ABSTRACT
We assessed the prevalence of E. coli O157:H7 in irrigation water and agricultural soil samples in two District Municipalities in South Africa using standard culture-based and molecular techniques. Presumptive E. coli O157:H7 counts in irrigation water and agricultural soil samples ranged from 1.00 Log10 CFU/100 ml to 4.11 Log10 CFU/100 ml and 2.20 Log10 CFU/g to 4.21 Log10 CFU/g respectively (P ≤ 0.05). Thirteen (28%) of the confirmed isolates (n = 46) were Shiga toxigenic and 33 (72%) were Shiga toxin negative. The presence of Shiga toxigenic E. coli O157:H7 strain in irrigation water and agricultural soil samples reported by this study suggests potential risk to food safety and population health.
Introduction
Escherichia coli O157:H7 serotype is a common Shiga toxigenic E. coli (STEC) that causes food-related disease in human beings. It is the most frequently recovered serotype of enterohaemorrhagic E. coli (EHEC) from sick individuals. Non-motile strains of the STEC are seldom recovered [Citation1].
E. coli O157:H7 harbours the somatic (O) antigen 157 and flagella (H) antigen 7 [Citation2], which are typically screened for during their molecular confirmation. Shiga-toxin (Stx) is the major virulence determinant of E. coli O157:H7 and promotes the extensive damage of the intestinal lining leading to diarrhoea. This toxin is further divided into Stx 1 and Stx 2, coded by the stx1 and stx2 genes which causes the haemorrhagic uraemic syndrome (HUS) [Citation3]. STEC O157:H7 poses a great danger to the health of humans owing to its high virulence potential, low infectious dose (10–100 CFUs), persistence in the environment and resistance to treatment options [Citation3]. Clinical syndromes produced by E. Coli O157:H7 range from slight intestinal distress to HUS, end-stage renal disease (ESRD), and death [Citation4]. Owing to the high rate of mortality associated with E. coli O157, this pathogen is easily differentiated from other pathotypes of E. coli such as enteroaggregative E. coli (AggEC), enteropathogenic E. coli (EPEC) and enterotoxigenic E. coli (ETEC) [Citation1].
Cattle are the main and usually asymptomatic carriers of E. coli O157:H7. Generally, 1–50% of healthy cattle pass this pathogen through their faeces at any given time, hence regarded as the main contamination source [Citation5]. Other animals that harbour this pathogen include goats, pigs, sheep and turkeys and these also pass it via faeces [Citation2].
E. coli O157:H7 is transferred to agricultural soils when the faeces of cattle and other ruminants are used as manure. The pathogen is also transferred to nearby freshwater bodies including rivers and streams through water runoff [Citation6]. E. coli O157:H7 is spread to the food chain and eventually to human consumers when farm produce grown on contaminated soil comes in contact with this pathogen or when polluted water is used to irrigate the farm’s crops. There is every possibility for vegetables grown on manure amended soil to be contaminated with E. coli O157 [Citation7] and the contamination routes are not only restricted to direct contact with the pathogen but also via passive diffusion from the soil, through plant roots into edible parts of the plant [Citation8].
Ready-to-eat (RTE) vegetables and fruits enhance the nutritional and health status of human beings, and the demand for fresh or minimally-processed farm produce has increased in the last few decades [Citation9,Citation10]. The contamination of fresh produce including fruits, leafy and non-leafy vegetables with E. coli O157 is well documented [Citation11,Citation12]. But there is limited information on the occurrence and relative abundance of Shiga toxin and non-Shiga toxin-producing E. coli O157:H7 in irrigation water and agricultural soil in South Africa, although these are known as important transmission routes of pathogenic bacteria to fresh produce. This study, therefore, aims at assessing the prevalence of Shiga toxin and non-Shiga toxin producing E. coli O157:H7 strains in irrigation water and agricultural soil samples collected from two District Municipalities in South Africa.
Materials and methods
Study sites
This study was carried out in Chris Hani and Amathole District Municipalities (DMs), Eastern Cape Province of South Africa. Agricultural production of fresh produce is one of the major activities within the Province. These DMs enjoy the benefit of proximity to seaports of Port Elizabeth and East London, attracting investment in agro-processing. Agricultural soil and irrigation water samples were retrieved from 19 sampling sites located within these two DMs (). We could not collect soil samples from S1, S4, S6, S10, S15 and S19 because the farms were not accessible.
