late Paleoproterozoic magmatic records in the Eerguna massif: Evidence from the zircon U–Pb dating of granitic gneisses: Geological Bulletin of China, v. 32, p. 341–352. [in Chinese with English abstract.]
- Sun, M.D., 2013, Late Mesozoic magmatism and its tectonic implication for the Jiamusi Block and adjacent areas of NE China, [Ph. D. Dissertation]: Hangzhou: Zhejiang University. [in Chinese with English summary.]
- Sun, M.D., Xu, Y.G., Wilde, S.A., Chen, H.L., and Yang, S.F., 2015a, The Permian Dongfanghong island-arc gabbro of the Wandashan Orogen, NE China: Implications for Paleo-Pacific subduction: Tectonophysics, v. 659, p. 122–136. doi: 10.1016/j.tecto.2015.07.034.
- Sun, M.D., Xu, Y.G., Wilde, S.A., and Chen, H.L., 2015b, Provenance of Cretaceous trench slope sediments from the Mesozoic Wandashan Orogen, NE China: Implications for determining ancient drainage systems and tectonics of the Paleo-Pacific: Tectonics, v. 34, p. 1269–1289. doi: 10.1002/2015TC003870.
- Sun, C.Y., Xu, W.L., Cawood, P.A., Tang, J., Zhao, S., Li, Y., and Zhang, X.M., 2019, Crustal growth and reworking: A case study from the Erguna Massif, eastern Central Asian Orogenic Belt: Scientific Reports, v. 9, p. 17671. doi: 10.1038/s41598-019-54230-x.
- Tang, K.D., 1990, Tectonic development of Palaeozoic fold belts at the north margin of the Sino–Korean Craton: Tectonics, v. 9, p. 249–260. doi: 10.1029/TC009i002p00249.
- Tang, K.D., Wang, Y., He, G.Q., and Shao, J.A., 1995, Continental-margin structure of northeast China and its adjacent areas: Acta Geologica Sinica, v. 69, p. 16–30. [in Chinese with English abstract.]
- Tang, J., Xu, W.L., Wang, F., Wang, W., Xu, M.J., and Zhang, Y.H., 2013, Geochronology and geochemistry of Neoproterozoic magmatism in the Erguna Massif, NE China: Petrogenesis and implications for the breakup of the Rodinia supercontinent: Precambrian Research, v. 224, p. 597–611. doi: 10.1016/j.precamres.2012.10.019.
- Tang, G.J., Chung, S.L., Hawkesworth, C.J., Cawood, P.A., Wang, Q., Wyman, D.A., Xu, Y.G., and Zhao, Z.H., 2017, Short episodes of crust generation during protracted accretionary processes: Evidence from Central Asian Orogenic Belt, NW China: Earth and Planetary Science Letters, v. 464, p. 142–154. doi: 10.1016/j.epsl.2017.02.022.
- Wang, Y.Q., 1996, Pre-Cambrian stratigraphy of northeastern China: Jilin Geology, v. 15, p. 1–14. [in Chinese.]
- Wang, F., Xu, W.L., Meng, E., Cao, H.H., and Gao, F.H., 2012a, Early Paleozoic amalgamation of the Songnen-Zhangguangcai Range and Jiamusi massifs in the eastern segment of the central asian orogenic belt: Geochronological and geochemical evidence from granitoids and rhyolites: Journal of Asian Earth Sciences, v. 49, p. 234–248. doi: 10.1016/j.jseaes.2011.09.022.
- Wang F, Xu W L, Gao F H, Meng, E., Cao, H.H., Zhao, L., and Yang, Y., 2012b, Tectonic history of the Zhangguangcailing group in eastern Heilongjiang Province, NE China: Constraints from U-Pb geochronology of detrital and magmatic zircons: Tectonophysics, v. 566–567, p. 105–122. doi: 10.1016/j.tecto.2012.07.018.
- Wang, F., Xu, W.L., Gao, F.H., Zhang, H.H., Pei, F.P., Zhao, L., and Yang, Y., 2013, Precambrian terrane within the Songnen-Zhangguangcai Range Massif, NE China: Evidence from U–Pb ages of detrital zircons from the Dongfengshan and Tadong groups: Gondwana Research, v. 26, p. 402–413. doi:10.1016/j.gr.2013.06.017.
