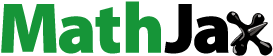
ABSTRACT
The Qinghai-Tibet Plateau (QTP) is one of the middle- and low-latitude regions with the most developed and well-preserved periglacial phenomena and remains in the world. On the northeastern QTP, periglacial remains are widespread and vital for investigating the evolution of regional periglacial and permafrost environments. The recently discovered periglacial remains on the northeastern QTP are mostly cryogenic wedges of Late Pleniglacial age (30–10 ka), deep and large cryogenic wedges mostly formed during 30–19 ka ago. Shallow cryogenic wedges have formed between 16 and 12.5 ka. A second wave of cryogenic wedge pseudomorphs seems to have followed, which was ending by about 7 ka. Based on the types of cryogenic wedges and in combination with stratigraphic data for dating, as well as the past Quaternary geological and periglacial landform data, we infer that the mean annual air temperature (MAAT) in this region during the local LPM (30–19 ka) was 7–9°C lower than that at present, and the lower limit of permafrost was lowered to about 2,200–2,500 m a. s. l. During the local LPM, permafrost was extensively and intensively developed on the northeastern QTP, and the continuous permafrost zone extended down to the source area of the Yellow River and the vicinity of the Zoîgé Plateau in the east. Next, discontinuous, sporadic and patchy permafrost occur in cascadingly lowering zones.
1. Introduction
Cryogenic remains are important indicators for palaeoclimatic environments and climatic stratigraphy and are valuable for reconstruction of the periglacial geomorphic evolution processes and Quaternary permafrost. The types known on the northeastern slopes of the Qinghai-Tibet Plateau (QTP) include cryogenic wedges and their pseudomorphs, cryogenic polygons, pingos, and cryoturbations (Cui et al. Citation2004; Harris et al. Citation2016, Citation2018; French et al. Citation2021). According to materials infilling the wedges and their lithology, their remains can be divided into relict sand wedges, ice wedge pseudomorphs, soil wedges, and composite wedge pseudomorphs (Murton Citation2013; French Citation2018; Wolfe et al. Citation2018). Among them, ice wedge pseudomorphs can be regarded as a reliable indicator for the occurrence of past permafrost, and remains of sand wedges in groups can be regarded as a reliable indicator of permafrost or deep seasonal frost (Murton et al. Citation2000; Murton and Kolstrup, Citation2003; Vandenberghe et al. Citation2004, Citation2019; Murton Citation2013; Bertran et al. Citation2014, Citation2017; Andrieux et al. Citation2016; Wolfe et al. Citation2018; Stadelmaier et al. Citation2021). In addition, cryogenic wedge structures and cryoturbations in coarse-grained sediments, such as gravels or weathered rocks, indicate a colder periglacial environment than those occurring in fine-grained subsoils (Romanovskii Citation1977; Vandenberghe et al. Citation2014; French et al. Citation2021). In summary, although ice-wedge pseudomorphs are a clear indication of past permafrost conditions, for sand wedges, it is necessary to consider the local geo-environmental factors, such as lithology, soil moisture, vegetation cover, snow thickness and others, in the reconstruction of the past periglacial environment.
Located at the intersection zone of three major natural regions, i.e. the eastern monsoon region, northwestern arid region and QTP, elevational permafrost on the northeastern QTP is very sensitive to climate change. Here, permafrost is thin, warm, and discontinuous and in a transition from the plateau to alpine permafrost. With cyclic climatic fluctuations and intermittent uplifts of the QTP in Quaternary glacial and interglacial periods, permafrost in this region has experienced some complicated evolutionary processes. During the last 150 ka, intensive expansion, shrinkage, and cycles of permafrost advances and retreats took place for at least four times (e.g. Pan and Chen Citation1997). The Late Pleniglacial (30–14 ka) was the period of the most intense permafrost expansion (Frechen et al. Citation2001; Jin et al. Citation2007, Citation2019, Citation2020; Bertran et al. Citation2014; Chang et al. Citation2017; Andrieux et al. Citation2018). Well-preserved periglacial remains for this period are diverse in genetic types and clear in morphology. In particular, many cryogenic wedge groups have been identified. They are very important for regional palaeo-permafrost and palaeoclimatic reconstruction.
During the last decade, six field studies have been carried out for collecting or clarifying the evidence of former and present-day permafrost preserved in the Late Pleniglacial sediments across the vast area on the northeastern QTP. Periglacial features, such as cryoturbations and cryogenic wedges, among others, have been examined, described, and sampled for Optically Stimulated Luminescence (OSL) and radiocarbon (AMS-14C) dating. In addition to earlier research results, these data were summarized, analysed, and systematically integrated for a better understanding of the permafrost distribution and palaeo-environment in this region during the local Last Permafrost Maximum. In addition, field investigations have also been conducted on the adjacent semi-arid regions to the north to elucidate the regional palaeopermafrost environment (e.g. Jin et al. Citation2019, Citation2020; He et al. Citation2021) to define the extents of present-day and earlier distribution of permafrost and seasonal frost in North-Central China during the LPM (Vandenberghe et al. Citation2019). From a comprehensive perspective, these study results will be of importance for the palaeoenvironmental reconstruction on the northeastern QTP and for delineating the southern and lower limits of the periglacial environment and elevational permafrost in China during the Late Pleistocene on a wider extent than before.
2. Materials and methods
2.1 Study region
The QTP is a unique geographical unit with the highest and largest plateau (2.6 million km2) on the earth. Its geomorphology is characteristic of rugged terrains and high elevations (>4,500 m a. s. l. on average) in the Interior of the QTP and >3,000 m a. s. l. in the periphery mountains. The study region is located on the northeastern QTP with a series of approximately paralleling mountains, basins, and valleys, thus forming the regional geomorphology and landform patterns (). The eastern Qilian Mountains in the north is an enormous marginal mountain system in transition to northern deserts; at the northwestern end, the Qaidam Basin is surrounded by mountains; the terrain in the east and northeast is low with alternating valleys of the upper Yellow River and its tributaries on the Longdong (eastern Gansu Province) Loess Plateau. It is stopped by the Qinling, Gongga, and Hengduan mountains in the east and southeast. In the west, southwest, and northwest, it is bordered by the eastern Kunlun and Bayan Har mountains.
Figure 1. (A) Map of China. (B) Map of the Qinghai-Tibet Plateau (QTP). (C) Location of the study area and distribution of sample sites for palaeo-permafrost remains on the northeastern Qinghai-Tibet Plateau (QTP), Southwest China.The red stars are the sample sites. SDHF, Shandan Horse Farm (); HCZ, Huangchengzi section in the Menyuan Basin, Qinghai Province, China (); MQHF, Maqu Horse Farm, southern Gansu Province, China (); NTS, Mt. Niutoushan on the west bank of the Ngöring Lake in the HAYR (); HHV, Huang’he Village, Madoi County, Qinghai Province in the SAYR (); QJT, Qiejitan (); XH, Xinghai (Fig. 11).
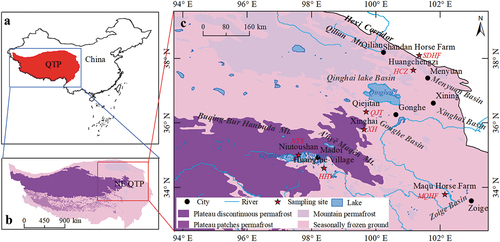
From south to north, the study region consists of the Zoîgé (Ruo’ergai) Plateau and the headwater area of the Yellow River (HAYR), Xinghai, Gonghe, and Qinghai Lake basins. Most of these mountain ranges have elevations over 4,500 m a. s. l (). Vegetation in the region is generally sparse and monotonous; it consists mainly of alpine meadows, alpine steppes, and cushion-like vegetation at higher mountains and hill-tops (Luo et al. Citation2018). Soil types mainly include alpine meadow soil and paludal meadow soil developed in lowlands, gentle piedmont slopes, and intermontane basins. Main rivers include the upper Yellow and Tongtian-Yangtze rivers and their tributaries, and; larger lakes include Gyaring, Ngöring, Donggi Cona, and Xingxinghai lakes.
