Abstract
Objective
We conducted this study to evaluate the effect of rTMS combined with rPMS on stroke patients with arm paralysis after CSCNTS.
Methods
A case-series of four stroke patients with arm paralysis, ages ranging from 39 to 51 years, that underwent CSCNTS was conducted. Patients were treated with 10 HZ rTMS on the contralesional primary motor cortex combined with 20 HZ rPMS on groups of elbow and wrist muscles for 15 days.
Results
The muscle tone of elbow flexor muscle (EFM), elbow extensor muscle (EEM), wrist flexor muscle (WFM) and flexor digitorum (FD) reduced immediately after operation followed by increasing gradually. After rehabilitation, the muscle tone of EEM and EFM reduced by 14% and 11%, respectively. There was a 13% and 45% change ratio in WFM and FD. The numeric rating scale (mean = 5.75 ± 1.71) was significantly lower (mean = 3.25 ± 1.90, t = 8.66, p = .00). Grip and pinch strength (mean = 23.65 ± 4.91; mean = 4.9 ± 0.59) were significantly higher (mean = 34.63 ± 5.23, t = −61.07, p = .00; mean = 7.1 ± 0.73, t = −13.91, p = .00).
Conclusions
The rehabilitation of stroke patients with arm paralysis after CSCNTS is a long, complicated process which includes great change of neuropathic pain, muscle tone, and muscle strength. In order to enhance the neural connection between the contralesional hemisphere and the hemiplegic limb, alleviate postoperative complications, as well as accelerate the rehabilitation process, we can consider to use rTMS combined with rPMS.
Introduction
Spastic paralysis is a common consequence of stroke and is an important contributor to long-term disability. It has been estimated that 30–60% of stroke patients are not able to voluntarily control their paretic hand, as most of them have shown a flexor spasm pattern [Citation1]. Some pharmacologic treatment options can be used to treat spastic paralysis. Additionally, stretching, fitting of splints, muscle strengthening and movement training are commonly used non-pharmacologic treatments [Citation2]. These methods either have side effects, limited effect of muscle tone, or have little effect on the function of paralyzed upper limb.
The recovery of patients with severe stroke is largely dependent on the compensatory mechanisms of unaffected hemisphere. However, only approximately 2% of motor nerve fibers in the upper and lower limbs do not cross and are innervated by the ipsilateral cerebral hemisphere, which heavily limits the compensatory effect of the contralesional brain [Citation3–7]. Through a number of studies and clinical observations, Xu et al. found that the paralyzed upper limb can be functionally connected to the contralesional (ipsilateral) hemisphere by transferring a cervical spinal nerve from the nonparalyzed side to the paralyzed side. This procedure was found to be associated with a greater improvement in function and reduction of spasticity than rehabilitation alone over a period of 12 months. In addition, physiological connectivity developed between the ipsilateral hemisphere and the paralyzed hand [Citation8]. Although studies have demonstrated that surgery can significantly relieve spasticity, improve function, muscle strength of paralyzed upper limb and functional activities from the tenth month after surgery [Citation8]. This procedure costs a lot of manpower, material and financial resources.
Repetitive transcranial magnetic stimulation (rTMS) is a widely utilized noninvasive technique that involves the use of a coil placed on the scalp in order to apply magnetic stimulation to possible target areas of the cortex to regulate neural excitability [Citation9]. Generally, high-frequency rTMS (HF-rTMS) (≥5 Hz) increases neuronal activity and cortical excitability, whereas low-frequency rTMS (LF-rTMS) (≤1 Hz) reduces neuronal activity and cortical excitability [Citation10]. The mechanism of TMS as a non-invasive stimulation technology in order to achieve treatment lies in four different aspects. One aspect is to regulate excitability of cerebral cortex and enhance synaptic plasticity. The second is to regulate the local cerebral blood flow. The third is to modulate secretion of brain-derived neurotrophic factor. Finally, the fourth is to regulate the release of neurotransmitters [Citation11–13]. In this manner, TMS can be used to improve motor function and neuropathic pain. In addition to rTMS, repetitive peripheral magnetic stimulation (rPMS) can also be used, which puts the magnetic stimulation coil on the peripheral nerve or muscle to treat spasms, urinary incontinences, dysphagia, and pain [Citation14–18].