- Wang, F., Xu, W.L., Xu, Y.G., Gao, F.H., and Ge, W.C., 2015a, Late Triassic bimodal igneous rocks in eastern Heilongjiang province, NE China: Implications for the initiation of subduction of the paleo-pacific plate beneath Eurasia: Journal of Asian Earth Sciences, v. 97, p. 406–423. doi: 10.1016/j.jseaes.2014.05.025.
- Wang, Z.H., Ge, W.C., Yang, H., Zhang, Y.L., Bi, J.H., Tian, D.X., and Xu, W.L., 2015b, Middle Jurassic oceanic island igneous rocks of the Raohe accretionary complex, northeastern China: Petrogenesis and tectonic implications: Journal of Asian Earth Sciences, v. 111, p. 120–137. doi: 10.1016/j.jseaes.2015.08.005.
- Wang, Z.W., Xu, W.L., Pei, F.P., Wang, F., Guo, P., 2015b, Geochronology and geochemistry of early Paleozoic igneous rocks of the Lesser Xing’an range, NE China: Implications for the tectonic evolution of the eastern central asian orogenic belt: Lithos, v. 261, p. 144–163. doi:10.1016/j.lithos.2015.11.006.
- Wang, Z.H., Ge, W.C., Yang, H., Bi, J.H., Ji, Z., Dong, Y., and Xu, W.L., 2017, Petrogenesis and tectonic implications of Early Jurassic volcanic rocks of the Raohe accretionary complex: NE China: Journal of Asian Earth Sciences, v. 134, p. 262–280. doi: 10.1016/j.jseaes.2016.09.021.
- Wen, Q.B., Liu, Y.J., Li, W.M., Han, G.Q., and Ding, L., 2008, Monazite ages and its geological significance of granitoid gneiss in the Jiamusi Massif: Journal of Jilin University (Earth Science Edition), v. 38, p. 187–193. [in Chinese with English abstract.]
- Wilde, S.A., Dorsett-Bain, H.L., and Liu, J.L., 1997, The identification of a Late Pan-African granulite facies event in northeastern China: SHRIMP U–Pb zircon dating of the Mashan group at Liu, in Proceedings of the 30th IGC, Precambrian Geology and Metamorphic Petrology, Mao, Heilongjiang Province, China, p. 59–74.
- Wilde, S., Helen, L., Dorsett, B., and Lennon, R.G., 1999, Geological setting and controls on the development of graphite, sillimanite and phosphate mineralization within the Jiamusi Massif: An Exotic fragment of Gondwanaland located in North-Eastern China: Gondwana Research, v. 2, p. 21–46. doi: 10.1016/S1342-937X(05)70125-8.
- Wilde, S.A., Zhang, X.Z., and Wu, F.Y., 2000, Extension of a newly-identified 500 Ma metamorphic terrain in Northeast China: Further U–Pb SHRIMP dating of the Mashan complex, Heilongjiang Province, China: Tectonophysics, v. 328, p. 115–130. doi: 10.1016/S0040-1951(00)00180-3.
- Wilde, S.A., Wu, F.Y., and Zhang, X.Z., 2001, The Mashan Complex: SHRIMP U-Pb zircon evidence for a Late-African metamorphic event in NE China and its implication for global continental reconstruction: Geochemica, v. 30, no. 1, p. 35–50. [in Chinese with English abstract.]
- Wilde, S.A., Wu, F.Y., and Zhang, X.Z., 2003, Late Pan-African magmatism in northeastern China: SHRIMP U–Pb zircon evidence from granitoids in the Jiamusi Massif: Precambrian Research, v. 122, p. 311–327. doi: 10.1016/S0301-9268(02)00217-6.
- Wilde, S.A., Wu, F.Y., and Zhao, G.C., 2010, The Khanka block, NE China, and its significance to the evolution of the central asian orogenic belt and continental accretion: London, Geological Society of London Special Publication, v. 338, p. 117–137. doi: 10.1144/SP338.6.
