Abstract
Objective
The aim of this study was to explore the effects of atypical antipsychotics (AaPs) on brain white matter (WM) tracts in healthy individuals with auditory verbal hallucinations (Hi-AVHs).
Methods
We analyzed neuroimaging, AVH symptoms, and cognitive assessment data obtained from 39 Hi-AVHs who reported being distressed by persistent AVHs and volunteered to receive AaP treatment. We used tract-based spatial statistics (TBSS) and t tests to explore AaP pharmacotherapy effects on AVH symptoms and brain WM alterations in Hi-AVH subjects.
Results
TBSS and t tests revealed WM alterations after AaP treatment, relative to pretreatment observations. Although AaPs alleviated AVH symptoms, WM alterations in these subjects expanded over 8 months of AaP treatment, encompassing most major WM tracts by the end of the observation period, including the corpus callosum, arcuate fasciculus, cortico-spinal tracts, anterior commissure, and posterior commissure.
Conclusions
The worsening of AaP-associated WM alterations observed in this study suggest that AaPs may not be a good choice for the treatment of Hi-AVHs despite their ability to alleviate AVHs.
Introduction
Auditory verbal hallucinations (AVHs) are prevalent among diverse psychiatric patients, including patients with schizophrenia, bipolar disorder, borderline personality disorder, and anxiety; AVHs are also experienced by individuals in the general population [Citation1–3]. Recent elucidation and increased exploration of the pathological features of AVHs and AVH treatment effects in the general population have provided information important for the diagnosis, pathological characterization, and treatment planning for AVHs in general populations [Citation4–7].
Applying the strictest AVH criteria, some 0.7% of the general population has experienced AVHs; these people can be defined as healthy individuals with AVH (Hi-AVHs) [Citation1,Citation8]. Investigating Hi-AVHs may help to clarify the precise pathological features, and thus treatment targets, of AVHs without conflation from other symptoms, such as delusions or thought disturbances [Citation9–11]. The occurrence of AVHs has been related to white matter (WM) alterations in the brain [Citation12–15], and atypical antipsychotic (AaP) medications are known to alleviate AVHs in a variety of psychiatric patients, including patients diagnosed with schizophrenia, bipolar disorder, and borderline personality disorder [Citation6,Citation16–19]. To the best of our knowledge, no study has investigated the relationship between AaP pharmacotherapy and brain WM alterations in Hi-AVHs. In particular, we could not find a cohort study of WM alterations before versus after an extended AaP treatment period.
Dynamic monitoring of WM aberrations in Hi-AVHs can help to further our understanding of the pathological features of AVHs in Hi-AVHs and AVHs in patients diagnosed with a mental disorder. Toward this aim, in the present pilot study, we used magnetic resonance imaging (MRI) to observe the evolution of WM alterations in Hi-AVHs taking AaPs from a pretreatment baseline assessment to 6- and 8-month follow-up time points. The patients’ cognitive functioning levels were assessed to examine whether the treatment had adverse effects on cognition. We used tract-based spatial statistics (TBSS) [Citation20] and t tests to explore the effects of AaP pharmacotherapy on AVH symptoms and brain WM alterations in a cohort of Hi-AVHs.
Methods
Study population
The protocol for this study was approved by the Tianjin Anding Hospital ethics review board. All assessments were carried out in compliance with the Declaration of Helsinki guidelines and approved by the institutional ethics committee of our hospital. All of the participants provided written informed consent for participation in this study after being informed about the potential deleterious effects of taking AaP medication and potential risks of participating in the study. Although the participants volunteered to participate the study, we included only those who self-reported suffering distress from persistent AVHs and wanted to receive AaP treatment. For safety, brain alterations were monitored with MRI examinations during the study period.