Based on the mechanism of rTMS and rPMS, as well as the effects on brain structural remodeling and functional reorganization, we carried out this case-series analysis to evaluate the effect of rPMS on spastic limb and HF-rTMS on contralesional primary motor cortex (M1). And make it clear whether this procedure can help relieve pain, affect upper limb function by increasing excitability of contralesional cortex and accelerating the establishment of direct pathway between contralesional cerebral cortex and paralyzed limb or not.
Subjects and methods
Subjects
In total, nine stroke patients with arm paralysis had a contralateral seventh cervical nerve transfer operation in the neurosurgical department of our hospital, from which eight patients were transferred to our department for rehabilitation since April 2019. Four patients agreed to receive rTMS and rPMS in addition to traditional rehabilitation therapy. There was three females and one male, with average age of 42.75 ± 5.65 years (range of 39–51). They all suffered from hemorrhage, and their lesion sites were frontal and temporal lobe (n = 1) and basal ganglia (n = 3). Two suffered from right hemiparesis, while the other two suffered from left hemiparesis. Average time from onset to operation was 9.25 ± 2.22 months (range of 7–12). Average time from operation to rehabilitation was 12.75 ± 0.96 days (range of 12–14). All data is outlined in .
Table 1. Patient demographics.
Standard protocol approvals, registration, and patient consents
This study followed the tenets laid out by the Declaration of Helsinki, and was performed according to the guidelines of Institutional Review Board of Nanjing First Hospital.
Protocol of rTMS
The protocol of rTMS is outlined in . A magnetic device (YRD CCY-I, China) and a ‘figure of eight’ coil was employed for stimulation. The first step was to determine the patient’s resting motor threshold (RMT). RMT was defined as the minimum stimulation intensity that would cause five out of 10 motor evoked potentials greater than 50 μV in the thumb short adductor contraction when stimulating the patient’s M1 [Citation19]. The rTMS treatment was conducted with the patient lying down, relaxing the limb as much as possible, and the stimulation on M1 of contralesional hemisphere. It was stimulated at 10 Hz for a total of 780 stimulations, with 15 stimulations per train and 10 s intervals between trains. The intensity was 80% RMT of the unaffected hemisphere. This protocol was carried out one time per day for 15 days.
Protocol of rPMS
The protocol of rPMS is outlined in . The rPMS procedure is utilized to reduce spasticity of the hemiplegic upper limb. The patient is placed in a supine position with the upper extremity naturally positioned at side of the body. Stimulation is prescribed according to Modified Ashworth Scale (MAS) classification [Citation20]. Muscles with a MAS classification ≥ I+ were stimulated at 5 Hz for a total of 750 stimulations, with 15 stimulations per string and 3 s intervals between trains. If the antagonist muscle has a MAS classification ≥ I+, it is then stimulated with similar parameters. Otherwise, it is stimulated at 20 Hz for a total of 5100 stimulations, with 30 stimulations per train and 3 s intervals between trains. The intensity was 100% RMT. The coil was placed on the skin without pressure, which led to stimulation of two groups of muscles, the elbow flexor and extensor groups and wrist extensor and flexor groups, respectively. When stimulating, the therapist held the coil, thus moving each group of muscles from proximal to distal stimulation and moving one time at the rate of the duration of each train [Citation21]. The rPMS was also carried out one time per day for 15 days.
Evaluation of clinical outcomes
Muscle tone
The muscle tone of elbow flexor muscles (EFM), elbow extensor muscles (EEM), wrist flexor muscles (WFM) and flexor digitorum (FD) was evaluated on the MAS before and after operation and rehabilitation. The MAS is the most widely utilized clinical spasticity scale. There are six levels of 0, I, I+, II, III and IV, with values of 0, 1, 1.5, 2, 3 and 4, respectively.
