ABSTRACT
Enterococci are a group of significant disease-causing bacteria, which have developed resistance to several conventionally used antibiotics. Of the 67 confirmed Enterococcus species from Kidd’s Beach, 40 (59.7%) were E. faecium, 19 (28.4%) were E. faecalis. The highest level of resistance was observed against rifampicin (83.6%) followed by erythromycin (64.2%), tetracycline (52.2%), linezolid (46.3%), tetracycline (46.3%), and vancomycin (32.8%). Ninety-six per cent of the Enterococcus spp. was found to be multi-drug resistant. MAR indices vary from 0.3 to 0.9. Two virulence determinants (ace and gelE) were detected and six resistance determinants were identified as follows: ermB (19%), tetM (30%), tetL (19%), gyrA (13%), ampC (1.5%) and Van C2/3 (4.5%). The presence of enterococci (E. faecium and E. faecalis) in Kidd’s Beach waters harbouring virulence genes that facilitate multiple antibiotic resistance signifies a possible health threat for beach goers.
Introduction
Antibiotic-resistant bacteria have been detected in several environmental sources comprising untreated and treated wastewater [Citation1,Citation2], rivers [Citation3] and coastal water [Citation4,Citation5]. The incidence of multidrug-resistant bacteria has been increasing at an alarming rate [Citation6–11], causing high rates of treatment failure [Citation12,Citation13], increased incidence and severity of infections [Citation14,Citation15], and ultimately, increased mortality [Citation16]. If unabated, it could instigate a ‘post-antibiotic era’, where infectious diseases could become incurable [Citation17]. There would then be serious socio-economic consequences for families and world economies. Patients would have to be hospitalised for longer periods at a time leading to loss of working hours, and there would be the loss of skilled labour to premature deaths [Citation18].
Enterococci species are among pathogens known to be intrinsically resistant to a wide range of antibiotics including beta-lactams, aminoglycosides and ampicillins [Citation19,Citation20]. This bacterium is one of the microflora inhabiting the gastrointestinal tract of warm-blooded animals, including human beings [Citation21]. The presence of enterococci in the aquatic environment therefore indicates faecal contamination [Citation22,Citation23]. Although microbial pollution of waterbodies can be linked to diffuse sources of faecal pollution including livestock, wildlife and municipal wastewater effluents [Citation24], the presence of antibiotic-resistant (AR) enterococci in marine coastal environments has been linked to human faecal pollution [Citation25,Citation26]. Vallero et al. [Citation27] reported that over 90% of bacterial strains recovered from coastal water are resistant to at least three antibiotics, and about 20% are resilient to at least five antibiotics.
Waterborne Enterococcus species have attracted particular clinical interest because they are labelled as opportunistic pathogens that cause nosocomial infections [Citation28–30]. Reports by Fisher and Phillips [Citation31] and Mendes et al. [Citation32] have shown that among Enterococcus species, E. faecium and E. faecalis are responsible for high levels of infection in human beings and animals, causing bacteraemia, endocarditis and pyelonephritis. Part of this observed virulence and antimicrobial resistance has been attributed to the vulnerability of enterococci species to acquire transposomes carrying varying genetic elements [Citation33]. Pathogenic Enterococcus species also aid the pathogenicity of other bacteria through interspecies conjugation of genetic elements required for colonisation of the host, breaching of host defence mechanisms and production of toxins for induction of inflammation [Citation34,Citation35]. In enterococci, the presence of genes encoding virulence factors including enterococcal aggregation substance (asa1), cytolysin (cylA), surface protein (esp), collagen-binding protein (ace), endocarditis antigen (efaA), hyaluronidase (hyl) and gelatinase (gelE) has been determined [Citation36,Citation37]. Although the virulence and antimicrobial resistance of Enterococcus species has been reported in many settings, there remains an information gap on the occurrence, virulence and antimicrobial resistance patterns of these species in recreational beaches of several countries. Thus, the aim of this research was to evaluate the virulence factors and antimicrobial susceptibility patterns of Enterococcus species recovered from the coastal waters of a resort beach in the Eastern Cape Province of South Africa.
Materials and methods
Description of study area and collection of water samples
Six sampling points were identified along Kidd’s Beach which is located in Buffalo City Metropolitan Municipality in the Eastern Cape Province of South Africa. Sampling points were coded as follows: KP1, KP2 and KP3 (all beach water); KP4 and KP5 (canal water), and KP6, pool water (). Presumed causes of faecal contamination affecting the sampling points included river discharge, coastal birds, swimming, surfing, animal inputs, and municipal sewage discharge. The sewage discharges were more pronounced at KP3 and KP6. Water samples were collected monthly in sterile 1 L bottles at each of the six sites between November 2017 and April 2018 and transported to laboratory in cooler boxes containing ice and processed within 6 h of collection.
Isolation of presumptive Enterococcus colonies
Water samples were subjected to 10-fold serial dilution after which 100 mL aliquots of each dilution were filtered using nitrocellulose membrane filters (0.45-μm pore size, Millipore, Ireland) following the membrane filtration technique for the isolation and enumeration of faecal coliforms according to standard methods for microbiological water analysis [Citation38]. The filters were aseptically transferred grid-up onto Bile Aesculin Azide agar and gently pressed to be totally in touch with the media surface and incubated at 37°C for 48 h. Following incubation, a total of 409 presumptive Enterococcus colonies presenting a black to dark brown colouration were purified by sub-culturing onto nutrient agar till axenic cultures were obtained. Lone standing colonies from the axenic culture plates were transferred onto 25% glycerol and stored at 4°C till further analysis.
DNA extraction and PCR confirmation of presumptive Enterococcus isolates
Total genomic DNA was extracted using the boiling method as described by Magueri et al. [Citation39]. Confirmative PCR identification of enterococci was done based on the method described by Ke et al. [Citation40] and Adeniji et al. [Citation41] using genus-specific primers targeting the tuf-gene using E. faecalis (DSM 20,478) as a positive control.