At present, this region is characteristic of semi-arid and cold continental climate at high elevations. It is in a zone with coexistence of seasonal frost, isolated patches of permafrost, and sporadic and discontinuous permafrost (Zhou et al. Citation2000; Li et al. Citation2016; Jin et al. Citation2019). During the Late Quaternary, alpine regions on the northeastern QTP were extensively glaciated, leaving behind many glacial landforms and sediments (Shi, Citation1995; Heyman et al. Citation2008, Citation2009; Jin et al. Citation2020). The surrounding intermontane basins, valleys, and high plateaus provided the vacant places for the periglacial action and permafrost development, as evidenced by a large number of palaeo-periglacial phenomena and palaeo-permafrost remains. Many of these wedges are well preserved with distinct and characteristic features.
2.2 Field methods
shows the distribution of several types of cryogenic wedges with distinct patterns found in the study area during the fieldwork. Typical periglacial features, such as cryoturbations, cryogenic wedges, and lithology and morpholog ical features of their profiles where they were located, as well as landform and surface conditions in the nearby areas, were observed in detail, and 28 samples were collected at proper positions and depths for OSL dating from the infillings or hosting sediments of freshly exposed wedges by hammering in the metal tubes (60 mm in diameter, 300 mm long). A visual inspection ensured that the sample cylinders did not penetrate beyond the wedges into the host sediment or a vein penetrating the original wedge structure. The host sediments were also sampled. Samples remained tightly sealed until processing under subdued red-light conditions. Six sections with cryogenic wedge structures, including ice wedge pseudomorphs, soil wedges or sand wedges or cryoturbations, were described across the study area. Several of them were described in Harris and Jin (Citation2012) and Harris et al. (Citation2018), while Pan and Xu (Citation1989) and Pan and Chen (Citation1997) obtained some radiocarbon dates from two other sections.
2.3 Laboratory methods
Samples were submitted to the dating lab of the Qinghai Institute of Salt Lakes, Chinese Academy of Sciences, Xining, China. All laboratory pre-treatment, sample preparation, and luminescence measurements were conducted under the subdued red light. For each end of sample tubes, about 3 cm of material were removed. The middle portion material in the tube was used to determine the equivalent dose (De). All samples using the standard preparation procedure (treatment with 10% HCl and 30% H2O2, wet sieving, 35% H2SiF6, and 10% HCl in sequence) (Lai and Wintle Citation2006; Roberts Citation2007; Mischke et al. Citation2013; Wang et al., Citation2018; Yu et al., Citation2020).
The optically stimulated luminescence (OSL) signals were measured using a Risø Thermoluminescence (TL)/OSL-DA-20 reader fitted with blue diodes () and an infrared (IR) laser diode (
). Irradiation was carried out using a 90Sr/Y90 beta sources with a dose rate of 0.089 Gy/s built into the reader. For the detailed SAR procedures, we refer to Lai et al. (Citation2008) and Wang et al. (Citation2018).
The quartz equivalent doses (De) were determined by the single-aliquot regenerative-dose (SAR) (Murray and Wintle Citation2000, Citation2003) and the standard growth curve (SGC) method (Roberts and Duller Citation2004; Lai Citation2006; Lai et al. Citation2007). For the detailed dating procedures, we refer to the publications of Lai et al. (Citation2014), Wang et al. (Citation2018), and Yu et al. (Citation2020). In order to select appropriate preheat conditions for the De determination using the SAR protocol, a pre-heat temperature plateau test was conducted for sample YRV-TL5 as an example (). Pre-heat temperatures varied from 220°C to 300°C at an interval of 20°C for 10 s, using a heating rate of 5°C/s, and the cut-heat temperature was fixed at 220°C. A plateau was observed for temperatures ranging from 260°C to 300°C (). The recycling ratios for different measurement temperatures all fall in the range of 0.9–1.1, varying between 0.93 and 1.10. Recuperation for 260°C preheat temperatures is less than 10% of the natural signal, ranging from 4% to 8%. Based on these results, the SAR sequence with a preheat temperature of 260°C and a cut-heat of 220°C was used for the SAR measurement of these samples. Dose recovery tests were carried out on natural samples to check the performance of SAR measurements as described by Murray and Wintle (Citation2003). The same preheating conditions and measurement sequence as selected for dating were used. The tests were conducted on the samples of YRV-TL5 and MQ-3 with given doses of 26 and 52 Gy, close to their natural De values. Six natural aliquots were stimulated twice by the blue-light stimulation at 130°C for 60 s. These doses were then measured in the same way as the natural doses. The measured values of Des were 23.5 ± 0.5 and 49.3 ± 0.8 Gy. Thus, the ratios of measured to given De values were 0.90 ± 0.02 and 0.95 ± 0.02, respectively, and they were within the acceptable limit. The dose recovery test results suggest that the selected SAR procedure conditions of the OSL dating were appropriate for the De determination. shows typical OSL decay curves and dose response curves (DRC) of sample YRV-TL5. During the first second of stimulation, the OSL signals rapidly declined, indicating that the OSL signal was mainly the fast component (Singarayer and Bailey Citation2003). The OSL dating results are listed in .
Figure 2. Preheat plateau test of OSL signal on temperature for sample YRV-TL5.
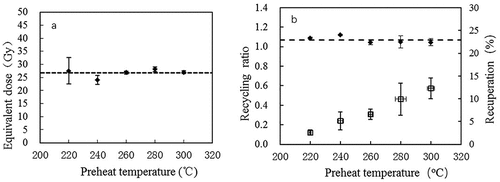
Figure 3. Growth curves (a) and OSL decay curves (d) of sample YRV-TL5.
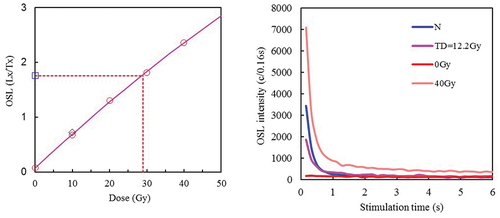
Table 1. Formation ages of sediments that contain permafrost and periglacial remains on NE Qinghai-Tibet Plateau.
2.4 Temperature inference
Temperature values in this study mainly include mean annual ground temperatures (MAGT) of palaeo-permafrost (Palaeo-MAGT) and palaeo-MAAT. Palaeo-MAGT is an important indicator for the occurrence and development of past permafrost. These temperatures can be inferred using cryogenic wedges, so as to obtain the palaeo-MAGT of wedge formation periods and to estimate the corresponding palaeo-MAAT. Cryogenic wedges were closely related to adjacent lithology, soil moisture content, and ground temperatures (Romanovskii Citation1977). The coarser the soil particle, the smaller the soil moisture content, the lower the ground temperature for the formation of cryogenic wedges (Romanovskii Citation1977). An MAGT of −2 ~ −1°C or lower is required to form ice wedges in the fine-grained soils; that of −5 ~ −3°C, soil and sand wedges in coarse-grained soils, and; that of −6 ~ −5°C, or lower, ice wedges in the coarse-grained soils. The inversion method of palaeo-MAGT using cryogenic wedges has been extensively applied to research on Quaternary permafrost (e.g. Vandenberghe Citation1983; Harris and Jin Citation2012; Vandenberghe et al. Citation2014, Citation2016, Citation2019; Harris et al. Citation2018; Jin et al. Citation2019), although a systematic error bar should be accounted for coping with different local conditions, such as vegetation cover, snow cover, and topography (Murton and Kolstrup, Citation2003; Van Huissteden et al. Citation2003). Therefore, this method was also used in this study for inversion of palaeo-MAGT.