Table 1. Description of sampling points
Sample collection
A grab sampling of 13 agricultural soil and 19 irrigation water samples was carried out, after the research team obtained permission from farm owners. During the sampling regimen, irrigation water samples (1 litre approximately) were collected in triplicates in 1 L sterile sample bottles and agricultural soil samples (30 g approximately) were collected in triplicates in sterile plastic bags. All the samples were placed on ice and transported to the laboratory for processing.
Processing of samples and enumeration of E. coli O157:H7 isolates
Irrigation water samples were serially diluted as described by Adefisoye et al. [Citation13]. One hundred millilitre aliquot of the dilutions was filtered via 0.45 µm pore size and 37 mm diameter membrane filters (Merck, SA) in triplicates aided with a vacuum pump. After filtration, the filters were aseptically transferred to plates containing Sorbitol-MacConkey agar (SMA) (Merck, SA) augmented with cefixime (50 μg/L) and tellurite (25 mg/L) for the enumeration of E. coli O157:H7. One gramme of each soil sample was vortexed with sterile 10 ml distilled water. The soil suspension was then serially diluted as described by Ogunmwonyi et al. [Citation14]. Thereafter, 100 µL of each dilution were inoculated on already prepared SMA (Merck, SA) augmented with cefixime (50 μg/L) and tellurite (25 mg/L) and spread evenly on the medium using a sterile glass spreader as described by Cheesbrough [Citation15] in triplicates for enumeration purposes. All the plates were then incubated for 24 hours at 37°C. After incubation, the target isolates were identified by morphology, counted and recorded. Colourless colonies were counted as presumptive E. coli O157:H7. The data were presented as ‘Log (x + 1) CFU/100 ml and Log (x + 1) CFU/g’ for water and soil samples respectively.
Isolation of E. coli O157:H7
Ten millilitres of each water sample and 10 g of each soil sample were transferred to 90 ml of Trypticase soy broth (TSB) (Merck, SA) for enrichment purposes, and incubated at 37°C for 24 hours, after which a loop full of the broth was streaked on SMA augmented with cefixime (50 μg/L) and tellurite (25 mg/L) and incubated at the same conditions. After incubation, pure colonies considered presumptive were stored in 25% glycerol at −80 °C for subsequent investigations. A total number of 202 presumptive isolates were stored in glycerol stocks.
Molecular identification and virulence typing
Prior to molecular identification, the DNA of the presumptive E. coli O157:H7 isolates was extracted using the boiling technique described by Maugeri et al. [Citation16] and Lopez-Saucedo et al. [Citation17]. Thereafter, the confirmation of the isolates was done using PCR as described by Wang et al. [Citation18]. Supplementary Table 1 details the primer sequence and conditions used for the identification of the target genes (rfbEO157 and fliCH7). For reference purposes, ‘E. coli O157:H7 ATCC 35,150’ was included as a control strain. PCR amplicons (5.0 µL aliquot each) were electrophoresed in 2% (w/v) agarose gel (Merck, SA) as described by Wang et al. [Citation18] and photographed using a UV transilluminator. A 50-bp DNA ladder (Inqaba Biotec, SA) was included in each reaction to serve as a DNA size marker.
Virulence genes including stx1 and stx2 that code for Shiga toxins were screened for using PCR as described previously [Citation19]. The extraction of the DNA template from confirmed isolates was done using the boiling technique as described by Maugeri et al. [Citation16] and Lopez-Saucedo et al. [Citation17]. Supplementary Table 2 details the specific primers and cycling conditions used for the screening of Shiga toxins. The electrophoresis of the PCR amplicons was done as described previously [Citation19] and photographed using a UV transilluminator. A 100 bp DNA ladder (Inqaba Biotec, SA) was included on each gel as a DNA size marker.
Data analysis
The ‘IBM Statistical Package for Social Sciences’ (SPSS version 21) was used to analyse the data obtained from the present study. The one-way analysis of variance was used to compare the counts of E. coli O157:H7 in irrigation water and agricultural soil samples obtained from various sampling sites. Mean was significant at P-values ≤ 0.05.