Screening for virulence genes
PCR confirmed enterococci strains were screened for six virulence genes formerly described by Mannu et al. [Citation42] and Vankerckhoven et al. [Citation43] using gene-specific primer sets listed in . Multiplex PCR was used with the following cycling conditions: denaturation at 94°C/3 min; 35 cycles of denaturation at 93°C/60 s, annealing at 50°C/60 s, elongation at 72°C/60 s, and a final extension step at 72°C for 10 min. The products were resolved in 2% agarose gel and visualised under an Ultraviolet (UV) transilluminator as already described.
Table 1. Virulence genes primers
Antibiotic susceptibility testing
Antimicrobial susceptibility testing was carried out using disk diffusion method on Muller Hinton agar (MHA) following the method of Kirby-Bauer according to the recommendation of CLSI [Citation44]. Four to five (4–5) colonies at log phase were picked with a sterile inoculating loop, transferred into test tubes containing 5 mL of sterile 0.85% normal saline and lightly vortexed. The bacterial suspensions were adjusted to 0.5 McFarland standards as described by Panda et al. [Citation45]. Mueller Hinton agar plates were inoculated by uniformly spread plating 100 µl of the bacterial suspensions over the surface of the agar, and allowing the plates to dry before antibiotic discs were placed on them. Each Enterococcus isolate was tested against a panel of 11 antibiotics (Mast Diagnostics, UK) including ampicillin (AP, 10 μg), vancomycin (VA, 30 μg), linezolid (LZD, 30 μg), ciprofloxacin (CIP, 5 μg), levofloxacin (LEV, 5 μg), norfloxacin (NOR, 10 μg), nitrofurantoin (NIT, 300 μg), chloramphenicol (C, 30 μg), tetracycline (T, 30 μg), erythromycin (E, 15 μg) and rifampicin (RP, 5 μg). Subsequently, the plates were incubated at 37°C for 24 h and zones of inhibition were measured and classified as either susceptible (S), intermediate (I), and resistant (R), using the CLSI [Citation43] chart for interpretation.
Determination of multiple antibiotic-resistant phenotypes (MARPs)
Following the method of Wose et al. [Citation46], MARPs were generated for each isolate and sample site. The multiple antibiotic resistance index (MARI) for each isolate was done using the mathematical expression of Blasco et al. [Citation47] as follows:
(MAR) index = c/d
where c is the number of antibiotics that the strain was resistant against and d is the aggregate number of antibiotics against which the individual strains was tested.
The antibiotic resistance index (ARI) was calculated for each sampling site using the formula described by Kuperman [Citation48], mathematically expressed as:
ARI = A/B(Z),
where A is the total antibiotic resistance score of all strains from the sample, B is the number of antibiotics and Z is the number of strains from the sample. Thereafter, the prevalence of relevant antibiotic resistance genes was determined using PCR.
Detection of antibiotic-resistant genes
The occurrence of antibiotic resistance genes among those that showed resistance to the antibiotics was determined using gene-specific primers synthesised by Inqaba Biotech (Pretoria, South Africa). PCR amplification was carried out in 25 µL reaction mixtures consisting of 12.5 µL of PCR master mix (Thermo Scientific, (EU) Lithuania), 0.5 µL of each primer (Inqaba Biotech, SA), 5 µL of template DNA and 6.5 µL of nuclease-free water. The primer sets, targeted genes, PCR conditions, antibiotic family and base pairs are all listed in .
Table 2. Oligonucleotide primers used to identify resistance genes
Data analysis
IBM SPSS Statistics version 25 was employed for data analysis in this work, and the significant levels were set at p < 0.05. Significance of difference in resistance among the isolates with respect to different sampling locations was determined by one-way analysis of variance (ANOVA).
Results
PCR confirmation and occurrence of Enterococcus isolates from six sampling sites
Presumptive Enterococcus species were identified in counts ranging from 64 to 168 CFU/100 mL of water samples at the six sampling sites. In total, 67 isolates were obtained from the Kidd’s Beach coastal waters after PCR confirmation of the isolates. PCR speciation of the isolates showed that 19 (28.4%) were Enterococcus faecalis and 40 (59.7%) were Enterococcus faecium. shows the presumptive enterococci counts obtained at each sampling site. shows the gel electrophoresis picture for the PCR amplification of tuf gene in the Enterococcus species.
Phenotypic pattern of resistance to antibiotics
High levels of resistance were detected against rifampicin (83.6%), erythromycin (64.2%) and tetracycline (52.2%). The isolates also showed resistance to other antibiotics as follows: vancomycin (46.3%), linezolid (49.3%), ampicillin (44.8%) and ciprofloxacin (35.8%). shows the full spectrum of the observed phenotypic resistance and susceptibility patterns against the tested antibiotics.
AP- Ampicillin 10 μg, VA- Vancomycin, 30 μg, LZD – Linezolid 30 μg, CIP – ciprofloxacin 5 μg, LEV- levofloxacin 5 μg, NOR- Norfloxacin 10 μg, NIT- nitrofurantoin 300 μg C – chloramphenicol 30 μg, T- tetracycline 30 μg, E – erythromycin 15 μg and RP- rifampicin 5 μg.
shows the antimicrobials, resistance patterns and number observed across different sampling locations with respect to the prevalence of multiple antibiotic resistance phenotypes (MARPs) of the Enterococcus isolates.
Table 3. Patterns of multiple antibiotics-resistance phenotypes (MARPs) of Enterococcus species for each sampling location
Identification of virulence genes
The 67 confirmed enterococci isolates were further analysed for the presence of the targeted virulence genes gelE, and ace gene. A total of 11 strains were positive for the ace gene and 13 for the gelE gene (). The gel showing the PCR amplification product of the virulence genes (ace gene and gelE gene) is shown in .
Table 4. Frequency of virulence genes among E. faecalis and E. faecium isolated from coastal water
Figure 5. A gel electrophoresis showing the distribution of virulence genes among E. faecalis and E. faecium.