- Windley, B.F., Alexeiev, D., Xiao, W.J., Kröner, A., and Badarch, G., 2007, Tectonic model for accretion of the central asian orogenic belt: London, Journal of Geological Society, v. 164, p. 31–47. doi: 10.1144/0016-76492006-022.
- Wu, F.Y., Sun, D.Y., Li, H.M., and Wang, X., 2000, Zircon U–Pb ages of the basement rocks beneath the Songliao Basin, NE China: Chinese Science Bulletin, v. 45, p. 656–660. [in Chinese.]
- Wu, F.Y., Wilde, S.A., and Sun, D.Y., 2001, Zircon SHRIMP U–Pb ages of gneissic granites in Jiamusi massif, northeastern China: Acta Petrologica Sinica, v. 17, p. 443–452. [in Chinese with English abstract].
- Wu, F.Y., Yang, J.H., Lo, C.H., Wilde, S.A., Sun, D.Y., and Jahn, B.M., 2007a, The Heilongjiang Group: A Jurassic accretionary complex in the Jiamusi Massif at the western pacific margin of northeastern: China: The Island Arc, v. 16, p. 156–172. doi: 10.1111/j.1440-1738.2007.00564.x.
- Wu, F.Y., Zhao, G.C., Sun, D.Y., Wilde, S.A., and Zhang, G.L., 2007b, The Hulan Group: Its role in the evolution of the central asian orogenic belt of NE China: Journal Asian Earth Science v. 30, p. 542–556. doi: 10.1016/j.jseaes.2007.01.003.
- Wu, F.Y., Sun, D.Y., Ge, W.C., Zhang, Y.B., Grant, M.L., Wilde, S.A., and Jahn, B.M., 2011, Geochronology of the Phanerozoic granitoids in northeastern China: Journal of Asian Earth Sciences, v. 41, p. 1–30. doi: 10.1016/j.jseaes.2010.11.014.
- Wu, X.W., Zhang, C., Zhang, Y.J., Guo, W., 2018, 2.7 Ga Monzogranite on the Songnen Massif and its geological implications: Acta Geologica Sinica (English Edition), v. 92, p. 1265–1266. doi: 10.1111/1755-6724.13609.
- Xiao, W.J., Windley, B.F., Badarch, G., Sun, S.L., Li, J.L., Qin, K.Z., and Wang, Z.H., 2004, Palaeozoic accretionary and convergent tectonics of the southern Altaids: Implications for the growth of Central Asia: Journal of the Geological Society, v. 161, p. 339–342. doi: 10.1144/0016-764903-165.
- Xie, H.Q., Miao, L.C., Chen, F.Q., Zhang, F.Q., and Liu, D.Y., 2008, Characteristics of the “Mashan Group” and zircon SHRIMP U–Pb dating of granite in Muling area, southeastern Heilongjiang Province, China: Constraint on crustal evolution of the southernmost of Jiamusi Massif: Geological Bulletin China, v. 27, p. 2127–2137. [in Chinese with English abstract.]
- Xin, Z.H., Han, J.T., Liu, C., Liu, L.J., Tian, Y., Yang, B.J., Li, Z.Y., and Mu, Q., 2021, Lithospheric structure and transformation of the Songnen and Jiamusi blocks in the eastern segment of the Central asian orogenic belt revealed by 3D magnetotelluric inversion: Tectonophysics, v. 816. doi: 10.1016/j.tecto.2021.229015.
- Xiong, S., Xu, W.L., Wang, F., and Ge, W.C., 2020, Formation age and provenance of the Iman Group in the Khanka Massif: Evidence from U-Pb geochronology of detrital and magmatic zircons: Acta Petrologica Sinica, v. 36, p. 741–758. doi: 10.18654/1000-0569/2020.03.07.
- Xu, X., Harbert, W., Drill, S., and Kravchinsky, V., 1997, New paleomagnetic data from the Mongol-Okhotsk collision zone, Chita region, south central Russia: Implications for Paleozoic paleogeography of the Mongol-Okhotsk ocean: Tectonophysics, v. 269, p. 113–129. doi: 10.1016/S0040-1951(96)00140-0.