3. Results
Since the study area in this paper is large and includes several distinct regions and a variety of environmental situations, the main results for each region are discussed starting with the most northerly site, which is also at the lowest elevation.
3.1 Northern slopes flank of the Qilian Mountains
This area receives cold air drainage from the Qilian Mountains in winter and snow meltwater from mountain slopes in spring and early summer. It is transitional between the mountain forest zone on the north Qilian mountain slopes and the semi-desert of the Hexi Corridor. The vegetation mainly consists of steppe grasslands.
The Shandan Horse Farm section (38°06′1.6″N, 101°20′27.8″E; 2,891 m a. s. l.) lies on the north slope of the Qilian Mountains slightly above the Hexi Corridor (). Two kinds of wedges were identified (Harris, Citation2018): the first being narrow wedges with vertical lamination, filled by sediment very similar in colour to the host sediment, interpreted as being a primary sand wedge, which occurs upslope from wedge ‘a’ (). The second type of wedge occurs further downslope (wedge ‘b’) (), lacks vertical lamination, and is typically >2.0 m deep and approximately 2.0 m wide at the top. The upper infill is much younger than that in the lower part (, ), suggesting a much later formation time of the upper part in comparison with the lower part of the wedge. The lower infilling includes blocks of the host sediment, suggesting that the filling originated from the collapse of the walls and possibly in part from primary sand filling. The date for the lowest sample is the oldest (), but there is no clear evidence for host origin. Sampling for OSL dating in various elementary sand veins provides various ages but sand wedges can open and fill repeatedly during the Last Glaciation and afterwards (Andrieux et al. Citation2018). The date for the lowest sample is probably that of the host sediment.
3.2 Menyuan basin
Large involutions, located at Huangchengzi village (37°38′25.2″N, 101°9′43.3″E; 3,148–3,153 m a. s. l.) in the Menyuan intermontane basin at the southern foothills of the Qilian Mountains, have been previously discussed by Wang et al. (Citation2013), Vandenberghe et al. (Citation2016, Citation2017) and Harris et al. (Citation2016). The section consists of a lower layer of river gravels more than 4.5 m thick (bottom not seen), overlain abruptly and conformably by a layer of sandy silt up to 1.4 m thick. The involutions have developed as a continuous series of very regular and symmetrical folds with an amplitude of >4.5 m. The involutions include upward pointed and lobate protrusions of gravels into fine sands and silts, and downward protrusions of sands and silts into gravels, they are characterized by a symmetrical form and a balance in dimensions between the parts that have moved upwards and downwards. By analogy to similar structures (e.g. Vandenberghe, Citation1992), the involutions of this size are attributed to periglacial load casting occurring above degradation permafrost (type 2 cryoturbations).
Six OSL dates have been obtained, three by Vandenberghe et al. (Citation2016) and the other three in this study (, ). The latter span a time slot from 30.0 to 19.3 ka. The involutions were formed when permafrost degraded shortly after 19 ka. The presence of cryoturbations and absence of sand wedges in the Menyuan basin contrasts with the general presence of sand-wedges and absence of cryoturbations in adjacent regions, indicating a much more humid northeastern QTP in comparison with adjacent desert environments (Vandenberghe et al. Citation2004; Wang et al. Citation2013).
3.3 Zoîgé Peat Plateau
A gravelly sand wedge with vertical lamination () was exposed in a roadcut near the Maqu Horse Farm on the Zoîgé Plateau (33°47′1.8″N, 102°08′17.2″E; 3,447 m a. s. l). It was overlain by a gravel layer washed down from a nearby fault and two layers of younger loess. This section has been previously described by Harris et al. (Citation2018). The Zoîgé Plateau is located further east of Maqu where the Yellow River turns north between north-south trending mountain ranges ().
Figure 6. (A) Sand wedge profile at a roadcut at the southern side of the Yellow River near the Maqu Horse Farm on the Zoîgé Plateau on northeastern Qinghai-Tibet Plateau, Southwest China (data in ) (Some of the information from Harris et al. (Citation2018). Notes: a) Sand wedge filling, and; b) The sample site for OSL dating in the overlying loess.). (B) Schematic diagram illustrating the section. (C) Schematic diagram illustrating sampling profile.
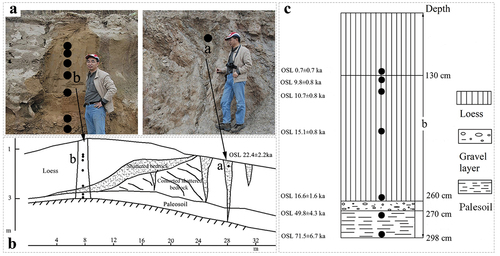
The OSL-dating of the wedge fill (a) is 22.4 ± 2.2 ka (, ). Section b is a complex loessic series consisting of rather old coarse-grained substratum sediments (the two lowermost samples), overlain by a ‘lower’ loess that was deposited during the same interval as the wedge formation (samples at depths of 1.90 and 2.60 m). Fill sediment was OSL-dated at 0.73 ± 0.66 ~ 71.5 ± 7.6 ka BP, whereas that below the loess, at 9.8 ± 0.8 ~ 16.6 ± 1.6 ka BP, and the palaeosol layer was formed during 49.8 ~ 71.5 ka (, ).
This area is located further to the east between mountain ranges in a cold air drainage location. This presumably explains the presence of a primary wedge, together with its relatively high elevation.
3.4 Headwater area of the Yellow River
3.4.1 Section of cryogenic wedges at a roadcut along the access road to Mount Niutoushan, west bank of the Ngöring Lake.
A wedge, 1-m-deep and approximately 1.0-m-wide at the top, filled with loessic sediment, showing the upturning of the host sediment, was found along the access road to Mount Niutoushan (35°00′20.1″N, 97°35′58.0″E; 4,304 m a. s. l.) along the west bank of the Ngöring Lake in the Headwater area of the Yellow River (). The structural characteristics point to a sand wedge. The wedges developed in weathered and crushed schist deposits were dated between 46 and 37 ka while the infilling of (aeolian) silty loess was dated at 16 ka (, ). The infilling of the upper part is dated at 10.8 ka. They were overlain unconformably by colluvium dated at 1.2 ka (, ). The size of the wedge is strikingly shallower than the usual wedges of LPM age, as those at Maqu and Shandan horse farms.
3.4.2 Section of cryogenic wedges along the Yellow River crossing near Huang’he Village, Madoi County.
The Huang’he village site is in the gorge that is subject to cold air drainage in winter (Luo et al. Citation2018, Citation2020). Several loess wedges were exposed in the cutting on the west side of the Yellow River crossing just beyond Huang’he village (34°36′10.8”N, 98°23′0.8”E; 4,214 m a. s. l.) (). The host bedrock consisted of weathered schists deposits, the top of which yielded an age of c. 11.5 ka. This substratum and the wedges that formed in it were truncated by an erosion surface, which was overlain by two layers of younger loess dated at 1.7 and 1.2 ka. The main wedge had weak vertical lamination while its bowl-shaped upper part (OSL dated at 8.7 ka) had a colour different from that of the lower part. Obviously, loess was windblown over the area during the deglaciation period up to the late Holocene. The structural characteristics of the wedge possibly point to a composite wedge pseudomorph or an ice wedge pseudomorph. The main wedge is very similar in appearance and age (c. 13 ka) to the wedge in front of the Mount Niutoushan ().