Identification of antimicrobial resistance genes from confirmed Enterococcus isolates
The isolates were screened for the eleven (11) genes coding for resistance to antimicrobials in five families (glycopeptides, beta-lactams, tetracycline, flouroquinnone and macrolides). Among the genes examined for vancomycin resistance, vanA and vanB were not detected, but vanC2/3 genes were found. Not all the strains that were phenotypically resistant to vancomycin were positive for vanA, vanB or vanC1. But, shows that 6 (9%) and 3 (4.5%) were positive for ermB and vanC2/3 genes, respectively. Thirteen per cent (13%) of the isolates possessed the gyrA gene, 1.5% harboured the ampC gene, whereas none of the isolates harboured either the blaTEM or blaZ gene. Among the tetracycline-resistance genes; tetK, tetL and tetM, the tetM gene (n = 20, 30%) was the predominant gene identified, followed by the tetL gene. None of the isolates harboured the tetK gene. show images of Gel electrophoresis of detected antimicrobial resistance genes.
Table 5. Frequency of resistance genes detected among the enterococcus isolates
Figure 6. A gel electrophoresis showing the tetracycline resistance tetM gene
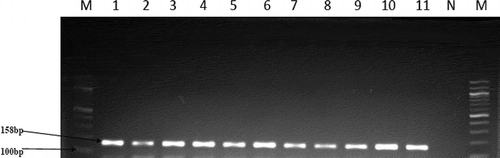
Figure 7. A gel electrophoresis showing the resistance determinants of ermB and ampC gene
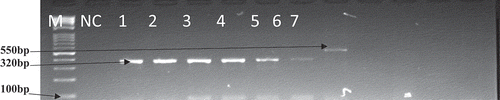
Figure 8. A gel electrophoresis showing the tetL gene

Discussion
The presence of faecal indicator bacteria such as enterococci in recreational water may be indicative of the presence of entero-pathogenic microorganisms such as viruses and some pathogenic bacterial species, including those of the genus Enterococcus. In the present study, the presence of Enterococcus species was confirmed by PCR techniques targeting the tuf- gene. Among the detected virulence genes was the ace gene which encodes a collagen adhesion protein active in mediating adherence to host cell epithelia, a virulence strategy mostly associated with infections caused by E. faecalis [Citation49]. Antibodies against ace have been established on many occasions in serum from patients with endocarditis [Citation50]. Some reports have suggested that genes for virulence factors may be more common in E. faecalis than in E. faecium [Citation51,Citation52], which confirms the findings of the present study. Among the E. faecalis isolates, the most frequently identified virulence gene was gelE (15%), followed by ace (13. 4%). Wade et al. [Citation53] reported a resilient affiliation between enterococci and illness among swimmers in coastal waters. In addition, out of the confirmed Enterococcus strains in this study, 96% were resistant to at least three or more of the antimicrobial agents tested, which corroborates the findings of Łuczkiewicz et al. [Citation54] who also reported resistant enterococci species from surface water samples. From previous studies, Garido et al. [Citation55] highlighted that strains that show resistance to linezolid may also display co-resistance to more antibiotics including chloramphenicol, gentamicin, vancomycin, fluoroquinolones, and macrolides. These authors [Citation55] also reported a high percentage of E. faecium and E. faecalis isolates that showed resistance to tetracycline which was very similar to our results. In another study conducted by Kimiran-Erdem et al. [Citation56], 3% of their seawater isolates showed resistance to chloramphenicol, whereas, chloramphenicol resistance in this study was observed to be 19.9%. It was observed that geographical factors could be responsible for the variances in the resistance.
The incidence of antimicrobial-resistant microbes in recreational waters is a major public health problem [Citation57]. Human beings are most likely to be exposed to antibiotic resistance bacteria in coastal water environments that are polluted with faecal wastes, especially during various recreational activities [Citation58,Citation59]. The high level of resistance to erythromycin, rifampicin, tetracycline, linezolid and vancomycin observed could be attributable to the influence of domestic wastes, surface runoff and several human activities in the sea catchment area (). The unrestrained use of antibiotics in the treatment of animals and their integration in animal feed has also been presumed to account considerably for the upsurge in antimicrobial resistance in pathogenic bacterial isolates [Citation60,Citation61]. Survival of strains with higher rates of mutation (e.g., bacteria with hypermutator phenotypes) may be a reason why antimicrobials are found in the environment, such as hospitals or intensive farm settings [Citation62–64]. The present study found a high level of resistance to ciprofloxacin (13%). This agrees with the report of Alipour et al. [Citation65] who found ciprofloxacin resistance to be 30% among enterococci isolated from river and coastal water. It has been suggested that although enterococci exhibit low levels of intrinsic resistance to quinolones, they can nevertheless develop high-level resistance through various mechanisms such as mutations in genes encoding the target site of fluoroquinolone (DNA gyrase and topoisomerase IV), over-expression of efflux pumps that extrude the drug from the cell, and protection of the FQ target site by a protein designated Qnr family [Citation55,Citation66]. Mutations have also been described in the target genes gyrA and parC in E. faecium and E. faecalis [Citation67] leading to continuously increasing levels of resistance [Citation68]. Among some of the most challenging enterococci to handle are the vancomycin-resistant enterococci (VRE) and their detection in the aquatic environments has been documented [Citation37,Citation69,Citation70]. VRE represented 8% (18 of 227) of enterococci isolates from some public beaches in Washington and California [Citation71]. Among the genes examined for vancomycin resistance in this study, vanA, vanB and vanC1 were not detected, and vanC2/3 (n = 3) genes were found in only 4.5% of the isolates. In northern Greece, the vanC2/C3 type of VRE was similarly recovered from beach waters. This indicates that vanC type VRE may be extensively disseminated in aquatic milieus, such as rivers and beach areas [Citation72]. VRE carrying vanA and vanB are the most essential genotype relating to nosocomial infections [Citation73], though these were not detected in Kidd’s Beach water. Several studies have shown that the rigorous use of avoparcin as a growth promoter in animals’ nutrition can contribute to a proliferation in VRE [Citation74–76].