Pain
The severity of pain of the non-hemiplegic side was assessed on a numeric rating scale (NRS) prior to and post rehabilitation. The numeric formula of 0 to 10 was utilized, instead of words, to indicate level of pain. A straight line was divided equally into 10 segments, and the degree of pain was assessed from order of 0 to 10. Higher scores indicate more pain (0 = no pain, 10 = extreme pain). The minimally clinical important difference (MCID) and minimal detectable change (MDC) for NRS is regularly reported as two points [Citation22].
Grip strength and pinch strength of un-paralyzed upper limb
Grip strength () and pinch strength () was assessed using the Elink comprehensive hand function test system (Biometrics, UK) prior to and post rehabilitation. The test was conducted in a seated position with elbow flexed at 90 degrees, and the forearm internally rotated at 90 degrees. Subject’s eyes were shielded so that they can’t see the assessment results. The grip and lateral pinch were performed with full power, while the average of three tests was taken. Higher values indicate greater muscle strength.
Data analysis
Descriptive statistics (mean ± SD) were calculated for patient demographics. The difference in grip, and pinch strength before and after treatment was first tested for normality. The Shapiro-Wilk (S-W) test was used because the sample size was 4, lying between 3 and 50. If the data conformed to a normal distribution, a paired t-test was utilized for analysis of pre/post treatment effects of grip strength, pinch strength and NRS pain rating. The Cohen’s d was calculated by G*Power version 3.1 to determine the effect size of each outcome measure. For Cohen’s d, an effect size of 0.2–0.3 was considered to be a ‘small’ effect, 0.5 to be a ‘moderate’ effect, and ≥0.8 to be a ‘large’ effect [Citation23]. All data were analyzed using SPSS version 26.0 (SPSS Inc., Chicago, IL, USA). The change in muscle tone was calculated by an average grade change ratio.
Results
The muscle tone of EFM, EEM, WFM and FD was reduced after operation, followed by increase before rehabilitation. After rehabilitation, the muscle tone of EEM and EFM reduced by 14% and 11% respectively, with 13% change ratio in WFM and 45% change ratio in FD ( and ).
Figure 3. shows the muscle tone of EEM (a), EFM (b), WFM (c) and FD (d) before and after operation, and before and 15 days after rehabilitation. All the change trends of average MAS at four time points are decreased after surgery immediately, gradually increase till to rehabilitation, and then decrease again after rehabilitation.
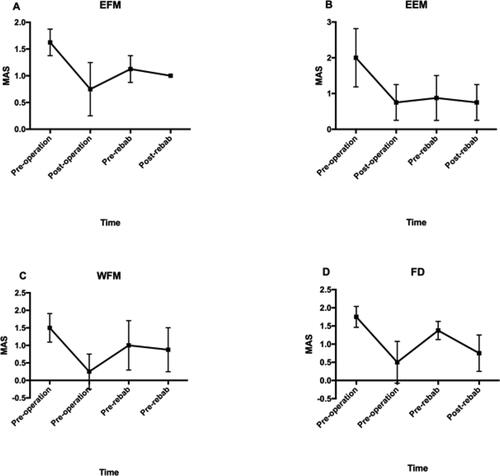
Table 2. Modified Ashworth Scale of ELM, EEM, WFM and FD before operation, after operation, before rehabilitation and 15 days after rehabilitation.