Results and discussion
The distribution of presumptive E. coli O157:H7
shows the distribution of presumptive E. coli O157:H7 in the samples collected from the study sites. In irrigation water samples, presumptive E. coli O157:H7 counts ranged from 1.00 Log10 CFU/100 ml in S12 and S17 to 4.11 Log10 CFU/100 ml in S18. In agricultural soil samples, presumptive E. coli O157:H7 counts ranged from 2.20 Log10 CFU/g in S12 to 4.21 Log10 CFU/g in S9. The distribution of the isolates in the irrigation water and agricultural soil samples with respect to each sampling site was statistically significant (P ≤ 0.05).
Figure 1. The distribution of E. coli O157:H7 in irrigation water samples (purple bars) and agricultural soil samples (blue bars) retrieved from the study sites. Mean of E. coli O157:H7 counts within the study sites are statistically significant at P ≤ 0.05, F = 211.790 for water samples and P ≤ 0.05, F = 437.191 for soil samples. The bars with the same colour which have the same letter(s) are not significantly different (P ≥ 0.05, Duncan) across the sampling sites
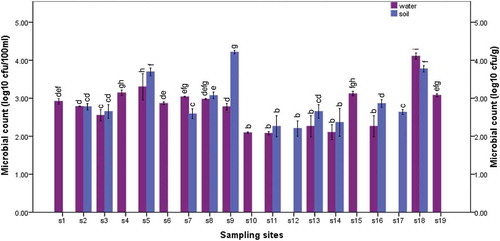
There are no available guidelines set for the counts of E. coli O157:H7 in irrigation water and agricultural soil in South Africa. The guidelines set for faecal coliforms (0.0 CFU/100 ml) in domestic water in South Africa [Citation20] and the standard (≤100 CFU/100 ml coliforms) set by the World Health Organisation on the use of wastewater in agriculture and aquaculture [Citation21] were therefore used to assess the quality of the irrigation water samples collected in this study. Accordingly, all the water samples, except those collected from S12 and S17 were unfit for the primary cultivation of farm produce, and consequently potential transmission routes of E. coli O157:H7 to fresh produce, corroborating previous reports [Citation6,Citation22]. This is probably because most irrigation water sources sampled in the present study were surface waters which were vulnerable to various contamination sources including runoff, animal intrusion, discharge of farm and WWTPs effluents. This suggests that these water sources are unfit for use to irrigate fresh produce unless adequately treated. Interestingly, no count was observed in water samples collected from S12 and S17, probably because, water used in S12 and S17 came from pipe borne and borehole water respectively, with little human interference. These water sources are recommended as the most reliable water sources for the irrigation of farm produce and other agricultural practices. Owing to their high cost, however, most farmers revert to surface water and sometimes, wastewater for irrigation purposes which is detrimental to human health unless adequately treated.
There is little information on counts of E. coli O157:H7 in agricultural soil. Regrettably, it is almost inevitable for agricultural soil to contain pathogens such as E. coli O157:H7 because it is naturally open to direct and indirect sources of microbial contaminants such as animal dung, runoff, contaminated irrigation water, free-ranging animals, municipal sewage and effluents [Citation23,Citation24]. Implementing good agricultural practices such as steady cleaning of farm tools, erecting of fences around the farm to avoid unwanted animal intrusion, efficient treatment of organic fertilisers before application to the soil, use of potable water for irrigation purposes and appropriate irrigation method such as surface irrigation rather than spray irrigation method, will help to prevent the incidence and transmission of pathogenic bacteria such as E. coli O157:H7 to fresh produce [Citation25,Citation26].
The prevalence of confirmed E. coli O157:H7 isolates
Presumptive E. coli O157:H7 was confirmed in 37% (7/19) of the irrigation water samples and 38% (5/13) of the agricultural soil samples.
The prevalence of E. coli O157:H7 in irrigation water samples in this study is much higher than the 2.1% reported by Chigor et al. [Citation27] in river water used for the irrigation of fresh produce. The prevalence is also higher than the 18.7% reported by Myataza et al. [Citation6] in samples collected from portable water channels, irrigation water systems and dairy wastewater in commercial dairy farms. This finding is not entirely surprising as herds of feral cattle, considered as the chief source of E. coli O157:H7 were seen flocking around most of the irrigation water sources. The high prevalence of E. coli O157:H7 in irrigation water samples reported in this study is also attributed to the vulnerability of most of the irrigation water sources to several anthropogenic activities: dumping of refuse, discharge of WWTP effluents, discharge of swine effluents, etc. These are indeed potential sources of E. coli O157:H7, which consequently impairs the safety of the fresh produce irrigated by the irrigation water sources.