The multiple antibiotic resistance (MAR) index shown on is a good risk assessment tool and the value of the MAR index of 0.2 has been applied to differentiate low- and high-risk areas where antibiotics are often used, as Riaz et al. [Citation77] and Christopher et al. [Citation78] have reported. The ARI and MARI in all sampling points on the beach were found to be greater than the 0.2 threshold value, indicating the possible excessive use of antibiotics and greater exposure to antibiotics which may cause high health risk to the water bathers. The ARI in the present study ranged from 0.45 to 0.64. The highest ARI for the tested sampling points were 0.6 at KP3 and 0.64 at KP5, approximately three times the 0.2 limit. This shows that the loads of antibiotics being discharged into these locations are greater than at other points. The increase in the threshold values could be as a result of the socio-economic factors associated with each sampling point. KP1 and KP2 are the major accessible places where swimming, surfing and fishing take place along the beach shore. KP1 and KP2 were also exposed to runoff from the canal during the downpour, possibly increasing the anthropogenic pollution in the area. KP3 and KP6 are very close to the point where municipal sewage is discharged into the ocean. The various socio-economic activities near the sampling sites suggest that there is a high risk of pollution in using the coastal water for recreation. Incidence of antibiotic-resistant bacteria in a given milieu may be a signal that the area is polluted with antimicrobials [Citation79]. For instance, Al-Bahry et al. [Citation80] reported that the major reasons responsible for the marine environment to be contaminated were inappropriate and excessive use of antimicrobial drugs by human beings, including in animal husbandry. Three most important reasons were reported for the emergence of MDR enterococci: baseline intrinsic resistance to numerous antimicrobial agents, acquired resistance, and the transferability of resistance [Citation81].
Generally, the one-way ANOVA showed that ampicillin, vancomycin, linezolid, levofloxacin, rifampicin and norfloxacin were significantly different in their effect against the isolates from all sites (P < 0.05); whereas the resistance profile of the isolates against nitrofurantoin, ciprofloxacin, tetracycline, chloramphenicol and erythromycin were not significantly different (P > 0.05). In the present study, all isolates were screened for β-lactams ampC, blaTEM and blaZ genes. Ampicillin resistance gene ampC was detected at a very low rate (). The low resistance to these β-lactam antibiotics was not astounding, for ampicillin is one of the most frequently used antibiotics for human and animal diseases [Citation82]. The ampC gene encoding β-lactams has been identified in the bacteriological strains from wastewater, surface water and even from drinking water films [Citation83].
Tetracycline has led to widely disseminated tetracycline-resistant bacteria [Citation84,Citation85]. Currently, over 20 different tet genes have been reported, most of which are harboured in mobile genetic elements [Citation86]. The tetM gene is implicated in ribosomal protection while tetL codifies an efflux pump; and both of them are commonly reported to be related to tetracycline resistance in enterococci strains [Citation87,Citation88]. In line with previous studies, results of the present research have also shown the presence of tetL and tetM genes encoding tetracycline resistance and were detected in 19% and 30%, respectively, of the total isolates in the study. Among enterococci species, tetM is the most frequently tetracycline-resistance gene encountered. It is most generally situated in the bacterial chromosome and has been found to be linked with conjugative transposons related to the Tn916/Tn1545 family [Citation89,Citation90]. Remarkably, some Enterococcus strains exhibiting resistance to tetracycline were negative for tetM and tetL indicating that these may harbour other tet determinants.
In the macrolide class, erythromycin is a common drug and its mechanisms of resistance have been recognised as target enzymes, of which more than 40 erm genes have been discovered [Citation91–93]. ermB genes happen to be one of the four main classes of erm resistance determinants that are correlated with disease-causing microorganism [Citation91]. In this study, 19% of the isolates showed the occurrence of the ermB gene signifying that the macrolides resistance in the Enterococcus species identified from Kidd’s Beach is mainly correlated with the presence of the ermB gene. Owing to the clinical importance of ciprofloxacin, Magiorakros et al. [Citation94] mentioned it as one of the means considered in MDR assessment tables. In the present study, 13% of the ciprofloxacin-resistant strains showed the gyrA gene. In another study, Torell et al. [Citation95] reported that 17% of ciprofloxacin-resistant strains contained the gyrA gene.
Conclusion and recommendation
This study presents the first documented evidence for multi-drug resistance and virulence characteristics among Enterococcus spp. at Kidd’s Beach. The evaluation of Enterococcus spp. in the coastal water samples clearly indicates the faecal contamination, which is resistant to various types of antibiotics in coastal waters and may cause illness among swimmers. To track the specific sources of each isolate found at the beach, it would be necessary to map the key determinants of antibiotic resistance in the entire municipality. Moreover, apprehension of the virulence features of circulating Enterococcus strains may help to comprehend the complex pathogenic processes of these opportunistic microorganisms. It has not been determined whether these bacteria constitute a threat to bathers through quantitative microbial risk assessment. The existence of antibiotic-resistant enterococci with virulence traits evaluated in this study suggests that these factors may play a causative role in enterococcal infections. The risk should be subject to regular monitoring to avert the threat of developing systemic disease. The occurrence of antibiotic-resistant Enterococcus spp. in coastal waters poses a high risk to public health.
Acknowledgments
The authors are grateful to the South African Medical Research Council (SAMRC) and the University of Fort Hare for funding this research.
Disclosure statement
No potential conflict of interest was reported by the authors.
References
- Anderson, M.E. and Sobsey, M.D., 2006, Detection and occurrence of antimicrobially resistant E. coli in groundwater on or near swine farms in eastern North Carolina. Water Science and Technology 54(3), 211–218.
- Shehabi, A.A., Odeh, J.F., and Fayyad, M., 2006, Characterization of antimicrobial resistance and class 1 integrons found in Escherichia coli isolates from human stools and drinking water sources in Jordan. Journal of Chemotherapy 18(5), 468–472.
- Cernat, R., Lazar, V., Balotescu, C., Cotar, A., Coipan, E., and Cojocam, C., 2002a, Distribution and diversity of conjugative plasmids along some multiple antibiotic resistant E. coli strains isolated from river waters. Bacteriologia, Virusologia, Parazitologia, Epidemiologia 47(3–4), 147–153.
- Boehm, A.B., Fuhrman, J.A., Mrše, R.D., and Grant, S.B., 2003, Tiered approach for identification of a human fecal pollution source at a recreational beach: Case study at Avalon Bay, Catalina Island, California. Environmental Science & Technology 37(4), 673–680.