An advertising campaign was used to recruit Hi-AVHs from 1000 communities (total source population > 200,000) between January 1st, 2016 and June 31st, 2018; the advertisements targeted individuals identified in our institutional database, which was established in 2011 [Citation21–23]. A total of 300 healthy volunteers with a diagnosis of AVHs according to the diagnostic criteria of Professor Johns [Citation8] were recruited. Of these, 115 reported having suffered mental distress from persistent AVHs and volunteered to receive AaP treatment. None of the AaP-treated Hi-AVHs had a diagnosis of any mental disorder according to the Diagnostic and Statistical Manual of Mental Disorders, 4th Edition, and none had a history of substance abuse, with the exception of moderate to severe nicotine dependence according to the Fagerstrom nicotine dependence questionnaire [Citation24]. AVH severity was assessed with the auditory verbal hallucinations rating scale (AHRS, Haddock et al. 1999) [Citation1].
MRI data acquisition
Each subject completed MRI examinations before starting treatment (baseline) as well as 6 months and 8 months after commencing treatment. The scans were performed with a 3.0-T MR system (Discovery MR750, General Electric, Milwaukee, WI). Tight but comfortable foam padding was used to stabilize the subjects’ head positions, and the subjects wore earplugs to attenuate scanner noise during image acquisition. A three-dimensional T1-weighted brain volume (BRAVO) sequence with 188 sagittal slices was obtained with the following parameters: repetition time = 8.2 ms; echo time = 3.2 ms; inversion time = 450 ms; flip angle = 12°; field of view = 256 mm × 256 mm; matrix = 256 × 256; slice thickness = 1 mm; and no gap.
MRI data analysis
Based on a previous study [Citation20], we employed PANDA software [Citation25], a MATLAB toolbox that integrates FSL (https://fsl.fmrib.ox.ac.uk/fsl/fslwiki), Diffusion Toolkit (http://www.trackvis.org/dtk/), and MRIcron (https://www.nitrc.org/projects/mricron) image processing software. Before processing, the images were subjected to quality checks; images with excessive movements or otherwise poor image quality were discarded. Images that passed the quality control checks were subjected to brain tissue extraction, wherein non-brain tissues were removed, and brain masks were estimated. Subsequently, the images were corrected for the elimination of movements and eddy current-induced distortions. For diffusion tensor imaging (DTI), diffusion tensor fitting was completed and diffusion metrics were calculated in FSL DTI Fit. Finally, the images were subjected to spatial normalization with FSL FNIRT, wherein diffusion metrics were normalized to Montreal Neurological Institute space and segmented images were spatially smoothed with a Gaussian kernel (full width at half maximum = 6 mm3).
TBSS
As described in detail previously [Citation20,Citation25–27], we registered all DTI metrics maps non-linearly to align them with a target image by selecting predefined target image with a 1-mm3 resolution in the standard space. Subsequently, DTI metric images in Montreal Neurological Institute-152 space of all of the subjects were converged into a single four-dimensional image. We set a threshold for the mean fractional anisotropy skeleton image with a default value of 0.2 to exclude voxels in gray matter and cerebrospinal fluid and then created a distance map. Distances from individual voxels to the skeleton were used to project DTI metric values onto the original mean fractional anisotropy skeleton according to the TBSS protocol [Citation20]. Finally, DTI metric images of all subjects were established in the WM skeleton for subsequent analysis.
Cognitive test
Cognitive function was assessed with the Wisconsin Card Sorting Test (WCST)[Citation28]. WCST scores were compared over the longitudinal time points with a repeated measures analysis of variance.
Statistical analysis
The data were analyzed in SPSS Statistics 20.0 for Windows. We used paired t tests to compare WM tract alterations and AVH symptoms before versus after AaP treatment. For AHRS score comparisons, p < 0.05 was considered significant. For WM tract alterations, the data were family wise error (FWE) corrected [Citation29] with a threshold of p < 0.05 to assess small volume corrections.