The prevalence of E. coli O157:H7 in agricultural soil samples in the present study is also high compared to the study of Khandaghi et al. [Citation28], where only 1.77% of soil samples fertilised with manure in agricultural farms were positive for E. coli O157:H7. In the present study, however, the prevalence of E. coli O157:H7 in agricultural soil was much lower than 52.7% reported for manure obtained from organic and low input dairy farms [Citation29]. Again, our finding is not entirely surprising as improperly composted manure of animal origin was used to amend the soil in most of the sampling sites during the primary production of fresh produce. This shows that the survival and persistence of E. coli O157:H7 in the soil is favoured by the presence of organic materials. This is evident in the study of Gagliardi and Karns [Citation30], where they observed that E. coli O157:H7 survived between 21–45 days in fallow soil following the application of dairy manure. Proper treatment of animal-based manure before being applied to the soil is necessary.
Detection of virulence markers
The confirmed isolates (n = 46) were screened for Shiga toxin genes. Thirteen (28%) were Shiga toxigenic E. coli O157:H7 (STEC O157:H7), out of which 6 (13%) harboured the stx1 gene and 7 (15%) harboured the stx2 gene. Thirty-three (72%) of the isolates did not harbour either of the stx genes. They are hence referred to as non-Shiga toxigenic E. coli O157:H7 (NSTEC O157:H7). All stx2 positive isolates were recovered from irrigation water samples. Two (33%) of the stx1 positive isolates were recovered from irrigation water and the remaining 4 (67%) were recovered from agricultural soil samples. Nine (27%) of the NSTEC O157:H7 were recovered from irrigation water samples and 24 (73%) NSTEC O157:H7 were recovered from agricultural soil samples as shown in .
Table 2. The prevalence of STEC and NSTEC O157:H7 isolates
The prevalence of STEC O157:H7 in the present study is high when compared to the prevalence observed in sediment and water samples (4.4% and 3.3%, respectively) collected from a leafy greens production region [Citation31]. This is attributed to the high level of intrusion by free-ranging cattle to most of the irrigation water sources as well as the use of cow dung as manure in some of the sampling sites. This, in turn, could lead to food safety problems when the pathogens are disseminated to the fresh produce grown on the contaminated soil or irrigated by the contaminated water.
In the present study, a higher prevalence of stx2 was observed than of stx1, contrary to a previous report [Citation32]. All the STEC O157:H7 isolated from irrigation water samples in this study harboured the stx2 genes. Epidemiologically, Stx2 is usually more implicated in human infections especially HC or HUS than the Stx1 [Citation33]. This implies that some of the irrigation water sources in this study might have been contaminated with faeces containing STEC O157:H7, coming from either surface runoff or from improperly treated municipal WWTPs effluents. For instance, the irrigation water source in sampling site S18 is a receiving shed for the effluents of a WWTP. This is detrimental to food safety since irrigation water is almost always in direct contact with farm produce. Conversely, most of stx1 positive variants were isolated from agricultural soil samples. Since stx1 genes are less implicated in human infections, it is hypothesised that the agricultural soil in this study was mostly contaminated by the faeces of ruminants, in the form of manure.
The overall prevalence of NSTEC O157:H7 was higher than STEC O157:H7 in this study. They have also been implicated in severe diseases, even though their impact as pathogens is unknown. It has been shown that Stx does not need to be involved in all manifestations of diseases associated with E. coli O157:H7 [Citation34]. Interestingly, Shigella dysenteriae serotype 1, which lacks the ability to secret Stx, causes non-bloody diarrhoea in monkeys [Citation35]. Li et al. demonstrated that NSTEC O157:H7 induced structural abnormalities in the colon of white rabbits in New Zealand [Citation36]. Streptococcus pneumoniae and Neisseria meningitides are examples of other bacteria that occasionally cause HUS without secreting Stx [Citation37]. Although Schmidt et al. identified stx-deficient E. coli O157 strains from human beings infected with diarrhoea and HUS, they speculated that the isolates had other characteristics that are closely similar to the toxigenic strains [Citation34]. Since the diagnosis of STEC infections is usually based on the detection of stx genes, there may be numerous misdiagnoses of NSTEC associated infections in laboratories [Citation38]. There is a need to improve procedures in testing for these emerging pathogenic strains [Citation38].