- Buschmann, A.H., Tomova, A., Lopez, A., Maldonado, M.A., Henríquez, L.A., Ivanova, L., Moy, F., Godfrey, H.P., and Cabello, F.C., 2012, Salmon aquaculture and antimicrobial resistance in the marine environment. PloS ONE 7(8), e42724.
- Gould, I.M. and Bal, A.M., 2013, New antibiotic agents in the pipeline and how they can help overcome microbial resistance. Virulence 4(2), 185–191.
- Golkar, Z., Bagasra, O., and Pace, D.G., 2014, Bacteriophage therapy: A potential solution for the antibiotic resistance crisis. The Journal of Infection in Developing Countries 8(2), 129–136.
- Ventola, C.L., 2015, The antibiotic resistance crisis: Part 1: Causes and threats. P & T: A Peer-Reviewed Journal for Formulary Management 40(4), 277–283.
- Frieri, M., Kumar, K., and Boutin, A., 2017, Antibiotic resistance. Journal of Infection and Public Health 10(4), 369–378.
- Sengupta, S., Chattopadhyay, M.K., and Grossart, H.P., 2013, The multifaceted roles of antibiotics and antibiotic resistance in nature. Frontiers in Microbiology 4, 47.
- Lushniak, B.D., 2014, Antibiotic resistance: A public health crisis. Public Health Reports 129(4), 314–316.
- Coenen, S., Francis, N., Kelly, M., Hood, K., Nuttall, J., Little, P., Verheij, T.J., Melbye, H., Goossens, H., and Butler, C.C. and GRACE Project Group, 2013, Are patient views about antibiotics related to clinician perceptions, management and outcome? A multi-country study in outpatients with acute cough. PLoS ONE 8(10), e76691.
- Tanwar, J., Das, S., Fatima, Z., and Hameed, S., 2014, Multidrug resistance: An emerging crisis. Interdisciplinary Perspectives on Infectious Diseases 1–7.
- Llor, C. and Bjerrum, L., 2014, Antimicrobial resistance: Risk associated with antibiotic overuse and initiatives to reduce the problem. Therapeutic Advances in Drug Safety 5(6), 229–241.
- Van den Honert, M.S., Gouws, P.A., and Hoffman, L.C., 2018, Importance and implications of antibiotic resistance development in livestock and wildlife farming in South Africa: A Review. South African Journal of Animal Science 48(3), 401–412.
- Khameneh, B., Diab, R., Ghazvini, K., and Bazzaz, B.S.F., 2016, Breakthroughs in bacterial resistance mechanisms and the potential ways to combat them. Microbial Pathogenesis 95, 32–42.
- Guerrero-Ramos, E., Cordero, J., Molina-González, D., Poeta, P., Igrejas, G., Alonso-Calleja, C., and Capita, R., 2016, Antimicrobial resistance and virulence genes in enterococci from wild game meat in Spain. Food Microbiology 53, 156–164.
- Hennessy, T.W., Marcus, R., Deneen, V., Reddy, S., Vugia, D., Townes, J., Bardsley, M., Swerdlow, D., and Angulo, F.J. and Emerging Infections Program FoodNet Working Group, 2004, Survey of physician diagnostic practices for patients with acute diarrhea: Clinical and public health implications. Clinical Infectious Diseases 38, S203–S211.
- Huycke, M.M., Sahm, D.F., and Gilmore, M.S., 1998, Multiple-drug resistant enterococci: The nature of the problem and an agenda for the future. Emerging Infectious Diseases 4(2), 239.
- Correll, C.U., Solmi, M., Veronese, N., Bortolato, B., Rosson, S., Santonastaso, P., Thapa‐Chhetri, N., Fornaro, M., Gallicchio, D., Collantoni, E., and Pigato, G., 2017, Prevalence, incidence and mortality from cardiovascular disease in patients with pooled and specific severe mental illness: A large‐scale meta‐analysis of 3,211,768 patients and 113,383,368 controls. World Psychiatry 16(2), 163–180.
- Enayati, M., Sadeghi, J., Nahaei, M.R., Aghazadeh, M., Pourshafie, M.R., and Talebi, M., 2015, Virulence and antimicrobial resistance of Enterococcus faecium isolated from water samples. Letters in Applied Microbiology 61(4), 339–345.
- Lanthier, M., Scott, A., Zhang, Y., Cloutier, M., Durie, D., Henderson, V.C., Wilkes, G., Lapen, D.R., and Topp, E., 2011, Distribution of selected virulence genes and antibiotic resistance in Enterococcus species isolated from the South Nation River drainage basin, Ontario, Canada. Journal of Applied Microbiology 110(2), 407–421.
- Leclercq, R., Oberlï, K., Cattoir, V., and Petit, F., 2013, Changes in enterococcal populations and related antibiotic resistance along a medical center-wastewater treatment plant-river continuum. Applied and Environmental Microbiology 79(7), 2428–2434.
- Byappanahalli, M.N., Nevers, M.B., Korajkic, A., Staley, Z.R., and Harwood, V.J., 2012, Enterococci in the environment. Microbiology and Molecular Biology Reviews 76(4), 685–706.
- Di Cesare, A., Luna, G.M., Vignaroli, C., Pasquaroli, S., Tota, S., Paroncini, P., and Biavasco, F., 2013, Aquaculture can promote the presence and spread of antibiotic-resistant Enterococci in marine sediments. PLoS ONE 8(4), e62838.
- Traversa, D., Di Regalbono, A.F., Di Cesare, A., La Torre, F., Drake, J., and Pietrobelli, M., 2014, Environmental contamination by canine geohelminths. Parasites & Vectors 7(1), 67.
- Vallero, M.V., Sipma, J., Annachhatre, A., Lens, P.N., and Pol, L.H., 2003, Biotechnological treatment of sulfur-containing wastewaters. Recent Advances in Marine Biotechnology 8, 233–268.
- Van den Bogaard, A.E. and Stobberingh, E.E., 2000, Epidemiology of resistance to antibiotics: Links between animals and humans. International Journal of Antimicrobial Agents 14(4), 327–335.