Results
Treatment status and medication compliance
Of the 115 Hi-AVHs who participated in this study and volunteered to accept pharmacological treatment with risperidone (Johns and Johns, Xi’an Yang-Sen
Pharmaceutical Co., Ltd.), 79 completed the full study-indicated course of risperidone (dropout rate, 31.30%). The main reason for dropping out was an inability or unwillingness to tolerate adverse secondary effects (ASEs) of risperidone. Specifically, 12/36 study dropouts (33.3%) cited ASEs beyond the central nervous system and 8/36 (22.2%) cited prolactin increases. The 36 participants who dropped out had experienced, on average, a 30.5% reduction in AVH symptom severity (determined by the AHRS) after 2 months of AaP treatment (mean dosage, 260.20 ± 55.0 mg, chlorpromazine equivalent).
During the first 6 months of treatment, 46/79 participants (58.22%) experienced extrapyramidal syndrome, and 5/79 participants (6.32%) were found to have elevated prolactin levels; no other obvious ASEs were reported. The mean AaP dosage taken by the 79 retained participants was 270.80 ± 55.50 mg (chlorpromazine equivalent). Most of the retained participants (52/79, 65.8%) experienced a dramatic alleviation of their AVHs (AHRS scores reduced 66.7% compared to baseline). The remaining 27 retained participants experienced a slight alleviation of their AVH symptoms (AHRS scores reduced by on average 27.5%). The retained participants who experienced robust symptom reduction and those who experienced moderate symptom reduction were similar with respect to gender, AVH history duration, AVH symptom severity at baseline, and AaP dosage after acquisition of first MRI scanning data (see ).
Table 1. Demographic and clinical characteristics of the study subjects.
MRI data findings
Of the 115 participants who agreed to undergo MRI scans, only 65 completed full baseline MRI scans, and only 49 completed both baseline and follow-up MRI scans. However, only 39 of the 49 participants who completed both scans had MRI data (DTI sequence acquisition) that were fully valid for TBSS analysis. The main reason for MRI data being unqualified was too much head movement in one or both scans (see limitations text in the Discussion).
Evolution of WM alterations and cognitive performance
Compared to the baseline assessment performed when the patients first accepted AaP, we observed a worsening of WM alterations after the patients had been taking AaP medication for 6 months, mainly in the corpus callosum, anterior commissure, and posterior commissure (). The WCST test results, however, did not show evidence of any decline in the patients’ cognitive function at the 6-month time point. Based on the WCST results, we and the subjects agreed to continue the treatment. All 39 participants with qualified MRI data for both the baseline and the 6-month follow-up scans were among those who experienced dramatic AVH symptom improvement from baseline to the 6-month treatment time point and participated in the subsequent 2-month continuation study. For the 2-month continuation study, all 39 participants were re-briefed regarding potential risks of participating in the study and, subsequently, signed a second written informed consent form. The ethics committee of our hospital also approved the continuation study.
Figure 1. WM changes from baseline to the 6-month AaP treatment time point. Green pseudocolor represents normal WM and blue pseudocolor represents sites of WM impairment.
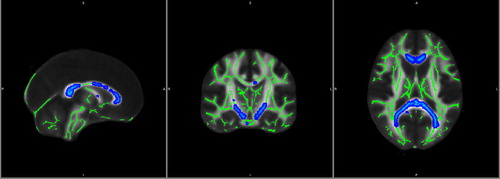
By the 8-month AaP treatment time point, we observed extensive impairment of WM tracts encompassing all major WM tracts, including the corpus callosum, arcuate fasciculus, anterior commissure, posterior commissure, cortico-spinal tracts, and other tracts linking the frontal, temporal, parietal, and occipital lobes (). Despite these disheartening imaging findings, the WCST scores of each subject remained within normal range. Although the Hi-AVHs remained interested in continuing the AaP treatment, we did not agree and suggested that they instead try psychotherapy [Citation30–32] or other interventions, such as avatar therapy or transcranial magnetic stimulation [Citation33–36]. Comparing the 8-month neuroimaging results to those obtained at 6 months, we found that the WM impairments appeared to be even more widespread at the 8-month time point than they had been at the 6-month time point, though still mainly impacting the same regions (). Meanwhile, the subjects did not experience further improvement in their AVH symptoms from the 6-month treatment time point to the 8-month treatment time point ().