- Xu, W.L., Pei, F.P., Wang, F., Meng, E., Ji, W.Q., Yang, D.B., and Wang, W., 2013, Spatial-temporal relationships of Mesozoic volcanic rocks in NE China: Constraints on tectonic overprinting and transformations between multiple tectonic regimes: Journal of Asian Earth Sciences, v. 74, p. 167–193. doi: 10.1016/j.jseaes.2013.04.003.
- Xu, J.L., Zheng, C.Q., Tajčmanová, L., Zhong, X., Han, X.M., Wang, Z.Y., and Yang, Y., 2018, Phase equilibria modeling and zircon dating for Precambrian metapelites from the xinghuadukou complex in the Lulin forest of the Erguna Massif, Northeast China: Journal of Earth Science, v. 5, p. 1276–1290. doi:10.1007/s12583-018-0843-z.
- Yan, Z.Y., Tang, K.D., Bai, J.W., and Mo, Y.C., 1989, High pressure Metamorphic rocks and their tectonic environment in northeastern China: Journal of Southeast Asian Earth Sciences, v. 3, p. 303–313. doi: 10.1016/0743-9547(89)90035-4.
- Yang, H.X., Li, P.W., and Yu, H.M., 1998, Palaeomagnetic study of the main terranes, northeast area, China: Journal of Changchun University of Science and Technology, v. 28, p. 202–205, 212. [in Chinese with English abstract.]
- Yang, H., Zhang, Y.L., Chen, H.J., and Ge, W.C., 2012, Zircon U–Pb ages of Khanka Lake granitic complex and its geological implication: Global Geology, v. 31, p. 621–630. [in Chinese with English abstract.]
- Yang, H., Ge, W.C., Zhao, G.C., Yu, J.J., and Zhang, Y.L., 2015a, Early Permian-late Triassic granitic magmatism in the Jiamusi–Khanka Massif, eastern segment of the central asian orogenic belt and its implications: Gondwana Research, v. 27, p. 1509–1533. doi: 10.1016/j.gr.2014.01.011.
- Yang, H., Ge, W.C., Zhao, G.C., Dong, Y., Xu, W.L., Wang, Z.H., Ji, Z., and Yu, J.J., 2015b, Late Triassic intrusive complex in the Jidong region, Jiamusi–Khanka Block, NE China: Geochemistry, zircon U–Pb ages, Lu–Hf isotopes, and implications for magma mingling and mixing: Lithos, v. 224–225, p. 143–159. doi: 10.1016/j.lithos.2015.03.001.
- Yang, H., 2017, Research on the Neoproterozoic-early Paleozoic tectono-magmatic events in the Jiamusi block: Changchun, Jilin University: [in Chinese with English abstract.]
- Yang, H., Ge, W.C., Dong, Y., Bi, J.H., Wang, Z.H., and Ji, Z., 2017a, Record of Permian–early Triassic continental arc magmatism in the western margin of the Jiamusi Block, NE China: Petrogenesis and implications for Paleo-Pacific subduction: International Journal of Earth Sciences, v. 106, p. 1919–1942. doi: 10.1007/s00531-016-1396-y.
- Yang, H., Ge, W.C., Zhao, G.C., Bi, J.H., Wang, Z.H., Dong, Y., and Xu, W.L., 2017b, Zircon U-Pb ages and geochemistry of newly discovered Neoproterozoic orthogneisses in the Mishan region, NE China: Constraints on the high-grade metamorphism and tectonic affinity of the Jiamusi-Khanka block: Lithos, v. 268–271. p. 16–31. doi: 10.1016/j.lithos.2016.10.017.
- Yang, H., Ge, W.C., Bi, J.H., Wang, Z.H., Tian, D.X., Dong, Y., and Chen, H.J., 2018, The Neoproterozoic-early Paleozoic evolution of the Jiamusi block, NE China and its East Gondwana connection: Geochemical and zircon U–Pb–Hf isotopic constraints from the Mashan complex: Gondwana Research, v. 54, p. 102–121. doi: 10.1016/j.gr.2017.10.002.