Figure 8. Composite wedge pseudomorph or ice wedge pseudomorph nearby the Huang’he Village, Madoi County, Qinghai Province in the source area of the Yellow River on the northeastern QTP (data in Table 1).
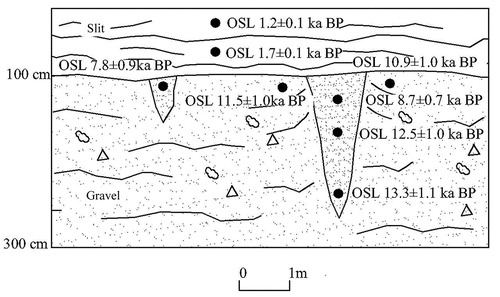
A second smaller, 60-cm-deep wedge occurred off to the side of the large wedge and its infilling yielded a date of 7.9 ka. Its age corresponds well with the bowl-shaped upper part of the main wedge. This section is very close to the road section near Madoi as described by VandenbergVandenberghe et al. (Citation2020). In that section, a few large and deep sand-wedges date from the LPM time, next to several shallow wedges of younger age.
3.4.3 Wedges on the access road from the western bank of the Ngöring Lake to Mount Niutoushan
Cheng et al. (Citation2005a, Citation2005b, Citation2006) found a series of ‘ice-wedge pseudomorphs along the road to Mount Niutoushan from the Ngöring Lake (~4,200 m a. s. l.). These were re-examined by Harris and Jin (Citation2012), who discovered a ‘rock tessellon’ (wedge) in the section, cut by one of the ‘ice-wedge pseudomorphs (). The host rock consists of frost-shattered schist with an OSL date of 38.9 ka. The rock must have been subjected to very cold and possibly moist conditions. The shattered schist has been cryoturbated. At an unknown time, a wedge filled with oriented plates of schist developed, partly in the master-joint/fault-plane. This would presumably have occurred during very cold and dry conditions when there was no moving aeolian deposit or moisture to form primary wedges. The sandy fill showed no sign of vertical foliation and provided an OSL date of ±13.49 ka (Harris et al., Citation2012). Later, the surface was eroded and covered unconformably with loess. Infillings of small-scale wedge structures in the top 50 cm of the loess provided dates of 5.5–6.0 ka.
Figure 9. Rock tessellon (wedge) at 35° 0′ 17.7″ N, 97° 35′ 58.3″ E on the access road from the Ngöring Lake to Mt. Niutoushan (Bullhead Hill) with its peak at an elevation of 4,610 m a. s. l. (from Harris et al., Citation2012).
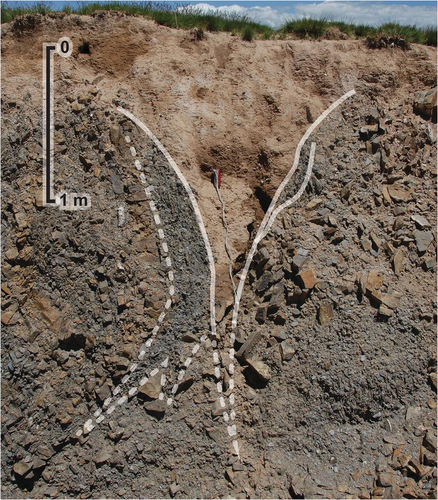
3.5 Results obtained by others
3.5.1 Gong’he Basin
Cryogenic wedges are widely distributed along the high terraces of the Yellow River and along the banks of the mouth of the Shazhuyu River, a tributary of the Yellow River. All kinds of wedges could have occurred.
Pan and Xu (Citation1989) reported a wedge 0.8 m in width and 1.2 m in depth, filled with slumped medium-fine sand and clay from the host sediment (), and radiocarbon dated at 20,430 ± 430 a BP. The adjacent host sediment was upturned and so the wedge was regarded as an ice-wedge pseudomorph (Pan and Xu Citation1989). However, it is now re-considered possibly as a sand wedge. It was overlain by a layer radiocarbon dated at 19,430 ± 360 a BP. This, in turn, was overlain by a palaeosol and loess. At this time, there were ample loess and sand dune deposits accumulated during the cold and dry preceding millennium. These same aeolian deposits also occurred in the nearby Xinghai, Guide, and Linxia basins on the northeastern QTP.
Figure 10. Ice-wedge pseudomorphs as reported by Pan and Xu (Citation1989) at Qiejitan (36°21“N, 99°42”E; 3,380 m a. s. l.) in the western Gong’he Basin in the Source Area of the Yellow River on the northeastern QTP, Southwest China.
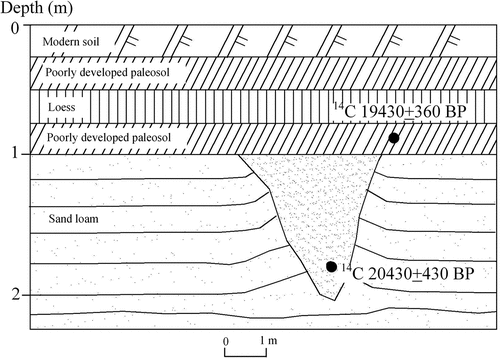
3.5.2. Xinghai Basin
Pan and Chen (Citation1997) reported wedges on the bank of the Yellow River in the Xinghai Basin. They were found in the upper layers of outwash gravels radiocarbon-dated at 33,880 ± 3450 a BP. The wedges and gravels were overlain unconformably by a layer of sandy loam, dated at 14,220 ± 180 a BP and a layer of loess ().
Figure 11. Sand wedges on the bank of the Yellow River in the Xinghai Basin (35°48′N, 99°38′E; 3,400 m a. s. l.) in the Source area Area of the Yellow River on the northeastern Qinghai-Tibet Plateau (QTP), Southwest China.

The Xinghai Basin is an isolated inland drainage basin surrounded by mountains with semi-desert and/or desert vegetation. The water evaporates, creating clouds which then locally produce precipitation which supports a meadowy grassland around the lake. Cryogenic wedges have been developed on the various terrace levels.
4. Discussions
4.1 Reliability of OSL dates
In spite of its popularity for dating sediments in arid regions where there is a lack of organic matter for radiocarbon dating, the OSL dating presents some problems. It necessarily presumes that the filling of the wedges comes from the surface and has no inherited properties from its previous history. If the infilling sediment has inherited properties, the OSL date will be too old. When sediment is detached from the wall of the thawing wedge, it inevitably contaminates the infilling, producing a hybrid age when used for OSL dating. Nevertheless, OSL has been successfully applied before to relict sand wedges as they contain well-bleached quartz-rich aeolian material (e.g. Murton et al. Citation2000; French et al. Citation2007; Buylaert et al. Citation2009; Bateman et al. Citation2010, Citation2014; Lai et al. Citation2014; Remillard et al. Citation2015; Andrieux et al. Citation2018).
At the time of sampling, in order to avoid sample contamination, samples were selected according to sedimentary context and, wherever possible, from close to boundaries between sedimentary units; the length (30 cm) of the sampling tubes is not a problem since only the middle part of the tube is used for OSL measurements while the near-perpendicular position of the wedges has been checked when digging up the tubes (in case of contamination by adjacent sediment the sampling has been re-done). In addition, a large portion of the samples were also collected from the host sediments for comparison with the wedge samples. It is worth pointing out that, as mentioned in Andrieux et al. (Citation2018), ‘One issue when using standard OSL dating is that the age reflects the average age of the individual veins present in each sample’, more effort in dating is required to improve the accuracy of the identified phases of thermal contraction cracking in future.