Discussion
In this pilot study, we observed mediocre treatment compliance, with a dropout rate of 31.30% principally due to ASEs, and a TBSS-qualified two-scan MRI data rate of only 33.91%. The relatively high dropout rate and low qualified MRI data rate limits the representativeness of our findings. Other strategies, such as repetitive transcranial magnetic stimulation, may be considered for those participants who are not well-suited for AaP therapy due to ASEs. Meanwhile, given the challenges we faced in obtaining TBSS-qualified MRI scans, we intend to develop an improved MRI scanning protocol and methodology to improve MRI data quality with this population. Despite the disappointing dropout rate and TBSS-qualified MRI data rate, we obtained useful information regarding AaP treatment effects and accompanying WM alterations in Hi-AVHs. The main findings of this pilot study were an association of AaP treatment with marked improvements in AVH symptoms within the first 6 months of treatment together with a persistent worsening and expansion of WM alterations in Hi-AVHs over time in the absence of detectable negative effects on cognition as determined by the WCST.
WM tracts mediate information sharing among brain regions, including modulation of diverse brain processes, including sensory, motor, cognitive, executive function, and emotive processes [Citation37–42]. Many previous studies have reported the presence of WM aberrations in association with AVHs in patients with neuropsychiatric diagnoses, supporting the hypothesis that AVHs may be the result of functional co-disturbance of multiple brain networks [Citation43–46].
Although AaPs have been demonstrated to be effective for alleviating AVHs in patients with schizophrenia, bipolar disorder, and borderline personality disorder [Citation16], information about AaP treatment effects on WM alterations is limited. The development of widespread WM alterations in Hi-AVHs taking AaPs while the AaP treatment is alleviating AVH symptoms is a puzzling finding. Notwithstanding, antipsychotic mediations have been reported to induce gray matter and WM structural impairments in the brains of patients with schizophrenia, while also alleviating their hallucinations [Citation47–49]. Further research is needed to clarify the clinical significance of these effects.
Limitations
This pilot study has notable limitations. First, as noted above, we did not obtain good treatment compliance or TBSS-qualified MRI data rates, which limits our ability to make strong inferences from our findings. Second, our study design was weaker than a randomized double-blind controlled study [Citation50,Citation51]. We compared data across time points with paired t tests, but we did not compare data from AaP-treated Hi-AVHs to data from an untreated Hi-AVHs control group or non-AVH control group. Third, we did not take into consideration the potential confounding factors of mood status or distress severity due to a lack suitable assessment tools. We are seeking suitable tools that will enable us to include such information in future research and thus obtain a more detailed characterization of brain alterations. Fourth, our final TBSS study sample included only 39 patients. Although Hi-AVHs are difficult to enroll, we are working to secure a larger sample to enable us to acquire higher accuracy findings in a future study. Fifth, we did not have the technical resources that would enable us to complete more detailed WM analyses, such as fractional anisotropy, radial diffusivity, and axial diffusivity. Additionally, given the extensiveness of the WM tracts affected, we could not establish a direct relationship between AaP dosage and WM impairment. We intend to collaborate with a senior mathematician to help us address these questions in the future. Likewise, due to technical limitations, we did not develop analyses of dynamic WM network alterations.
Conclusion
Despite the aforementioned limitations, in this pilot study, we found that AaP treatment was associated with marked WM deterioration while alleviating AVH symptoms in Hi-AVHs. Though the scope of WM alterations increased over time, the AaP treatment did not cause detectable cognitive impairments in Hi-AVHs. Considering the rapid WM changes observed, we do not recommend that AaPs be a first-line treatment for AVHs in Hi-AVHs. Rather, we suggest that other types of intervention, such as psychotherapy or avatar therapy, be used to treat AVH symptoms in Hi-AVHs. Finally, given our technical limitations, we welcome collaboration with scholars with complementary expertise for future research.