- Yang, H., Ge, W.C., Dong, Y., Bi, J.H., Ji, Z., He, Y., and Xu, W.L., 2019, Permian subduction of the Paleo-Pacific (Panthalassic) oceanic lithosphere beneath the Jiamusi Block: Geochronological and geochemical evidence from the Luobei mafic intrusions in Northeast China: Lithos, v. 332–333, p. 207–225. doi: 10.1016/j.lithos.2019.03.004.
- Yang, H., Xu, W.L., Sorokin, A.A., Ovchinnikov, R.O., and Ge, W.C., 2020a, Geochronology and geochemistry of Neoproterozoic magmatism in the Bureya Block, Russian Far East: Petrogenesis and implications for Rodinia reconstruction: Gondwana Research, v. 342. doi: 10.1016/j.precamres.2020.105676.
- Yang, Y., Liang, C.Y., Zheng, C.Q., Xu, X.C., Zhou, X., and Hu, P.Y., 2020b, The metamorphic characteristics of metapelites of the Mashan Group in Mashan area, eastern Heilongjiang Province: China: Constraint on the Crustal Evolution of the Jiamusi Massif: Gondwana Research doi: 10.1016/j.gr.2020.10.004.
- Yang, Y., Liang, C.Y., Zheng, C.Q., Xu, X.C., Zhou, J.B., Zhou, X., and Cao, C.G., 2021a, Metamorphic evolution of high-grade granulite-facies rocks of the Mashan Complex, Liumao area, eastern Heilongjiang Province, China: Evidence from zircon U–Pb geochronology, geochemistry and phase equilibria modelling: Precambrian Research, doi: 10.1016/j.precamres.2021.106095.
- Yang, Y., Zheng, C.Q., Liang, C.Y., Xu, X.C., Zhou, J.B., and Zhou, X., 2021b, in press. Mineral phase equilibria and zircon geochronology constraining the P–T–t path of granulite–facies metapelites of the Mashan Complex in the Shangsanyang area, eastern Heilongjiang Province, China: Precambrian Research, doi:10.1016/j.precamres.2021.106283.
- Zhang, M.S., Peng, X.D., and Sun, X.M., 1998, The Paleozoic tectonic geographical pattern of northeast China: Liaoning Geology, v. 2, p. 91–96. [in Chinese with English abstract.]
- Zhang, J., Sun, M., Schulmann, K., Zhao, G.C., Wu, Q., Jiang, Y.D., Guy, A., and Wang, Y.J., 2015a, Distinct deformational history of two contrasting tectonic domains in the Chinese Altai: Their significance in understanding accretionary orogenic process: Journal of Structural Geology, v. 73, p. 64–82. doi: 10.1016/j.jsg.2015.02.007.
- Zhang, X.R., Zhao, G.C., Eizenhöfer, P.R., Sun, M., Han, Y.G., Hou, W.Z., Liu, D.X., Wang, B., Liu, Q., and Xu, B., 2015b, Latest Carboniferous closure of the Junggar Ocean constrained by geochemical and zircon U–Pb–Hf isotopic data of granitic gneisses from the Central Tianshan block, NW China: Lithos, v. 238, p. 26–36. doi: 10.1016/j.lithos.2015.09.012.
- Zhang, X.R., Zhao, G.C., Eizenhöfer, P.R., Sun, M., Han, Y.G., Hou, W.Z., Liu, D.X., Wang, B., Liu, Q., and Xu, B., 2015c, Paleozoic magmatism and metamorphism in the Central Tianshan block revealed by U–Pb and Lu–Hf isotope studies of detrital zircons from the South Tianshan belt, NW China: Lithos, v. 233, p. 193–208. doi: 10.1016/j.lithos.2015.06.002.
- Zhang, X.R., Zhao, G.C., Sun, M., Eizenhöfer, P.R., Han, Y.G., Hou, W.Z., Liu, D.X., Wang, B., Zhu, Y.L., Liu, Q., and Xu, B., 2016, Tectonic evolution from oceanic subduction to arc–continent collision of the Junggar ocean: Constraints from U–Pb dating and Hf isotopes of detrital zircons from the North Tianshan belt: NW China: Geological Society of America Bulletin, v. 128, no. 3–4, p. 644–660. doi: 10.1130/B31230.1.