- Treitman, A.N., Yarnold, P.R., Warren, J., and Noskin, G.A., 2005, Emerging incidence of Enterococcus faecium among hospital isolates (1993 to 2002). Journal of Clinical Microbiology 43(1), 462–463.
- Bonten, M.J. and Willems, R.J., 2012, Vancomycin-resistant enterococcus–chronicle of a foretold problem. Nederlands Tijdschrift Voor Geneeskunde 156(38), A5233–A5233.
- Fisher, K. and Phillips, C., 2009, The ecology, epidemiology and virulence of Enterococcus. Microbiology 155(6), 1749–1757.
- Mendes, R.E., Castanheira, M., Farrell, D.J., Flamm, R.K., Sader, H.S., and Jones, R.N., 2016, Longitudinal (2001–14) analysis of enterococci and VRE causing invasive infections in European and US hospitals, including a contemporary (2010–13) analysis of oritavancin in vitro potency. Journal of Antimicrobial Chemotherapy 71(12), 3453–3458.
- Clewell, D.B., Weaver, K.E., Dunny, G.M., Coque, T.M., Francia, M.V., and Hayes, F., 2014, Extrachromosomal and Mobile Elements in Enterococci: Transmission, Maintenance, and Epidemiology. In: M.S. Gilmore, D.B. Clewell, Y. Ike, T.M. Coque, M.V. Francia, and F. Hayes (Eds) Enterococci: From Commensals to Leading Causes of Drug Resistant Infection [Internet] (Boston: Massachusetts Eye and Ear Infirmary), pp. 1–112. Available online at: https://www.ncbi.nlm.nih.gov/books/NBK190430/ (accessed 27 February 2018).
- Kayaoglu, G. and Ørstavik, D., 2004, Virulence factors of Enterococcus faecalis: Relationship to endodontic disease. Critical Reviews in Oral Biology & Medicine 15(5), 308–320.
- Cetinkaya, Y., Falk, P., and Mayhall, C.G., 2000, Vancomycin-resistant enterococci. Clinical Microbiology Reviews 13(4), 686–707.
- Duprè, I., Zanetti, S., Schito, A.M., Fadda, G., and Sechi, L.A., 2003, Incidence of virulence determinants in clinical Enterococcus faecium and Enterococcus faecalis isolates collected in Sardinia (Italy). Journal of Medical Microbiology 52(6), 491–498.
- Novais, C., Coque, T.M., Ferreira, H., Sousa, J.C., and Peixe, L., 2005, Environmental contamination with vancomycin-resistant enterococci from hospital sewage in Portugal. Applied and Environmental Microbiology 71(6), 3364–3368.
- APAH, W., 2005, AWWA. Standard Methods for the Examination of Water and Wastewater. 21st ed. (Washington, DC: American Public Health Association). Available online at: https://www.scirp.org/(S(czeh2tfqyw2orz553k1w0r45))/reference/ReferencesPapers.aspx?ReferenceID=1870039 (accessed 10 June 2018).
- Maugeri, N., Giordano, G., Petrilli, M.P., Fraticelli, V., De Gaetano, G., Cerletti, C., Storti, S., and Donati, M.B., 2006, Inhibition of tissue factor expression by hydroxyurea in polymorphonuclear leukocytes from patients with myeloproliferative disorders: A new effect for an old drug? Journal of Thrombosis and Haemostasis 4(12), 2593–2598.
- Ke, D., Picard, F.J., Martineau, F., Ménard, C., Roy, P.H., Ouellette, M., and Bergeron, M.G., 1999, Development of a PCR assay for rapid detection of enterococci. Journal of Clinical Microbiology 37(11), 3497–3503.
- Adeniji, O.O., Sibanda, T., and Okoh, A.I., 2019, Recreational water quality status of the Kidd’s Beach as determined by its physicochemical and bacteriological quality parameters. Heliyon 5(6), e01893.
- Mannu, L., Paba, A., Daga, E., Comunian, R., Zanetti, S., Duprè, I., and Sechi, L.A., 2003, Comparison of the incidence of virulence determinants and antibiotic resistance between Enterococcus faecium strains of dairy, animal and clinical origin. International Journal of Food Microbiology 88(2–3), 291–304.
- Vankerckhoven, V., Van Autgaerden, T., Vael, C., Lammens, C., Chapelle, S., Rossi, R., Jabes, D., and Goossens, H., 2004, Development of a multiplex PCR for the detection of asa1, gelE, cylA, esp, and hyl genes in enterococci and survey for virulence determinants among European hospital isolates of. Enterococcus Faecium. Journal of Clinical Microbiology 42(10), 4473–4479.
- CLSI, 2018, Performance Standards for Antimicrobial Susceptibility Testing. 28th ed. CLSI supplement M100 (Wayne, PA: Clinical and Laboratory Standards Institute). Available online at: https://clsi.org/media/1930/m100ed28_sample.pdf (accessed 04 April 2017).
- Panda, S.K., Patra, A.K., and Kar, R.N., 2012, Monitoring of multiple drug-resistant pathogens in a selected stretch of Bay of Bengal, India. Environmental Monitoring and Assessment 184(1), 193–200.
- Wose Kinge, C. and Mbewe, M., 2010, Characterisation of Shigella species isolated from river catchments in the North West province of South Africa. South African Journal of Science 106(11–12), 1–4.
- Blasco, M.D., Esteve, C., and Alcaide, E., 2008, Multiresistant waterborne pathogens isolated from water reservoirs and cooling systems. Journal of Applied Microbiology 105(2), 469–475.
- Krumperman, P.H., 1983, Multiple antibiotic resistance indexing of Escherichia coli to identify high-risk sources of fecal contamination of foods. Applied and Environmental Microbiology 46(1), 165–170.
- Lebreton, F., Riboulet-Bisson, E., Serror, P., Sanguinetti, M., Posteraro, B., Torelli, R., Hartke, A., Auffray, Y., and Giard, J.C., 2009, ace, which encodes an adhesin in Enterococcus faecalis, is regulated by Ers and is involved in virulence. Infection and Immunity 77(7), 2832–2839.