Contributors
Chuanjun Zhuo, Chunhua Zhou, Yong Xu conceived and designed the experiments; Xiaodong Lin, Gongying Li, Feng Ji, Langlang Cheng performed the experiments; Guangdong Chen, Wei Zhang, Zhonghua Su, Zhenqing Zhang, Wenqiang Wang analyzed the data, Chuanjun Zhuo, Xiaodong Lin wrote the paper. All authors approved the final version of the paper.
Disclosure statement
No potential conflict of interest was reported by the author(s).
Additional information
Funding
References
- Upthegrove R, Broome MR, Caldwell K, et al. Understanding auditory verbal hallucinations: a systematic review of current evidence. Acta Psychiatr Scand. 2016;133(5):352–367.
- Laroi F, Sommer IE, Blom JD, et al. The characteristic features of auditory verbal hallucinations in clinical and nonclinical groups: state-of-the-art overview and future directions. Schizophr Bull. 2012;38(4):724–733.
- Waters F, Woods A, Fernyhough C. Report on the 2nd International Consortium on Hallucination Research: evolving directions and top-10 “hot spots” in hallucination research. Schizophr Bull. 2014;40(1):24–27.
- Diederen KM, van Lutterveld R, Sommer IE. Neuroimaging of voice hearing in non-psychotic individuals: a mini review. Front Hum Neurosci. 2012;6(111).
- Looijestijn J, Blom JD, Hoek HW, et al. Draining the pond and catching the fish: Uncovering the ecosystem of auditory verbal hallucinations. Neuroimage Clin. 2018;20:830–843.
- Bohlken MM, Hugdahl K, Sommer IE. Auditory verbal hallucinations: neuroimaging and treatment. Psychol Med. 2017;47(2):199–208.
- Alderson-Day B, Diederen K, Fernyhough C, et al. Auditory hallucinations and the brain’s resting-state networks: findings and methodological observations. Schizophr Bull. 2016;42(5):1110–1123.
- Johns LC, Kompus K, Connell M, et al. Auditory verbal hallucinations in persons with and without a need for care. Schizophr Bull. 2014;40(Suppl_4):S255–S64.
- de Leede-Smith S, Barkus E. A comprehensive review of auditory verbal hallucinations: lifetime prevalence, correlates and mechanisms in healthy and clinical individuals. Front Hum Neurosci. 2013;7:367
- Garrison JR, Fernyhough C, McCarthy-Jones S, et al. Paracingulate Sulcus Morphology and Hallucinations in Clinical and Nonclinical Groups. Schizophr Bull. 2019:45(4):733–741.
- Spray A, Beer AL, Bentall RP, et al. Microstructure of the superior temporal gyrus and hallucination proneness - a multi-compartment diffusion imaging study. Neuroimage Clin. 2018;20:1–6.
- Zhang X, Gao J, Zhu F, et al. Reduced white matter connectivity associated with auditory verbal hallucinations in first-episode and chronic schizophrenia: A diffusion tensor imaging study. Psychiatry Res Neuroimaging. 2018;273:63–70.
- Leroux E, Delcroix N, Dollfus S. Abnormalities of language pathways in schizophrenia patients with and without a lifetime history of auditory verbal hallucinations: a DTI-based tractography study. World J Biol Psychiatry. 2017;18(7):528–538.
- Psomiades M, Fonteneau C, Mondino M, Luck D, et al. Integrity of the arcuate fasciculus in patients with schizophrenia with auditory verbal hallucinations: A DTI-tractography study. Neuroimage Clin. 2016;12:970–975.
- McCarthy-Jones S, Oestreich LK, Whitford TJ. Reduced integrity of the left arcuate fasciculus is specifically associated with auditory verbal hallucinations in schizophrenia. Schizophr Res. 2015;162(1–3):1–6.