- Zhang, X.R., Zhao, G.C., Sun, M., Eizenhöfer, P.R., Han, Y.G., Hou, W.Z., Liu, D.X., Wang, B., Zhu, Y.L., Liu, Q., and Xu, B., 2016, Tectonic evolution from oceanic subduction to arc–continent collision of the Junggar ocean: Constraints from U–Pb dating and Hf isotopes of detrital zircons from the North Tianshan belt, NW China: Geological Society of America Bulletin. v. 128, no. 3–4, p. 644–660. doi: 10.1130/B31230.1.
- Zhang, S.W., Wang, F., Xu, W.L., Gao, F.H., and Tang, J., 2021, Tectonic history of the Huangsong tectonic terrains in the Khanka Massif in the easternmost central asian orogenic belt: Constraints from detrital zircon U–Pb geochronology: Gondwana Research, v. 99, p. 149–162. doi: 10.1016/j.gr.2021.07.002.
- Zhao, C.J., Peng, Y.J., and Dang, Z.X., 1995, The formation and evolution of crust in eastern Jilin and Heilongjiang provinces: Shenyang, Liaoning University Press, p. 1–226. [in Chinese with English abstract.]
- Zhao, G.C., Sun, M., Wilde, S.A., and Li, S.Z., 2004, A Paleo-Mesoproterozoic supercontinent: Assembly, growth and breakup: Earth-Science Reviews, v. 67, p. 91–123. doi: 10.1016/j.earscirev.2004.02.003.
- Zhao, G.C., 2014, Jiangnan Orogen in South China: Developing from divergent double subduction: Gondwana Research, v. 27, p. 1173–1180. doi: 10.1016/j.gr.2014.09.004.
- Zhao, G.C., Wang, Y.J., Huang, B.C., Dong, Y.P., Li, S.Z., Zhang, G.W., Yu, S., 2018, Geological reconstructions of the East Asian blocks: From the breakup of Rodinia to the assembly of Pangea: Earth-Science Reviews, v. 186, p. 262–286. doi: 10.1016/j.earscirev.2018.10.003.
- Zhao, C., Qin, K.Z., Song, G.X., Li, G.M., Li, Z.Z., 2019, Early Palaeozoic high-Mg basalt-andesite suite in the Duobaoshan Porphyry Cu deposit, NE China: Constraints on petrogenesis, mineralization, and tectonic setting: Gondwana Research, v. 71, p. 91–116. doi: 10.1016/j.gr.2019.01.015.
- Zheng, Y.F., Xiao, W.J., and Zhao, G.C., 2013, Introduction to tectonics of China: Gondwana Research, v. 23, p. 1189–1206. doi: 10.1016/j.gr.2012.10.001.
- Zhou, J.B., Wilde, S.A., Zhang, X.Z., Zhao, G.C., Zheng, C.Q., Wang, Y.J., and Zhang, X.H., 2009, The onset of Pacific margin accretion in NE China: Evidence from the Heilongjiang high-pressure metamorphic belt: Tectonophysics, v. 478, p. 230–246. doi:10.1016/j.tecto.2009.08.009.
- Zhou, J.B., Wilde, S.A., Zhao, G.C., Zhang, X.Z., Wang, H., and Zeng, W.S., 2010a, Was the easternmost segment of the central Asian orogenic belt derived from Gondwana or Siberia: An intriguing dilemma? Journal of Geodynamics, v. 50, p. 300–317. doi:10.1016/j.jog.2010.02.004.
- Zhou, J.B., Wilde, S.A., Zhao, G.C., Zhang, X.Z., Zheng, C.Q., and Wang, H., 2010b, Pan-African metamorphic and magmatic rocks of the Khanka Massif, NE China: Further evidence regarding their affinity: Geological Magazine, v. 147, no. 5, p. 737–749. doi:10.1017/S0016756810000063.
- Zhou, J.B., Wilde, S.A., Zhao, G.C., Zhang, X.Z., Zheng, C.Q., and Wang, H., 2010c, New SHRIMP U–Pb Zircon ages from the Heilongjiang Complex in NE China: Constraints on the Mesozoic evolution of NE China: American Journal of Science, v. 310, p. 1024–1053. doi:10.2475/09.2010.10.