All patients experienced neuropathic pain after surgery, with pain concentrated in the shoulder, the arm and the radial side of the hand of the non-hemiplegic side. Postoperatively, some patients were treated with medications, including flurbiprofen axetil injection, celebrex and parecoxib sodium injection. However, the pain was still relatively pronounced. NRS after treatment (3.25 ± 1.89) was significantly lower compared to the pre-treatment score (5.75 ± 1.71, t = 8.66, p = .00, two-tailed). The mean decrease in NRS was 2.5 points, with a 95% CI ranging from 1.58 to 3.42. The mean difference satisfied the established MCID. The Cohen’s d effect size (d = 1.38) indicated a high level of practical significance of the treatment, and the mean change indicated that the treatment was clinically effective. The result of (S-W) test for difference in NRS is 0.73 of W score and 0.02 of p value which means the data didn’t conform to a normal distribution ().
Table 3. NRS, Grip strength and pinch strength before and after rehabilitation.
The patient complained about a reduction in grip and pinch strength on the non-hemiplegic side post-surgery. The result of (S-W) test for difference in grip strength pre/post treatment is 0.97 of W score and 0.85 of p value. The result of (S-W) test for difference in pinch strength is 0.94 of W score and 0.65 of p value. The data conformed to a normal distribution. After rehabilitation, there was some improvement in grip strength and pinch strength found by the ELINK assessment. Grip strength post-treatment (mean = 34.63 ± 5.23) was significantly higher compared to pre-treatment (mean = 23.65 ± 4.91, t = −61.07, p = .00, two-tailed). The average increase was 10.98, with a 95% CI ranging from −11.54 to −10.40. The Cohen’s d effect size (d = 2.16) indicated a high level of practical significance of the treatment. Furthermore, the mean change indicated that the treatment was clinically effective. Pinch strength after treatment (mean = 7.1 ± 0.73) was significantly higher compared to pre-treatment (mean = 4.9 ± 0.59, t = −13.91, p = .00, two-tailed). The average increase after treatment in pinch strength was 2.2, with a 95% CI ranging from −2.70 to −1.70. The Cohen’s d effect size (d = 3.29) indicated a high level of practical significance of the treatment ().
Discussion
In 1986, Gu Yudong et al. pioneered the use of CSCNTS to repair and treat brachial plexus nerve injuries, and achieved satisfactory clinical results. After decades of basic research and clinical practice, the safety and efficacy of the CSCNTS for the treatment of brachial plexus avulsion injuries has been recognized by scholars all over the world [Citation24]. Xu Wendong et al. found that motor and sensory remodeling could be induced through peripheral ‘left-right nerve displacement exchange’ surgery. In the case of the central motor system, if one hemisphere cannot be activated, the other hemisphere can be functionally remodeled to achieve central control of the upper limbs bilaterally. It was therefore hypothesized that by surgically connecting the 7th cervical nerve root of the affected limb to the contralateral one, both limbs could be co-operatively innervated by the unaffected motor cortex [Citation25]. Thus, based on this theory, Xu Wendong et al. treated 18 patients with arm paralysis after central nerve injury by using CSCNTS via the anterior vertebral approach. It was found that the patients who treated with surgery had better hand function than that with no surgery 12 months later and the affected hemisphere was significantly activated during upper limb and hand movements on the affected side. The neurosurgery team in our hospital modified this surgical approach and began a posterior vertebral approach to the stroke patients with arm paralysis from April 2019, which is based on the same principles as the classic surgical approach but significantly shortens the length of the bridging nerve. Regardless of the modality of the CSCNTS, post-operative pain, discomfort and loss of muscle strength in the unaffected upper limb, as well as some changes in muscle tone in the affected upper limb, may occur as a result of the severance of the nerve root. In addition, the ultimate goal of surgery is to achieve structural and functional reorganization of the brain which can achieve bilateral upper limb dominance by the unaffected hemisphere. However, studies have shown that this process need a considerable amount of time. Thus, it is important to find a protocol which can effectively resolve post-operative complications and facilitate bilateral upper limb dominance by unaffected hemisphere.
Neurosurgery department of our hospital has performed 9 CSCNTS of stroke patients with upper limb paralysis, in which 8 patients were transferred to our department to have a rehabilitation. Only half of them agreed with rTMS combined with rPMS treatment. Thus, we conducted this case series aimed at examining the potential effects of this protocol on stroke patients with arm paralysis after CSCNTS. Specifically, we investigated whether the treatment could suppress spasticity, alleviate neuropathic pain, and even achieve bilateral innervation of upper limbs especially paralyzed side by unaffected hemisphere.