- Slotema CW, Blom JD, Niemantsverdriet MBA, et al. Auditory verbal hallucinations in borderline personality disorder and the efficacy of antipsychotics: a systematic review. Front Psychiatry. 2018;9:347.
- Johnsen E, Hugdahl K, Fusar-Poli P, et al. Neuropsychopharmacology of auditory hallucinations: insights from pharmacological functional MRI and perspectives for future research. Expert Rev Neurother. 2013;13(1):23–36.
- Kroken RA, Johnsen E. Is rational antipsychotic polytherapy feasible? A selective review. Curr Psychiatry Rep. 2012;14(3):244–251.
- Maijer K, Palmen S, Sommer I. Children seeking help for auditory verbal hallucinations; who are they?. Schizophr Res. 2017;183:31–35.
- Bach M, Laun FB, Leemans A, et al. Methodological considerations on tract-based spatial statistics (TBSS). Neuroimage. 2014;100:358–369.
- Yin H, Xu G, Tian H, et al. The prevalence, age-of-onset and the correlates of DSM-IV psychiatric disorders in the Tianjin Mental Health Survey (TJMHS). Psychol Med. 2018;48(3):473–487.
- Yin H, Phillips MR, Wardenaar KJ, et al. The Tianjin Mental Health Survey (TJMHS): study rationale, design and methods. Int J Methods Psychiatr Res. 2017;26(3):e1535.
- Yin H, Wardenaar KJ, Xu G, et al. Help-seeking behaviors among Chinese people with mental disorders: a cross-sectional study. BMC Psychiatry. 2019;19(1):373.
- Monteschi M, Ignacio de Padua A, Riva Perez C, et al. Status of smokers. Clin Respir J. 2018;12(11):2606–2612.
- Cui Z, Zhong S, Xu P, et al. PANDA: a pipeline toolbox for analyzing brain diffusion images. Front Hum Neurosci. 2013;7:42. DOI:10.3389/fnhum.2013.00042
- Lu M, Yang C, Chu T, et al. Cerebral white matter changes in young healthy individuals with high trait anxiety: a tract-based spatial statistics study. Front Neurol. 2018;9:704.
- Smith SM, Jenkinson M, Johansen-Berg H, et al. Tract-based spatial statistics: voxelwise analysis of multi-subject diffusion data. Neuroimage. 2006;31(4):1487–1505.
- Westwood H, Stahl D, Mandy W, et al. The set-shifting profiles of anorexia nervosa and autism spectrum disorder using the Wisconsin Card Sorting Test: a systematic review and meta-analysis. Psychol Med. 2016;46(9):1809–1827.
- Walker L, Gozzi M, Lenroot R, et al. Diffusion tensor imaging in young children with autism: biological effects and potential confounds. Biol Psychiatry. 2012; 72(12):1043–1051.
- Baethge C, Jänner M, Gaebel W, et al. Psychopathological and demographic characteristics of hallucinating patients with schizophrenia and schizoaffective disorder: an analysis based on AMDP data. Eur Arch Psychiatry Clin Neurosci. 2017;267(4):295–301.
- Fovet T, Orlov N, Dyck M, et al. Translating neurocognitive models of auditory-verbal hallucinations into therapy: using real-time fMRI-neurofeedback to treat voices. Front Psychiatry. 2016;7:103.
- Ubeda-Gomez J, Leon-Palacios MG, Escudero-Perez S, et al. Relationship between self-focused attention, mindfulness and distress in individuals with auditory verbal hallucinations. Cogn Neuropsychiatry. 2015;20(6):482–488.
- Dellazizzo L, Percie Du Sert O, Phraxayavong K, et al. Exploration of the dialogue components in Avatar Therapy for schizophrenia patients with refractory auditory hallucinations: a content analysis. Clin Psychol Psychother. 2018;25(6):878–885.