4.2 The timing of the formation of the Late Pleistocene wedges
To sum up, on the northeastern QTP, the discovered periglacial remains are mostly cryogenic wedges of the Late Pleniglacial (30–15 ka). This cold period is basically consistent with the results of previous studies (Zheng Citation1990; Zhao et al. Citation2013; Harris et al. Citation2016; Vandenberghe et al. Citation2016; Jin et al. Citation2019, Citation2020). Most of cryogenic wedges were developed in basins, valleys, high plateaus, or low foothills above 3,200 m a. s. l. Cryogenic wedges were mostly formed in the Quaternary strata, but some of them were formed in or penetrated through deeply weathered rocks. Fillings were mainly sand mixed with gravels or loess with different cementation degrees, and they were penetrated by cryogenic cracks of later periods. It is most important to state that the deep and well-developed wedges mostly formed at 30–19 ka. They point to continuous permafrost conditions. After 19 ka, the formation of deep and large cryogenic wedges disappeared. There was only widespread development of shallow sand wedges pointing to local and short phases of permafrost or even absence of permafrost with only deep seasonal frost. The latter wedges have been formed between 16 and 12.5 ka. Another wave of cryogenic wedge pseudomorphs seems to have followed, which was ending by about 7 ka, presumably reflecting later climate fluctuations before the onset of the Holocene Megathermal period. Such conditions of frozen ground are in accordance with the findings of Wolfe et al. (Citation2018), Bertran et al. (Citation2017) and Andrieux et al. (Citation2018) in France and previous results from the Ordos Plateau (Vandenberghe et al. Citation2004, p. 2018).
Based on the age of cryogenic wedges, climate changes, and transitions occurred during the Late Pleistocene on the northeastern QTP, China. They can be divided into three periods of cold climates: Local LPM (30–19 ka) with continuous permafrost development and expansion; Last Deglacial (16–12.5 ka) with permafrost degradation and local permafrost or only deep seasonal frost, and; early Holocene (12.5–7 ka) with some cool periods with deep seasonal frost.
4.3 Reconstruction of the LPM permafrost environment
Ice-wedge pseudomorphs, sand wedges, composite-wedge pseudomorphs, and cryoturbations can provide important information in the reconstruction of periglacial conditions in past and present environments. No other palaeo-permafrost feature has so significantly contributed to our knowledge of former extents and exact timing of permafrost formation at regional and local scales. Based on the type of cryogenic wedges and in combination with stratigraphic data for dating, as well as the past Quaternary geological and periglacial landform data, climate, and permafrost in this region during the LPM are further discussed as follows.
4.3.1 Cooling of the LPM
MAGT is generally higher than MAAT by about 2°C to 3°C in this region (Luo et al. Citation2018, Citation2019, Citation2020). Based on multiple cryogenic wedges found on the northeastern QTP during the LPM, the inversion method of Romanovskii (Citation1977) and local conditions were combined for inferring the corresponding palaeo-temperatures. Taking the cryogenic wedges () discovered at Qiejitan in the Gong’he Basin as an example, the hosting materials of these wedges were coarse and medium sands, in which an MAGT of – 6 ~ – 5°C was required for the formation of ice-wedges, and that of – 4 ~ – 3°C, for sand wedges (Romanovskii Citation1977). At present, the MAAT at Gong’he is about – 0.5°C and the MAGT, 2 ~ 3°C. Thus, the MAAT during the LPM was about 6 ~ 9°C lower than that at present. Taking the sand wedges () in the Maqu Horse Farm No. 1 as an example, their hosting soils were gravel and loess. MAAT and MAGT in the Maqu Basin at present are about 2°C and 5°C, respectively. The highest ground temperature needed to form sand wedges was lower than – 4 ~ – 3°C (Romanovskii Citation1977), and then MAAT of the LPM was 8 ~ 9°C lower than present. Accordingly, the climate cooling of the local LPM was 7 ~ 9°C in the HAYR, 7 ~ 8°C in the Xinghai Basin, 6.5 ~ 8.0°C in the Qinghai Lake Basin, and 7 ~ 9°C in the east and middle segments of northern flanks of the Qilian Mountains.
Previous studies showed that the MAAT in the middle and high parts of the QTP during the LPM, or Last Glacial Maximum (LGM), was 7°C colder than today (Shi et al. Citation1995; Shi Citation2006); on the interior QTP, it was 5 ~ 7°C colder; in the peripheries, 8–10°C colder than today (Zheng Citation1990); on the Zoîgé Plateau, 5.8 ~ 7.9°C colder than today (Shen and Tang Citation1996), and; in the Menyuan Basin, at least 7°C colder than today (Wang et al. Citation2013). Moreover, climatic model reconstruction results on the QTP have manifested an LGM cooling of 6°C (Böhner and Lehmkuhl Citation2005) and 1.8 ~ 6.4°C (Ju et al. Citation2007). Based on vegetation reconstruction and inversion, Wu et al. (Citation2019) concluded that, in the coldest and warmest months, it was cooled by ~11.0 ± 1.6 and ~2.6 ± 0.9°C, respectively. This result is less intensive than the previous estimates.
In conclusion, the climate cooling inferred by different proxies is basically similar, all indicating an extremely cold climate during the local LPM. As an overall consideration, it is proper to set the MAAT lowering of the local LPM at 7 ~ 9°C in this region.
4.3.2 Permafrost on the northeastern QTP during local LPM
On the northeastern QTP, elevation controls permafrost distribution, but latitude and longitude also exert certain influences (VandenbergVandenberghe et al. Citation2020). According to statistical data, MAATs at the contemporary lower limit of permafrost (LLP) in this region range around – 3 ~ – 2°C. When the MAAT declines by 1°C, the LLP correspondingly would descend by 160 ~ 170 m (Wang and Luo Citation1991; Luo et al. Citation2018, Citation2020). According to the estimated climate cooling of 7 ~ 9°C in this region during the local LPM and by reference to the spatial distribution of local LPM wedges and other permafrost remains, as well as lowering of the upper timberline, the LLP of local LPM was 1,200–1,400 m lower than that at present. This inference agrees well with the prevailing understanding by Jin et al. (Citation2007), (Citation2019) and Chang et al. (Citation2017). The plateau permafrost extended into several peripheral basins of the northeastern QTP, including the Gong’he and Qinghai Lake basins and the Zoîgé Plateau (). At that time, permafrost was continuous on the QTP, while alpine permafrost dominated the surrounding mountains. The LLP on the main mountain ranges has been inferred: 2,200–2,400 m a. s. l. for the Maya Snow Mountain in the eastern Qilian Mountains, 2,200–2,400 m a. s. l. for the Lenglongling Mountains, 2,300–2,500 m a. s. l. for north slopes of the South Mountains of the Hexi Corridor, 2,600–2,800 m a. s. l. for the eastern Bayan Har Mountains, 2,400–2,600 m a. s. l. for the Anymaqên and Öla mountains, and 2,300–2,500 m a. s. l. for the Dalijia, Laji and Qinghai South mountains.
Figure 12. The estimated extent of permafrost during the Last Permafrost Maximum (LPM) (Enlarged, updated and modified from Jin et al. Citation2019).