- Lin, Y.T., Hsieh, K.S., Chen, Y.S., Huang, I.F., and Cheng, M.F., 2013, Infective endocarditis in children without underlying heart disease. Journal of Microbiology, Immunology and Infection 46(2), 121–128.
- Sharifi, M., Bay, C., Skrocki, L., Rahimi, F., and Mehdipour, M., 2013, Moderate pulmonary embolism treated with thrombolysis (from the “MOPETT” Trial). The American Journal of Cardiology 111(2), 273–277.
- Tiwari, S. and Beriha, S.S., 2015, Pantoea species causing early onset neonatal sepsis: A case report. Journal of Medical Case Reports 9(1), 188.
- Wade, T.J., Sams, E., Brenner, K.P., Haugland, R., Chern, E., Beach, M., Wymer, L., Rankin, C.C., Love, D., Li, Q., and Noble, R., 2010, Rapidly measured indicators of recreational water quality and swimming-associated illness at marine beaches: A prospective cohort study. Environmental Health 9(1), 66.
- Łuczkiewicz, A., Jankowska, K., Fudala-Książek, S., and Olańczuk-Neyman, K., 2010, Antimicrobial resistance of fecal indicators in municipal wastewater treatment plant. Water Research 44(17), 5089–5097.
- Garrido, A.M., Gálvez, A., and Pulido, R.P., 2014, Antimicrobial resistance in enterococci. Journal of Infectious Diseases and Therapy 2, 150.
- Kimiran-Erdem, A., Arslan, E.O., Yurudu, N.O.S., Zeybek, Z., Dogruoz, N., and Cotuk, A., 2007, Isolation and identification of enterococci from seawater samples: Assessment of their resistance to antibiotics and heavy metals. Environmental Monitoring and Assessment 125(1–3), 219–228.
- Leonard, A.F., Zhang, L., Balfour, A.J., Garside, R., and Gaze, W.H., 2015, Human recreational exposure to antibiotic resistant bacteria in coastal bathing waters. Environment International 82, 92–100.
- Department of Health, Public Health England, Department for Environment Food & Rural Affairs, Veterinary Medicines Directorate, 2014, Antimicrobial resistance systems map: overview of the factors influencing the development of AMR and the interactions between them. Available online at: https://www.nao.org.uk/wp-content/uploads/2014/11/The-Performance-of-the-Department-for-Environment-Food-and-Rural-Affairs-2013-14.pdf (accessed 15 March 2018).
- Turgeon, P., Michel, P., Levallois, P., Chevalier, P., Daignault, D., Crago, B., Irwin, R., McEwen, S.A., Neumann, N.F., and Louie, M., 2012, Antimicrobial-resistant Escherichia coli in public beach waters in Quebec. Canadian Journal of Infectious Diseases and Medical Microbiology 23(2), e20–e25.
- World Health Organization, 2000, WHO global principles for the containment of antimicrobial resistance in animals intended for food: Report of a WHO consultation with the participation of the Food and Agriculture Organization of the United Nations and the Office International des Epizooties, Geneva, Switzerland 5–9 June 2000 (No. WHO/CDS/CSR/APH/2000.4). Available online at: https://apps.who.int/iris/bitstream/handle/10665/68931/WHO_CDS_CSR_APH_2000.4.pdf;jsessionid=842D3D52483BCF8022819CEFDC06A90A?sequence=1 (accessed 21 November 2018).
- Gallard, J.C., Hyatt, D.R., Crupper, S.S., and Acheson, D.W., 2001, Prevalence, antibiotic susceptibility and diversity of E. coli O157: H7 isolates from a longitudinal study of beef cattle feedlots. Applied and Environmental Microbiology 67, 1619–1627.
- Baquero, F., Martinez, J.L., and Canton, R., 2008, Antibiotics and antibiotic resistance in water environments. Current Opinion in Biotechnology 19, 260–265.
- Courvalin, P., 2008, Predictable and unpredictable evolution of antibiotic resistance. Journal of Internal Medicine 264(1), 4–16.
- Aminov, R.I. and Mackie, R.I., 2007, Evolution and ecology of antibiotic resistance genes. FEMS Microbiology Letters 271(2), 147–161.
- Alipour, M., Hajiesmaili, R., Talebjannat, M., and Yahyapour, Y., 2014, Identification and antimicrobial resistance of Enterococcus spp. isolated from the river and coastal waters in northern Iran. The Scientific World Journal 2014, 1–5, Article ID 287458.
- Munita, J.M. and Arias, C.A., 2016, Mechanisms of antibiotic resistance. Microbiology Spectrum 4(2), 1–24, VMBF-0016-2015.
- Miller, W.R., Munita, J.M., and Arias, C.A., 2014, Mechanisms of antibiotic resistance in enterococci. Expert Review of Anti-Infective Therapy 12(10), 1221–1236.
- Hooper, D.C. and Jacoby, G.A., 2015, Mechanisms of drug resistance: Quinolone resistance. Annals of the New York Academy of Sciences 1354(1), 12.
- Goldstein, R.E.R., Micallef, S.A., Gibbs, S.G., George, A., Claye, E., Sapkota, A., Joseph, S.W., and Sapkota, A.R., 2014, Detection of vancomycin-resistant enterococci (VRE) at four US wastewater treatment plants that provide effluent for reuse. Science of the Total Environment 466, 404–411.
- Nishiyama, M., Iguchi, A., and Suzuki, Y., 2015, Identification of Enterococcus faecium and Enterococcus faecalis as vanC-type vancomycin-resistant enterococci (VRE) from sewage and river water in the provincial city of Miyazaki, Japan. Journal of Environmental Science and Health Part A 50, 16–25.
- Roberts, M.C., Soge, O.O., Giardino, M.A., Mazengia, E., Ma, G., and Meschke, J.S., 2009, Vancomycin‐resistant Enterococcus spp. in marine environments from the West Coast of the USA. Journal of Applied Microbiology 107, 300–307.
- Zdragas, A., Partheniou, P., Kotzamanidis, C., Psoni, L., Koutita, O., Moraitou, E., Tzanetakis, N., and Yiangou, M., 2008, Molecular characterization of low-level vancomycin-resistant enterococci found in coastal water of Thermaikos Gulf, Northern Greece. Water Research 42, 1274–1280.