- Craig TK, Rus-Calafell M, Ward T, et al. AVATAR therapy for auditory verbal hallucinations in people with psychosis: a single-blind, randomised controlled trial. Lancet Psychiatry. 2018;5(1):31–40.
- Dellazizzo L, Potvin S, Phraxayavong K, et al. Avatar therapy for persistent auditory verbal hallucinations in an ultra-resistant schizophrenia patient: a case report. Front Psychiatry. 2018;9:131.
- Chen X, Ji GJ, Zhu C, et al. Neural correlates of auditory verbal hallucinations in schizophrenia and the therapeutic response to theta-burst transcranial magnetic stimulation. Schizophr Bull. 2019;45(2):474–483.
- Ćurčić-Blake B, Ford JM, Hubl D, et al. Interaction of language, auditory and memory brain networks in auditory verbal hallucinations. Prog Neurobiol. 2017;148:1–20.
- Scheinost D, Tokoglu F, Hampson M, et al. Data-driven analysis of functional connectivity reveals a potential auditory verbal hallucination network. Schizophr Bull. 2019;45:415–424..
- Alderson-Day B, Lima CF, Evans S, Krishnan S, et al. Distinct processing of ambiguous speech in people with non-clinical auditory verbal hallucinations. Brain. 2017;140(9):2475–2489.
- Cui LB, Liu L, Guo F, et al. Disturbed brain activity in resting-state networks of patients with first-episode schizophrenia with auditory verbal hallucinations: a cross-sectional functional MR imaging study. Radiology. 2017;283(3):810–819.
- Zhu J, Wang C, Liu F, et al. Alterations of functional and structural networks in schizophrenia patients with auditory verbal hallucinations. Front Hum Neurosci. 2016;10:114.
- Koubeissi MZ, Fernandez-Baca Vaca G, Maciunas R, et al. A white matter tract mediating awareness of speech. Neurology. 2016;86(2):177–179.
- Chang X, Xi YB, Cui LB, et al. Distinct inter-hemispheric dysconnectivity in schizophrenia patients with and without auditory verbal hallucinations. Sci Rep. 2015;5(1):11218.
- van Tol MJ, van der Meer L, Bruggeman R, et al. Voxel-based gray and white matter morphometry correlates of hallucinations in schizophrenia: the superior temporal gyrus does not stand alone. Neuroimage Clin. 2014;4:249–257.
- Blom JD. Auditory hallucinations. Handb Clin Neurol. 2015;129:433–455.
- Lawrie SM. Are structural brain changes in schizophrenia related to antipsychotic medication? A narrative review of the evidence from a clinical perspective. Ther Adv Psychopharmacol. 2018;8(11):319–326.
- Ho BC, Andreasen NC, Ziebell S, et al. Long-term antipsychotic treatment and brain volumes: a longitudinal study of first-episode schizophrenia. Arch Gen Psychiatry. 2011;68(2):128–137.
- Andreasen NC, Liu D, Ziebell S, et al. Relapse duration, treatment intensity, and brain tissue loss in schizophrenia: a prospective longitudinal MRI study. Am J Psychiatry . 2013;170(6):609–615.
- Xi YB, Guo F, Li H, et al. The structural connectivity pathology of first-episode schizophrenia based on the cardinal symptom of auditory verbal hallucinations. Psychiatry Res Neuroimaging. 2016; 257:25–30.
- Ramasubbu R, Clark DL, Golding S, et al. Long versus short pulse width subcallosal cingulate stimulation for treatment-resistant depression: a randomised, double-blind, crossover trial. Lancet Psychiatry. 2020;7(1):29–40.
- Li CT, Cheng CM, Chen MH, et al. Antidepressant efficacy of prolonged intermittent theta burst stimulation monotherapy for recurrent depression and comparison of methods for coil positioning: a randomized, double-blind, sham-controlled study. Biol Psychiatry. 2020;87(5):443–450.