Conclusion
The irrigation water and agricultural soil samples collected from Amathole and Chris Hani District Municipalities harbour E. coli O157:H7, which could be transferred to fresh produce intended for human consumers. Some of the isolates harboured either the stx1 or stx2 gene making them Shiga toxigenic and highly virulent, thus capable of causing severe forms of infection in human beings. We, therefore, recommend the control of the incidence of E. coli O157:H7 on the farm via improved cattle management practices, fencing of farms and irrigation water sources to prevent the encroachment of ruminants, besides proper treatment of ruminant-derived manure and irrigation water before application to the soil.
Author Contributions
CDI and AIO contributed to the conception and design of the study. CDI collected the data and prepared the draft manuscript. EDP, LK, AIO and CDI revised the sections of the manuscript. All authors contributed to revision of the manuscript, and read and approved the submitted version.
Acknowledgments
We are grateful to the South African Medical Research Council, United States Agency for International Development and the Department of Science and Technology of South Africa for financial support; and to the Editor, Dr Michael Brett-Crowther for his many efforts.
Disclosure statement
No potential conflict of interest was reported by the authors.
Supplementary materials
Supplemental data for this article can be accessed here.
Additional information
Funding
References
- Law, D., 2000, Virulence factors of Escherichia coli O157 and other Shiga toxin-producing E. coli. Journal of Applied Microbiology 88(5), 729–745. doi: 10.1046/j.1365-2672.2000.01031.x
- Lim, J.Y., Yoon, J.W., and Hovde, C.J., 2010, A brief overview of Escherichia coli O157: H7and Its Plasmid O157. Journal of Microbial Biotechnology 20(1), 5–14. doi: 10.4014/jmb.0908.08007
- Lupindu, A.M., 2018, Epidemiology of Shiga toxin-producing Escherichia coli O157:H7 in Africa in review. Southern African Journal of Infectious Diseases 33(1), 24–30. doi: 10.1080/23120053.2017.1376558
- Gould, L.H., Demma, L., Jones, T.F., Hurd, S., Vugia, D.J., Smith, K., Shiferaw, B., Segler, S., Palmer, A., Zansky, S., and Griffin, P.M., 2009, Hemolytic uremic syndrome and death in persons with Escherichia coli O157:H7 Infection, foodborne diseases active surveillance network sites, 2000–2006. Clinical Infectious Diseases 49(10), 1480–1485. doi: 10.1086/644621
- Baker, C.A., Rubinelli, P.M., Park, H.S., Carbonero, F., and Ricke, S.C., 2016, Shiga toxin-producing Escherichia coli in food: Incidence, ecology, and detection strategies. Food Control 59, 407–419. doi: 10.1016/j.foodcont.2015.06.011
- Myataza, A., Igbinosa, E.O., Igumbor, E.U., Nontongana, N., and Okoh, A.I., 2017, Incidence and antimicrobial susceptibility of Escherichia coli O157: H7isolates recovered from dairy farms in amathole district municipality, Eastern Cape, South Africa. Asian Pacific Journal of Tropical Disease 7(12), 765–770. doi: 10.12980/apjtd.7.2017D7-198
- Saeed, A.Y., Mazin, H., Saadi, A., and Hussein, S.O., 2013, Detection of Escherichia coli O157 in vegetables. IOSR Journal of Agriculture and Veterinary Science 6(2), 16–18. doi: 10.9790/2380-0621618
- Solomon, E.B., Yaron, S., and Matthews, K.R., 2002, Transmission of Escherichia coli O157: H7from contaminated manure and irrigation water to lettuce plant tissue and its subsequent internalization. Applied and Environmental Microbiology 68(1), 397–400. doi: 10.1128/AEM.68.1.397-400.2002
- Oladunjoye, A.O., Oyewole, S.A., Singh, S., and Ijabadeniyi, O.A., 2017, Prediction of Listeria monocytogenes ATCC 7644 growth on fresh-cut produce treated with bacteriophage and sucrose monolaurate by using artificial neural network. LWT - Food Science and Technology 76, 9–17. doi: 10.1016/j.lwt.2016.10.042
- Iwu, C.D., Du Plessis, E.M., Korsten, L., Nontongana, N., and Okoh, A.I., 2020, Antibiogram signatures of some enterobacteria recovered from irrigation water and agricultural soil in two district municipalities of South Africa. Microorganisms 8(8), 1206. doi: 10.3390/microorganisms8081206
- Chang, W.S., Afsah-Hejri, L., Rukayadi, Y., Khatib, A., Lye, Y.L., Loo, Y.Y., Mohd Shahril, N., Puspanadan, S., Kuan, C.H., Goh, S.G., John, Y.H.T., Nakaguchi, Y., Nishibuchi, M., and Son, R., 2013, Quantification of Escherichia coli O157: H7in organic vegetables and chickens. International Food Research Journal 20, 1023–1029.