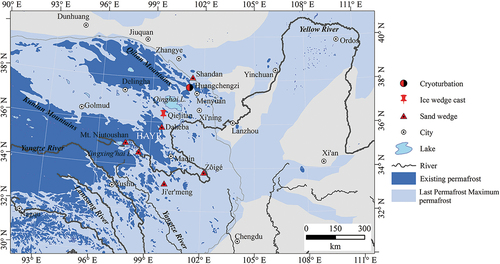
The elevation of the lower boundaries of numerous cryogenic wedges and cryoturbations indicate that the whole QTP was extensively covered with permafrost or glaciers during the local LPM, and the continuous permafrost zone on the plateau extended northeastwards. At that time, the headwater area of Yellow River and Xing’hai Basin were in the continuous permafrost zone. Mountains around this region were all underlain by stable, continuous alpine permafrost centred on their peaks, and by sporadic and patchy permafrost in other surrounding regions. The overall permafrost extent on the QTP reached about 2.2 million km2, 40% larger than that of the present (Jin et al. Citation2019). Then, the Gong’he and Qinghai Lake basins were both in permafrost zones. During the same period, the permafrost extent on the adjacent Ordos Plateau was also enlarging (He et al. Citation2021). Vandenberghe et al. (Citation2019, Citation2020) inferred the southern boundary of continuous permafrost on the Ordos Plateau a bit south of Wushenqi (Uxin Banner), Inner Mongolia Autonomous Region, North China.
5. Conclusions
1) Based on the age of the cryogenic wedges, climate changes and transitions occurred during the Late Pleistocene on the northeastern QTP, Southwest China, which could be divided into three periods of cold climates: local LPM (30–19 ka); Last Deglacial 16–12.5 ka, and early Holocene (12.5–7 ka).
2) During the local LPM, cryogenic wedges of diversified types occurred extensively on the northeastern QTP. According to climatic and environmental conditions needed to form these cryogenic wedges and ambient soil characteristics, as well as comprehensive analyses of glacial, palaeontological and other periglacial evidence, the authors concluded that this region was extremely cold and arid during the local LPM. The inferred MAAT at that time was 7–9°C lower than at present and precipitation was also reduced to a large extent.
3) Based on the spatial distribution of cryogenic wedges and lowering of the upper timberline, the inferred LLP was about 1,200–1,400 m lower than the contemporary LLP by inferring from the lowering of 7–9°C in MAAT and by integration with other evidence. In this region, permafrost was very well developed, and the continuous permafrost zone on the QTP expanded greatly towards the east and north, and the source area of the Yellow River fell into the continuous permafrost zone during the local LPM.
Acknowlegements
We sincerely thank Editor Joshy and the anonymous reviewers for many constructive and detailed comments on the manuscript, and we greatly appreciate their generous time and effort spent on this paper. We also thank Professor Stuart A. Harris for his generous help and guidance. This research is jointly supported by the programme of Gansu Province Science and Technology Project (21JR7RA057), CAS Strategic Pilot Science and Technology Project (Grant No. XDA05120302), and the programme of National Natural Science Foundation of China (41401081). Special thanks also given to Director and Professor Qingbai Wu with the State Key Laboratory of Frozen Soils Engineering for his staunch support for some field investigations. The late Professor Hugh M. French provided some inspiring comments during several joint fieldtrips and generous guidance for the preparation of the manuscript. The authors are very appreciative of their efforts
Supplemental Material
Download MS Word (21.2 KB)Disclosure statement
No potential conflict of interest was reported by the author(s).
Supplementary material
Supplemental data for this article can be accessed online at https://doi.org/10.1080/00206814.2022.2137860
Additional information
Funding
References
- Andrieux, E., Bateman, M.D., and Bertran, P., 2018, The chronology of Late Pleistocene thermal contraction cracking derived from sand wedge OSL dating in central and southern France: Global and Planetary Changes, v. 162, p. 84–100. 10.1016/j.gloplacha.2018.01.012
- Andrieux, E., Bertrain, P., Antoine, P. et al. 2016, Spatial analysis of the French Pleistocene permafrost by a GIS database: Permafrost and Periglacial Processes, v. 27, no. 1, p. 17–30. 10.1002/ppp.1856
- Bateman, M.D., Hitchens, S., Murton, J.B. et al. 2014, The evolution of periglacial patterned ground in East Anglia, UK: Journal of Quaternary Science, v. 29, no. 4, p. 301–317. 10.1002/jqs.2704
- Bateman, M.D., Murton, J.B., and Boulter, C., 2010, The source of De variability in periglacial sand wedges: Depositional processes versus measurement issues: Quaternary Geochronology, v.5, no. 2–3, p. 250–256. 10.1016/j.quageo.2009.03.007
- Bertran, P., Andrieux, E., Antoine, P. et al. 2014, Distribution and chronology of Pleistocene permafrost features in France: Database and first results: Boreas, v. 43, no. 3, p. 699–711. 10.1111/bor.12025
- Bertran, P., Andrieux, E., Antoine, P. et al. 2017, Pleistocene involutions and patterned ground in France: Examples and analysis using a GIS database: Permafrost and Periglacial Processes, v. 28, no. 4, p. 710–725. 10.1002/ppp.1957
- Böhner, J., and Lehmkuhl, F., 2005, Environmental change modelling for Central and High Asia: Pleistocene, present and future scenarios: Boreas, v.34, no. 2, p. 220–231. 10.1080/03009480510012917
- Buylaert, J.P., Ghysels, G., Murray, A.S. et al. 2009, Optical dating of relict sand wedges and composite-wedge pseudomorphs in Flanders: Belgium. Boreas, v. 38, no. 1, p. 160–175. 10.1111/j.1502-3885.2008.00037.x
- Chang, X.L., Jin, H.J., He, R.X. et al. 2017, Evolution and changes of permafrost on the Qinghai-Tibet Plateau during the Late Quaternary: Sciences in Cold and Arid Regions, v. 9, no. 1. p. 1–19. 10.3724/SP.J.1226.2017.00xxx
- Cheng, J., Jiang, M.Z., Zan, L.H. et al. 2005a, Progress in Research on the Quaternary geology in the source area of the Yellow River (in Chinese): Geoscience, v. 19, no. 2, p. 239–246.
- Cheng, J., Zhang, X.J., Tian, M.Z. et al. 2005b, Ice-wedge casts showing climatic change since the Late Pleistocene in the source area of the Yellow River, north-east Tibet: Journal of Mountain Science, v. 2, no. 3, p, 193–201. 10.1007/BF02973192
- Cheng, J., Zhang, X.J., Tian, M.Z. et al. 2006, Ice-wedge casts discovered in the source area of the Yellow River, Northeast Tibetan Plateau and their palaeoclimatic implications (in Chinese): Quaternary Sciences, v. 26, no. 1, p. 92–98.
- Cui, Z.J., Yang, J.J., Zhang, W. et al. 2004, Discovery of a large area of ice-wedge networks in Ordos: Implications for the southern boundary of permafrost in North China as well as for the environment in the latest 20 ka: Chinese Science Bulletin, v. 49, no. 11, p, 1177–1184. 10.1360/03wd0211
- Frechen, M., Vliet-Lanoe, B.V., and Haute, P.V.D., 2001, The upper Pleistocene loess record at Harmignies/Belgium high resolution terrestrial archive of climate forcing: Palaeogeography, Palaeoclimatology, Palaeoecology, v.173, no. 3–4, p. 175–195. 10.1016/S0031-0182(01)00319-4
- French, H.M., 2018, The Periglacial Environment. 4th ed. Chichester, UK, and Hoboken, New Jersey, USA: John Wiley & Sons, p. 1–515.