- Zhou, J.B., Wilde, S.A., Zhang, X.Z., Ren, S.M., and Zheng, C.Q., 2011a, Early Paleozoic metamorphic rocks of the Erguna Block in the Great Xing’an Range, NE China: Evidence for the timing of magmatic and metamorphic events and their tectonic implications: Tectonophysics, v. 499, p. 105–117. doi:10.1016/j.tecto.2010.12.009.
- Zhou, J.B., Wilde, S.A., Zhang, X.Z., Zhao, G.C., and Liu, F.L., 2011b, A >1300 km late Pan-African metamorphic belt in NE China: New evidence from the Xing’an block and its tectonic implications: Tectonophysics, v. 509, p. 280–292. doi:10.1016/j.tecto.2011.06.018.
- Zhou, J.B., Zhang, X.Z., Wilde, S.A. and Zheng, C.Q., 2011c, Confirming of the Heilongjiang ~500Ma Pan-African khondalite belt and its tectonic implications: Acta Petrologica Sinica, v. 27, p. 1235–1245. [in Chinese with English abstract.]
- Zhou, J.B., and Wilde, S.A., 2013, The crustal accretion history and tectonic evolution of the NE China segment of the central asian orogenic belt: Gondwana Research, v. 23 no. 4, p. 1365–1377. doi: 10.1016/j.gr.2012.05.012.
- Zhou, J.B., Cao, J.L., Wilde, S.A., Zhao, G.C., Zhang, J., and Wang, B., 2014, Paleo-Pacific subduction–accretion: Evidence from geochemical and U–Pb zircon dating of the Nadanhada accretionary complex, NE China: Tectonics, v. 33, p. 2444–2466. doi:10.1002/2014TC003637.
- Zhou, J.B., Han, J., Zhao, G.C., Zhang, X.Z., Cao, J.L., Wang, B., and Pei, S.H., 2015a, The emplacement time of the Hegenshan ophiolite: Constraints from the unconformably overlying Paleozoic strata: Tectonophysics. v. 662, p. 398–415. doi:10.1016/j.tecto.2015.03.008.
- Zhou, J.B., Wang, B., Wilde, S.A., Zhao, G.C., Cao, J.L., Zheng, C.Q., and Zeng, W.S., 2015b, Geochemistry and U–Pb zircon dating of the Toudaoqiao blueschists in the Great Xing’an Range, northeast China, and tectonic implications: Journal of Asian Earth Sciences, v. 97, p. 197–210. doi: 10.1016/j.jseaes.2014.07.011.
- Zhou, J.B., Wilde, S.A., Zhao, G.C., and Han, L., 2018, Nature and assembly of microcontinental blocks within the Paleo-Asian Ocean: Earth-Science Reviews, v. 186, p. 76–93. doi: 10.1016/j.earscirev.2017.01.012.
- Zhu, G., Wang, Y.S., Wang, D.X., Niu, M.N., Liu, G.S., and Xie, C.L., 2006a, Constraints of foreland sedimentation and deformation on synorogenic motion of the Tan-Lu fault zone: Chinese Journal of Geology, v. 41, p. 102–121. [in Chinese with English abstract.]
- Zhu, G., Xu, Y., Liu, G.S., Wang, Y.S., and Xie, C.L., 2006b, Structural and deformational characteristics of strike-slipping along the middle-southern sector of the Tan-Lu fault zone: Scientia Geologica Sinica, v. 41, p. 226–241+255. [in Chinese with English abstract.]
- Zonenshain, L.P., Kuzmin, M.I., and Natapov, L.M. 1990. Geology of the USSR: A Plate Tectonic Synthesis: Geodynamics Series 21, AGU, Washington, DC, p. 242.
- Zorin, Y.A., 1999, Geodynamics of the western part of the Mongolia–Okhotsk collisional belt, Trans-Baikal region (Russia) and Mongolia: Tectonophysics, v. 306, p. 33–56. doi:10.1016/S0040-1951(99)00042-6.