- Abadias, M., Alegre, I., Oliveira, M., Altisent, R., and Viñas, I., 2012, Growth potential of Escherichia coli O157 : H7 on fresh-cut fruits (melon and pineapple) and vegetables (carrot and escarole) stored under different conditions. Food Control 27(1), 37–44. doi: 10.1016/j.foodcont.2012.02.032
- Adefisoye, M.A. and Okoh, A.I., 2016, Identification and antimicrobial resistance prevalence of pathogenic Escherichia coli strains from treated wastewater effluents in Eastern Cape, South Africa. Microbiology Open 5(1), 143–151.
- Ogunmwonyi, I.N., Igbinosa, O.E., Aiyegoro, O.A., and Odjadjare, E.E., 2008, Microbial analysis of different top soil samples of selected site in Obafemi Awolowo University, Nigeria. Scientific Research and Essay 3, 120–124.
- Cheesbrough, M., 2006, District Laboratory Practice in Tropical Countries, 2nd, (New York: Cambridge University Press).
- Maugeri, T.L., Carbone, M., Fera, M.T., Irrera, G.P., and Gugliandolo, C., 2004, Distribution of potentially pathogenic bacteria as free living and plankton associated in a marine coastal zone. Journal of Applied Microbiology 97(2), 354–361. doi: 10.1111/j.1365-2672.2004.02303.x
- Lopez-Saucedo, C., Cerna, J.F., Villegas-Sepulveda, N., Thompson, R., Velazquez, F.R., Torres, J., Tarr, P.I., and Estrada-Garcia, T., 2003, single multiplex polymerase chain reaction to detect diverse loci associated with diarrheagenic Escherichia coli. Emerging Infectious Diseases 9(1), 127–131. doi: 10.3201/eid0901.010507
- Wang, G., Clark, C.G., and Rodgers, F.G., 2002, Detection in Escherichia coli of the genes encoding the major virulence factors, the genes defining the O157: H7serotype, and components of the type 2 Shiga toxin family by multiplex PCR. Journal of Clinical Microbiology 40(10), 3613–3619. doi: 10.1128/JCM.40.10.3613-3619.2002
- Paton, A.W. and Paton, J.C., 1998, Detection and characterization of shiga ToxigenicEscherichia coli by using multiplex PCR assays forstx 1, stx 2,eaeA, Enterohemorrhagic E. coli hlyA,rfb O111, andrfb O157. Journal of Clinical Microbiology 36(2), 598–602. doi: 10.1128/JCM.36.2.598-602.1998
- DWAF, 2012, Department of water affairs and forestry. South African water quality guidelines. Domestic Water Use 1, 1–197.
- WHO World Health Organization., 2018, Health guidelines for the use of wastewater in agriculture and aquaculture Available online: https://apps.who.int/iris/bitstream/handle/10665/39401/WHO_TRS_778.pdf?sequence=1&isAllowed=y (accessed 3 June 2020).
- Van Donkersgoed, J., Hancock, D., Rogan, D., and Potter, A.A., 2005, Escherichia coli O157: H7vaccine field trial in 9 feedlots in Alberta and Saskatchewan. The Canadian Veterinary Journal 46, 724–728.
- Alegbeleye, O.O., Singleton, I., and Sant’Ana, A.S., 2018, Sources and contamination routes of microbial pathogens to fresh produce during field cultivation: A review. Food Microbiology 73, 177–208. doi: 10.1016/j.fm.2018.01.003
- Iwu, C.D. and Okoh, A.I., 2019, Preharvest transmission routes of fresh produce associated bacterial pathogens with outbreak potentials: a review. International Journal of Environmental Research and Public Health 16(22), 4407. doi: 10.3390/ijerph16224407
- Iwu, C.D. and Okoh, A.I., 2020, Characterization of antibiogram fingerprints in Listeria monocytogenes recovered from irrigation water and agricultural soil samples. PLoS ONE 15(2), e0228956. doi: 10.1371/journal.pone.0228956
- Iwu, C.D., Korsten, L., and Okoh, A.I., 2020, The incidence of antibiotic resistance within and beyond the agricultural ecosystem: A concern for public health. MicrobiologyOpen, e1035.