- French, H.M., Demitroff, M., Forman, S.L. et al. 2007, A chronology of late-Pleistocene permafrost events in southern New Jersey, Eastern USA: Permafrost and Periglacial Processes, v. 18, no. 1, p. 49–59. 10.1002/ppp.572
- French, H.M., Vandenberghe, J., Jin, H.J., and He, R.X., 2021, Possible initial factors in the development of seasonal sand-wedge on the Ordos Plateau, North China: Sciences in Cold Arid Regions, v. 13, no. 2, p. 167–176. 10.3724/SP.J.1226.2021.20095
- Harris, S.A., and Jin, H.J., 2012. Tessellons and “sand wedges” on the Qinghai-Tibet Plateau and their palaeoenvironmental implications. Proceedings of the Tenth International Permafrost Conference, Salekhard. Volume I, 149–153.
- Harris, S.A., Jin, H.J., and He, R.X., 2016, Very large cryoturbation structures of Last Permafrost Maximum age at the foot of the Qilian Mountains (NE Tibet Plateau, China): A discussion: Permafrost and Periglacial Processes, v. 28, no. 4, p. 757–762. 10.1002/ppp.1942
- Harris, S.A., Jin, H.J., He, R.X. et al. 2018, Tessellons, topography, and glaciations on the Qinghai-Tibet Plateau: Sciences in Cold and Arid Regions, v. 10, no. 3, p. 187–206.
- He, R.X., Jin, H.J., French, H.M. et al 2021, Cryogenic wedges and cryoturbations on the Ordos Plateau in North China since 50 ka BP and their paleo-environmental implications: Permafrost and Periglacial Processes, v. 32, p. 231–247
- Heyman, J., Hattestrand, C., and Stroeven, A.P., 2008, Glacial geomorphology of the Bayan Har sector of the NE Tibet Plateau: Journal of Maps, v.4, no. 1, p. 42–62. 10.4113/jom.2008.96
- Heyman, J., Stroeven, A.P., Alexanderson, H. et al. 2009, Palaeoglaciation of Bayan Har Shan, Northeastern Tibet Plateau: Glacial geology indicates maximum extents limited to ice cap and ice field scales: Quaternary Science Review, v. 24, p. 710–727.
- Jin, H.J., Chang, X.L., and Wang, S.L., 2007, Evolution of permafrost on the Qinghai-Tibet Plateau since the end of the Pleistocene: Journal of Geophysical Research: Earth Surface, v.112, no.F2, p. F02S09. 10.1029/2006JF000521
- Jin, H.J., Jin, X.Y., He, R.X. et al. 2019, Evolution of permafrost in China during the last 20 ka: Science China Earth Sciences, v. 62, no. 8, p, 1181–1192. 10.1007/s11430-018-9272-0
- Jin, H.J., Vandenberghe, J., Luo, D.L. et al. 2020, Quaternary permafrost in China: A preliminary framework and some discussions: Quaternary, v. 3, no. 4, p. 32. 10.3390/quat3040032
- Ju, L.X., Wang, H.K., and Jiang, D.B., 2007, Simulation of the last glacial maximum climate over East Asia with a regional climate model nested in a general circulation model: Palaeogeography, Palaeoclimatology, Palaeoecology, v.248, no. 3–4, p. 376–390. 10.1016/j.palaeo.2006.12.012
- Lai, Z.P., 2006, Testing the use of an OSL standardised growth curve (SGC) for De determination on quartz from the Chinese Loess Plateau: Radiation Measurements, v.41, no. 1, p. 9–16. 10.1016/j.radmeas.2005.06.031
- Lai, Z.P., and Brückner, H., 2008, Effects of Feldspar contamination on equivalent dose and the shape of growth curve for OSL of Silt-Sized Quartz extracted from Chinese Loess: Geochronometria, v.30, no.–1, p. 49–53. 10.2478/v10003-008-0010-0
- Lai, Z.P., Brückner, H., Zöller, L. et al. 2007, Existence of a common growth curve for silt-sized quartz OSL of loess from different continents: Radiation Measurements, v. 42, no. 9, p. 1432–1440. 10.1016/j.radmeas.2007.08.006
- Lai, Z.P., Mischke, S., and Madsen, D., 2014, Paleoenvironmental implications of new OSL dates on the formation of the “Shell Bar” in the Qaidam Basin, northeastern Qinghai-Tibetan Plateau: Journal of Paleolimnology, v.51, no. 2, p. 197–210. 10.1007/s10933-013-9710-1
- Lai, Z.P., and Wintle, A.G., 2006, Locating the boundary between the Holocene and Pleistocene in Chinese loess using luminescence: Holocene, v.16, no. 6, p. 893–899. 10.1191/0959683606hol980rr
- Li, J., Sheng, Y., Wu, J.C. et al. 2016, Variations in the ground temperatures of permafrost in the two watersheds of the interior and eastern Qilian Mountains: Environmental Earth Sciences, v. 75, no. 6, p, 480. 10.1007/s12665-016-5330-1
- Luo, D.L., Jin, H.J., and Bense, V.F., 2019, Ground surface temperature and the detection of permafrost in the rugged topography on NE Qinghai-Tibet Plateau: Geoderma, v. 333, p. 57–68. 10.1016/j.geoderma.2018.07.011
- Luo, D.L., Liu, L., Jin, H.J. et al. 2018, Thermal regime of warm-dry permafrost in relation to ground surface temperature in the Source Areas of the Yangtze and Yellow Rivers on the Qinghai-Tibet Plateau, SW China: Science of the Total Environment, v. 618, p. 1033–1045. 10.1016/j.scitotenv.2017.09.083
- Luo, D.L., Liu, L., Jin, H.J. et al. 2020, Characteristics of ground surface temperature at Chalaping in the source area of the Yellow River, northeastern Tibetan Plateau: Agricultural and Forest Meteorology, v. 281, p. 107819. 10.1016/j.agrformet.2019.107819
- Mischke, S., Lai, Z.P., and Zhang, C.J., 2013, Re-assessment of the paleoclimate implications of the Shell Bar in the Qaidam Basin, China: Journal of Paleolimnology, v.51, no. 2, p. 179–195. 10.1007/s10933-012-9674-6
- Murray, A.S., and Wintle, A.G., 2000, Luminescence dating of quartz using an improved single-aliquot regenerative-dose protocol: Radiation Measurements, v.32, no. 1, p. 57–73. 10.1016/S1350-4487(99)00253-X
- Murray, A.S., and Wintle, A.G., 2003, The single aliquot regenerative dose protocol: Potential for improvements in reliability: Radiation Measurements, v.37, no. 4–5, p. 377–381. 10.1016/S1350-4487(03)00053-2
- Murton, J.B., 2013, Permafrost and periglacial features. Ice wedges and ice-wedge casts, Encyclopedia of Quaternary Science, Second, Elsevier, Amsterdam, p. 436–451.
- Murton, J.B., and Kostrup, E., 2003, Ice-wedge casts as indicators of palaeotemperatures: Precise proxy or wishful thinking?: Progress in Physical Geography, v. 27, no. 2, p. 155–170. 10.1191/0309133303pp365ra
- Murton, J.B., Worsley, P., and Gozdzik, J., 2000, Sand veins and wedges in cold Aeolian environments: Quaternary Science Reviews, v. 19, no. 9, p. 899–922. 10.1016/S0277-3791(99)00045-1
- Pan, B.T., and Chen, F.H., 1997, Permafrost evolution in the northeastern Qinghai-Tibetan Plateau during the last 150000 years (In Chinese): Journal of Glaciology and Geocryology, v. 19, p. 124–132.