- Kak, V. and Chow, J.W., 2002, Acquired antibiotic resistances in Enterococci. In: M.S. Gilmore, D.B. Clewell, P. Courvalin, G.M. Dunny, B.E. Murray, and L.B. Rice (Eds) The Enterococci: Pathogenesis, Molecular Biology, and Antibiotic Resistance (Washington, D. C.: American Society for Microbiology Press), pp. 355–383.
- Bustamante, W., Alpízar, A., Hernández, S., Pacheco, A., Vargas, N., Herrera, M.L., Vargas, Á., Caballero, M., and García, F., 2003, Predominance of vanA genotype among vancomycin-resistant Enterococcus isolates frim poultry and swine in Costa Rica. Applied and Environmental Microbiology 69, 7414–7419.
- Manson, J.M., Smith, J.M.B., and Cook, G.M., 2004, Persistence of vancomycin resistant enterococci in New Zealand broilers after discontinuation of avoparcin use. Applied and Environmental Microbiology 70, 5764–5768.
- Nilsson, O., 2012, Vancomycin resistant enterococci in farm animals–occurrence and importance. Infection Ecology & Epidemiology 2(1), 16959.
- Riaz, S., Faisal, M., and Hasnain, S., 2011, Antibiotic susceptibility pattern and multiple antibiotic resistances (MAR) calculation of extended spectrum b- (ESBL) producing Escherichia coli and Klebsiella species in Pakistan. African Journal of Biotechnology 10, 6325–6331.
- Christopher, A.F., Hora, S., and Ali, Z., 2013, Investigation of plasmid profile, antibiotic susceptibility pattern multiple antibiotic resistance index calculation of Escherichia coli isolates obtained from different human clinical specimens at tertiary care hospital in Bareilly-India. Annals of Tropical Medicine and Public Health 6(3), 285.
- Ruban, P. and Gunaseelan, C., 2011, Antibiotic resistance of bacteria from Krishna Godavari Basin, Bay of Bengal, India. Environmental and Experimental Biology 9, 33–136.
- Al-Bahry, S., Mahmoud, I., Elshfie, A., Al-Harthy, A., Al-Ghafri, S., and Al-Amri, I., 2009, Bacterial flora and antibiotic resistance from eggs of green turtles Chelonia myans: An indication of polluted effluents. Maine Pollution Bulletin 58, 720–725.
- Mundy, L.M., Sahm, D.F., and Gilmore, M., 2000, Relationship between enterococcal virulence and antimicrobial resistance. Clinical Microbiology Reviews 13(4), 513–522.
- Gundogan, N., Citak, S., Yucel, N., and Devren, A., 2005, A note on the incidence and antibiotic resistance of Staphylococcus aureus isolated from meat and chicken samples. Meat Science 69(4), 807–810.
- Schwartz, T., Kohnen, W., Jansen, B., and Obst, U., 2003, Detection of antibiotic-resistant bacteria and their resistance genes in wastewater, surface water, and drinking water biofilms. FEMS Microbiology Ecology 43, 325–335.
- Hellweger, F., Ruan, X., and Sanchez, S., 2011, A simple model of tetracycline antibiotic resistance in the aquatic environment (with application to the Poudre River). International Journal of Environmental Research and Public Health 8(2), 480–497.
- Suzuki, S. and Hoa, P.T., 2012, Distribution of quinolones, sulfonamides, tetracyclines in aquatic environment and antibiotic resistance in Indochina. Frontiers in Microbiology 3, 67.
- Partridge, S.R., Kwong, S.M., Firth, N., and Jensen, S.O., 2018, Mobile genetic elements associated with antimicrobial resistance. Clinical Microbiology Reviews 31(4), e00088–17.
- Aarestrup, F.M., Agerso, Y., GernerSmidt, P., Madsen, M., and Jensen, L.B., 2000, Comparison of antimicrobial resistance phenotypes and resistance genes in Enterococcus faecalis and Enterococcus faecium from humans in the community, broilers, and pigs in Denmark. Diagnostic Microbiology and Infectious Disease 37, 127–137.
- Del Campo, R., Ruiz-Garbajosa, P., Sa´nchez-Moreno, M.P., Baquero, F., Torres, C., Canto´n, R., and Coque, T.M., 2003, Antimicrobial resistance in recent fecal enterococci from healthy volunteers and food handlers in Spain: Genes and phenotypes. Microbial Drug Resistance 9, 47–60.
- Rice, L.B., 1998, Tn916 family conjugative transposons and dissemination of antimicrobial resistance determinants. Antimicrobial Agents and Chemotherapy 42, 1871–1877.
- Roberts, M.C., 2005, Update on acquired tetracycline resistance genes. FEMS Microbiology Letters 245, 195–203.
- Leclercq, R., 2002, Mechanisms of resistance to macrolides and lincosamides: Nature of the resistance elements and their clinical implications. Clinical Infectious Diseases 34, 482–492.
- Navaneeth, B.V., 2006, A preliminary in vitro study on inducible and constitutive clindamycin resistance in Staphylococcus aureus from a South Indian tertiary care hospital. International Journal of Infectious Diseases 10(2), 184–185.
- Roberts, M.C., 2011, Environmental Macrolide–Lincosamide–Streptogramin and Tetracycline Resistant Bacteria. Frontiers in Microbiology 2, 40.
- Magiorakos, A.P., Srinivasan, A., Carey, R.B., Carmeli, Y., Falagas, M.E., and Giske, C.G., 2014, Multidrug-resistant, extensively drug-resistant and pandrug-resistant bacteria: An international expert proposal for interim standard definitions for acquired resistance. Clinical Microbiology and Infection 18(3), 268–281.
- Torell, E., Kuhn, I., Olsson-Liljequist, B., Haeggman, S., and Hoffman, B.M., 2003, Clonality among ampicillin-resistant Enterococcus faecium isolates and relationship with ciprofloxacin resistance. Journal of Clinical Microbiology and Infection 9, 1011–1019.