- Chigor, V.N., Umoh, V.J., and Smith, S.I., 2010, Occurrence of Escherichia coli O157 in a river used for fresh produce irrigation in Nigeria. African Journal of Biotechnology 9, 178–182.
- Khandaghi, J., Razavilar, V., and Barzgari, A., 2010, Isolation of Escherichia coli O157: H7from manure fertilized farms and raw vegetables grown on it, in Tabriz city in Iran. African Journal of Microbiology Research 4, 891–895.
- Franz, E., Klerks, M.M., De Vos, O.J., Termorshuizen, A.J., and Van Bruggen, A.H.C., 2007, Prevalence of Shiga toxin-producing Escherichia coli stx1, stx2, eaeA, and rfbE genes and survival of E. coli O157: H7in manure from organic and low-input conventional dairy farms. Applied and Environmental Microbiology 73(7), 2180–2190. doi: 10.1128/AEM.01950-06
- Gagliardi, J.V. and Karns, J.S., 2002, Persistence of Escherichia coli O157: H7in soil and on plant roots. Environmental Microbiology 4(2), 89–96. doi: 10.1046/j.1462-2920.2002.00273.x
- Cooley, M.B., Jay-Russell, M., Atwill, E.R., Carychao, D., Nguyen, K., Quiñones, B., Patel, R., Walker, S., Swimley, M., Pierre-Jerome, E., Gordus, A.G., and Mandrell, R.E., 2013, Development of a robust method for isolation of shiga toxin-positive Escherichia coli (STEC) from fecal, plant, soil and water samples from a leafy greens production region in California. PLoS ONE 8(6), e65716. doi: 10.1371/journal.pone.0065716
- Iweriebor, B.C., Iwu, C.J., Obi, L.C., Nwodo, U.U., and Okoh, A.I., 2015, Multiple antibiotic resistances among Shiga toxin producing Escherichia coli O157 in feces of dairy cattle farms in Eastern Cape of South Africa. BMC Microbiology 15(213), 1–9. doi: 10.1186/s12866-015-0553-y
- Fuller, C.A., Pellino, C.A., Flagler, M.J., Strasser, J.E., and Weiss, A.A., 2011, Shiga toxin subtypes display dramatic differences in potency. Infection and Immunity 79(3), 1329–1337. doi: 10.1128/IAI.01182-10
- Schmidt, H., Scheef, J., Huppertz, H.I., Frosch, M., and Karch, H., 1999, Escherichia coli O157: H7and O157: H(-)strains that do not produce Shiga toxin: Phenotypic and genetic characterization of isolates associated with diarrhea and hemolytic-uremic syndrome. Journal of Clinical Microbiology 37(11), 3491–3496. doi: 10.1128/JCM.37.11.3491-3496.1999
- Fontaine, A., Arondel, J., and Sansonetti, P.J., 1988, Role of Shiga Toxin in the pathogenesis of bacillary dysentery, studied by using a tox- mutant of shigella dysenteriae 1. Infection and Immunity 56(12), 3099–3109. doi: 10.1128/IAI.56.12.3099-3109.1988
- Li, Z., Bell, C., Buret, A., Robins-Browne, R., Stiel, D., and O’Loughlin, E., 1993, The effect of enterohemorrhagic Escherichia coli 0157: H7on intestinal structure and solute transport in rabbits. Gastroenterology 104(2), 467–474. doi: 10.1016/0016-5085(93)90415-9
- Alon, U., Adler, S.P., and Chan, J.C.M., 1984, Hemolytic-uremic syndrome associated with streptococcus pneumoniae: report of a case and review of the literature. American Journal of Diseases of Children 138(5), 496–499. doi: 10.1001/archpedi.1984.02140430072019
- Ferdous, M., Zhou, K., Mellmann, A., Morabito, S., Croughs, P.D., De Boer, R.F., Kooistra-Smid, A.M.D., Rossen, J.W.A., and Friedrich, A.W., 2015, Is Shiga toxin-negative Escherichia coli O157: H7enteropathogenic or enterohemorrhagic Escherichia coli? comprehensive molecular analysis using whole-genome sequencing. Journal of Clinical Microbiology 53, 3530–3538.