After contralateral C7 nerve transfer, muscle tone of the paralyzed side decreased immediately post-operation and then gradually increased. The MAS scores decreased in the paralyzed elbows, wrists and fingers among all patients after surgery, which means that there is an obvious release of spasticity immediately after surgery. This release of spasticity may be a result of sectioning of the proximal C7 nerve, which contains nerve fibers from gamma motor neurons that innervate muscle spindles and maintain muscle tone [Citation8]. After surgery, the muscle tone gradually increased again until the time point when beginning rehabilitation.
The spasticity was suppressed after rehabilitation. In addition to traditional rehabilitation treatment, we also added rPMS on the elbow flexor-extensor group, the wrist and digitorum flexor muscle of the paralyzed side. rPMS is able to generate repetitive contraction-relaxation cycles that enhance the proprioceptive input from the affected limb. Additionally, rPMS stimulates action potentials in motor axons that can evoke muscle contraction. However, as it is able to penetrate into deeper regions of muscles, it has the advantages of being painless and tolerable. There are two possible mechanisms by which the muscle tone can be controlled in the MST group. The first mechanism is similar to that of neuromuscular electrical stimulation. There is a special tension receptor that is located in the normal tendon, called Golgi’s apparatus. When stimulation is applied to the spastic muscle, it will make it strongly contract. In order to avoid muscle damage caused by extensive violent contraction, this receptor is excited and the impulse is transmitted to the spinal cord through the intermediate neurons to the corresponding anterior horn cells, so that the strongly contracted muscle is reflexively inhibited. Thus, the spastic muscle is relaxed after strong stimulation. In addition, stimulating the antagonist muscle of the spastic muscle produces a cross-inhibitory effect (i.e. when a muscle is excited, its antagonist muscle will be inhibited) [Citation26]. Our study was similar to that of Krewer et al. who also applied on flexors and extensors of paralyzed elbow, wrist and fingers. They found that rPMS had short-term effects on spasticity for wrist flexors, and long-term effects for elbow extensors [Citation18]. Another study which applied rPMS on six muscle groups of upper limb found that spasticity immediately decreased in the elbow flexors and wrist flexors after just a single session of rPMS [Citation27]. Furthermore, spasticity is not a pure motor disorder, and sensory deficits have been shown to be key risk factors that are associated with the development of spasticity [Citation28]. Thus, some factors leading to increased somatosensory and proprioceptive input can inhibit spasticity. Direct activation of sensorimotor nerve fibers with orthodromic, antidromic conduction and indirect activation of mechanoreceptors (fiber groups Ia, Ib, II) during rhythmic contraction relaxation, as well as muscle vibration, are likely to be the sensory factors [Citation29].
Peripheral nerve trauma often causes severe neuropathic pain, and this pain often turns into chronic pain, which can cause abnormal pain status, such as hyperalgesia, allodynia, and spontaneous pain [Citation30]. Pathological pain can be treated using both pharmacological and non-pharmacological treatments. As the pathological mechanisms of neuropathic pain are complex, treatment also faces many challenges. Mechanisms of pathological pain likely include peripheral nociceptor sensitization, peripheral ectopic discharges, central sensitization with changes in the dorsal horn of the spinal cord, and cortical reorganization [Citation31]. Maladaptive plasticity, involving extensive neural circuit dysfunction, also plays an important role in sensitization of nociceptive pathways [Citation32–34]. It can also occur in peripheral nerves, dorsal root ganglia, spinal cord, and higher brain centers and can cause ectopic generation of action potentials, facilitation and de-inhibition of synaptic transmission, loss of synaptic connections and formation of new synaptic circuits, and neuroimmune interactions [Citation20]. Hence, therapies that promote adaptive neuroplastic changes and reverse maladaptation can be utilized to treat neuropathic pain.rTMS can modulate cortical excitability [Citation35], which can help induce motor function, and increase muscle strength [Citation36]. It can also improve cerebral blood flow [Citation37], and optimize neurotransmitter levels, including release of endogenous opioids and increasing the concentration of the neurotransmitter γ-aminobutyric acid (GABA) [Citation38]. rTMS also promotes increased secretion of brain-derived neurotrophic factors [Citation39], which ultimately causes plasticity changes in the nervous system, thus relieving pain, such as neuropathic pain [Citation36] and hemiplegic shoulder pain [Citation40].