- Pan, B.T., and Xu, S.Y., 1989, Discussion on the quaternary environmental evolution in the Eastern regions of Qinghai-Xizang Plateau (in Chinese): Chinese Science Bulletin, v. 34, no. 7, p. 534–536.
- Remillard, A.M., Hetu, B., Bernatchez, P. et al. 2015, Chronology and palaeoenvironmental implications of the ice-wedge pseudomorphs and composite-wedge casts on the Magdalen Islands (eastern Canada): Boreas, v. 44, no. 4, p. 658–675. 10.1111/bor.12125
- Roberts, H.M., 2007, Assessing the effectiveness of the double-SAR protocol in isolating a luminescence signal dominated by quartz: Radiation Measurements, v.42, no. 10, p. 1627–1636. 10.1016/j.radmeas.2007.09.010
- Roberts, H.M., and Duller, G.A.T., 2004, Standardised growth curves for optical dating of sediment using multiple-grain aliquots: Radiation Measurements, v.38, no. 2, p. 241–252. 10.1016/j.radmeas.2003.10.001
- Romanovskii, N.N., 1977, Formation of polygonal-wedge structures: Nauka (Science) Press, Novosibirsk, v. 215, p. 70–85.
- Shen, C.M., and Tang, L.Y., 1996, Vegetation and climate during the last 250000 years in Zoîgé Plateau (in Chinese): Acta Micropalaeontol Sinica, v. 13, p. 401–406.
- Shi, Y., 2006, Quaternary Glaciers in China and Environmental Changes (in Chinese), Science Press, Beijing, China, p. 134–138.
- Shi, Y.F., Zheng, B., Li, S.X. et al. 1995, Studies on altitude and climate environment in the middle and east parts of Tibetan Plateau during Quaternary maximum glaciation (in Chinese): Journal of Glaciol and Geocryology, v. 17, p. 99–112.
- Singarayer, J.S., and Bailey, R.M., 2003, Further investigations of the quartz optically stimulated luminescence components using linear modulation: Radiation Measurements, v.37, no. 4–5, p. 451–458. 10.1016/S1350-4487(03)00062-3
- Stadelmaier, K.H., Ludwig, P., Bertran, P. et al. 2021, A new perspective on permafrost boundaries in France during the last glacial maximum: Climate of the Past, v. 17, no. 6, p. 2559–2576. 10.5194/cp-17-2559-2021
- Vandenberghe, J., 1983. Ice-wedge casts and involutions as permafrost indicators and their stratigraphic position in the Weichselian. Proceedings of the 4th International Conference on Permafrost, Fairbanks. National Academy Press, Washington DC, pp. 1298–1302.
- Vandenberghe J. 1992, Cryoturbations: a sediment structural analysis: Permafrost and Periglacial Processes, v. 3, no. 4, p. 343–352.
- Vandenberghe, J., Cui, Z., Zhao, L., and Zhang, W., 2004, Thermal-contraction-crack networks as evidence for Late-Pleistocene Permafrost in Inner Mongolia, China: Permafrost and Periglacial Processes, v. 15, no. 1, p. 652–666. 10.1002/ppp.476
- Vandenberghe, J., Cui, Z., Zhao, L., and Zhang, W., 2004, Thermal-contraction-crack networks as evidence for Late Pleistocene permafrost in Inner Mongolia, China: Permafrost and Periglacial Processes, v. 15, p. 21–29.
- Vandenberghe, J., French, H.M., Gorbunov, A. et al. 2014, The Last Permafrost Maximum (LPM) map of Northern Hemisphere: Permafrost extent and mean annual air temperatures, 25-17 ka B.P: Boreas, v. 43, no. 3, p, 652–666. 10.1111/bor.12070
- Vandenberghe, J., French, H.M., Jin, H.J. et al. 2019, The extent of permafrost during the Last Permafrost Maximum (LPM) on the Ordos Plateau, North China: Quaternary Science Reviews, v. 214, p. 87–97. 10.1016/j.quascirev.2019.04.019
- Vandenberghe, J., French, H.M., Jin, H.J. et al. 2020, The impact of latitude and latitude on the extent of permafrost during the last Permafrost Maximum (LPM) in North China: Geomorphology, v. 350, p. 106909. 10.1016/j.geomorph.2019.106909
- Vandenberghe, J., Wang, X., and Vandenberghe, D., 2016, Very large cryoturbation structures of last maximum permafrost age at the foot of the Qilian Mountain (NE Tibet Plateau, China): Permafrost and Periglacial Processes, v.27, no. 1, p. 138–143. 10.1002/ppp.1847
- Vandenberghe, J., Wang, X., and Vandenberghe, D., 2017, Comment on “Very large cryoturbation structures of Last Maximum Permafrost age at the foot of the Qilian Mountain (NE Tibet Plateau, China): A discussion” by Stuart A: Harris, Huijun Jin and Ruixia He in PPP. Permafrost and Periglacial Processes, v.28, no. 4, p. 763–766. 10.1002/ppp.1947
- Van Huissteden, J., Vandenberghe, J., and Pollard, D., 2003, Palaeotemperature reconstructions of the European permafrost zone during marine oxygen isotope stage 3 compared with climate model results: Journal of Quaternary Science, v. 18, no. 18, p. 453–464. 10.1002/jqs.766
- Wang, Y.X., Chen, T.Y., E, C.Y. et al 2018, Quartz OSL and K-feldspar post-IR IRSL dating of loess in the Huangshui river valley, northeastern Tibetan plateau: Aeolian Research, v.33, p.23–32. 10.1016/j.aeolia.2018.04.002
- Wang, S.L., and Luo, X.R., 1991, The distributive characteristics of frozen ground in the east of Qinghai-Xizang Plateau (in Chinese): Journal of Glaciology and Geocryology, v. 4, no. 2, p. 131–140.
- Wang, X.Y., Vandenberghe, D., Yi, S.W. et al. 2013, Late Quaternary paleoclimatic and geomorphological evolution at the interface between the Menyuan basin and the Qilian Mountains, northeastern Tibetan Plateau (in Chinese): Quaternary Research, v. 80, no. 3, p. 534–544. 10.1016/j.yqres.2013.08.004
- Wolfe, S.A., Morse, P.D., Neudorf, C. et al. 2018, Contemporary sand wedge development in seasonally frozen ground and paleoenvironmental implications: Geomorphology, v. 308, p. 215–219. 10.1016/j.geomorph.2018.02.015
- Wu, H., Li, Q., Yu, Y. et al. 2019, Quantitative climate reconstruction of the Last Glacial Maximum in China: Science China Earth Sciences, v. 62, no. 8, p. 1269–1278. 10.1007/s11430-018-9338-3
- Yu, S.Y., Hou, Z.F., Chen, X.X. et al 2020, Extreme flooding of the lower Yellow River near the Northgrippian-Meghalayan boundary: Evidence from the Shilipu archaeological site in southwestern Shandong Province, China: Geomorphology, v. 350, p. 1–12.
- Zhao, L., Jin, H.J., Li, C.C. et al. 2013, The extent of permafrost in China during the local Last Glacial Maximum (LLGM): Boreas, v. 43, no. 3, p. 688–698. 10.1111/bor.12049
- Zheng, B.X., 1990, The glacier environment and its changes since the Last Glaciation in West China (In Chinese): Quaternary Sciences, v. 10, p. 101–110.
- Zhou, Y.W., Qiu, G.Q., Guo, D.X. et al. 2000, Geocryology in China. Beijing, Science Press, p. 1–450.