The process of pain relief and strength improvement obtained by rTMS is assumed that the stimuli act locally within M1 can modulate the remote, deep brain structures through the subcortical fibers. The four patients had more or less neuropathic pain and different degree of muscle strength decrease due to severance of the contralateral seventh cervical nerve. Additionally, some of the patients took medications, for instance, celebrex, parecoxib sodium injection and flurbiprofen axetil injection after surgery. Although, NRS after treatment was significantly lower compared to the pre-treatment score. And the Cohen’s d effect size (d = 1.38) indicated a high level of practical significance of the treatment, and the mean change indicated that the treatment was clinically effective. The result of (S-W) test for difference in NRS is 0.73 of W score and 0.02 of p value which means the data didn’t conform to a normal distribution. The improvement of pain treated by rTMS should be interpreted with caution. After rehabilitation, there was some improvement in grip strength and pinch strength found by the ELINK assessment. The Cohen’s d effect size indicated a high level of practical significance of the treatment. Furthermore, the mean change indicated that the treatment was clinically effective. Therefore, this treatment may have some effect in improving the loss of muscle strength as a result of surgery.
Previously, HF-rTMS was applied to the M1 region of the unaffected cerebral hemisphere as the reason for the contralateral seventh cervical nerve transfer was to achieve bilateral innervation of upper limbs, especially the paralyzed side via the unaffected hemisphere.
From these results, we can see that HF-rTMS applied to M1 may help relieve pain in the contralateral limb [Citation41,Citation42]. In addition, stroke can cause a loss of inhibition of the upper motor neurons and cause spasticity, as well as a decrease in muscle strength. Recovery of function after stroke involves bilateral cerebral hemispheres, including structural remodeling and functional reorganization [Citation43,Citation44]. Thus, the contralesional hemisphere has a very important role in the accuracy and complexity of motor execution of hemiplegic upper extremity [Citation45]. However, there is little direct connection between the contralesional hemisphere and the ipsilateral limb, which limits the development of this compensatory mechanism [Citation46]. Starting eight months after contralateral seventh cervical nerve transfer, activation of the contralesional cerebral hemisphere gradually increased when the paralyzed side of the limb moved, which implies a gradual increase in the connection between contralesional hemisphere and ipsilateral limb. HF-rTMS can help promote the release of nerve growth factor and increase excitability of the cerebral cortex on the side of stimulation. Therefore, HF-rTMS of the M1 area may help accelerate the growth of peripheral nerves and enhance the connectivity between contralesional hemisphere and ipsilateral limb, which can accelerate recovery of the hemiplegic upper limb.
Unfortunately, as the procedure required immobilization of the cervical and shoulder joints for at least three weeks post-operative, a full functional assessment of the hemiplegic upper limb and hand was not possible, and regular rehabilitation of the hemiplegic limb consisted mainly of passive movements of the elbow and fingers and low intensity active movements. Additionally, 15 days of rehabilitation did not reveal any significant changes to active motor function. Although the purpose of the 10 Hz high-frequency rTMS applied on the M1 region of the unaffected hemisphere was not only to relieve pain on the non-hemiplegic side, but also to accelerate the establishment of contact between the affected hemisphere and both side of the upper limbs, thus improving motor function of the hemiplegic upper limb. However, due to the short observation period, these objectives were not achieved. Because of the specificity of the subjects, only four patients were included in this study. If using the Wilcoxon test, which is one of non-parametric tests according to small samples, p value of NRS, grip strength and pinch strength is 0.063, 0.068 and 0.068 respectively. At the 0.05 level, this does not meet the criterion of significance. In our exploratory study, we used parametric test, and the results preliminarily indicated that there were significant differences after treatment. Series of problems of this kind of patients may be alleviated by rTMS combined with rPMS. In future studies, in addition to increase the sample size, we need to design a control group and long-term follow-up can be conducted in order to observe improvements in motor function of the hemiplegic upper limb.
Conclusions
The rehabilitation of stroke patients with arm paralysis after contralateral seventh cervical nerve transfer is a long and complicated process, and includes the onset and relief of neuropathic pain, reduction and subsequent improvement of muscle tone, and loss and gain of muscle strength. In order to enhance the neural connection between the contralesional hemisphere and hemiplegic limb, alleviate postoperative complications and accelerate the rehabilitation process, we can try to use rTMS combined with rPMS.
Disclosure Statement
No potential conflict of interest was reported by the authors.
2020 Nanjing Health Science and Technology Development Special Fund Project.
References
- Wissel J, Manack A, Brainin M. Toward an epidemiology of poststroke spasticity. Neurology. 2013;80(3 Suppl 2):S13–S19.
- Bethoux F. Spasticity management after Stroke. Phys Med Rehabil Clin N Am. 2015;26(4):625–639.
- Cicinelli P, Pasqualetti P, Zaccagnini M, et al. Interhemispheric asymmetries of motor cortex excitability in the postacute stroke stage: a paired-pulse transcranial magnetic stimulation study. Stroke. 2003;34(11):2653–2658.
- Buetefisch CM. Role of the contralesional hemisphere in post-stroke recovery of upper extremity motor function. Front Neurol. 2015;6:214.
- Payne BR, Lomber SG. Reconstructing functional systems after lesions of cerebral cortex. Nat Rev Neurosci. 2001;2(12):911–919.
- Hammerbeck U, Hoad D, Greenwood R, et al. The unsolved role of heightened connectivity from the unaffected hemisphere to paretic arm muscles in chronic stroke. Clin Neurophysiol. 2019;130(5):781–788.
- Shimizu T, Hosaki A, Hino T, et al. Motor cortical disinhibition in the unaffected hemisphere after unilateral cortical stroke. Brain. 2002;125(Pt 8):1896–1907.
- Zheng MX, Hua XY, Feng JT, et al. Trial of contralateral seventh cervical nerve transfer for spastic arm paralysis. N Engl J Med. 2018;378(1):22–34.
- Barker AT, Jalinous R, Freeston IL. Non-invasive magnetic stimulation of human motor cortex. Lancet. 1985;1(8437):1106–1107.
- Hong Y, Liu Q, Peng M, et al. High-frequency repetitive transcranial magnetic stimulation improves functional recovery by inhibiting neurotoxic polarization of astrocytes in ischemic rats. J Neuroinflammation. 2020;17(1):150.
- Park E, Kang MJ, Lee A, et al. Real-time measurement of cerebral blood flow during and after repetitive transcranial magnetic stimulation: a near-infrared spectroscopy study. Neurosci Lett. 2017;653:78–83.
- Wang HY, Crupi D, Liu J, et al. Repetitive transcranial magnetic stimulation enhances BDNF-TrkB signaling in both brain and lymphocyte. J Neurosci. 2011;31(30):11044–11054.
- Zhang N, Xing M, Wang Y, et al. Repetitive transcranial magnetic stimulation enhances spatial learning and synaptic plasticity via the VEGF and BDNF-NMDAR pathways in a rat model of vascular dementia. Neuroscience. 2015;311:284–291.
- Renner T, Sollmann N, Heinen F, et al. Alleviation of migraine symptoms by application of repetitive peripheral magnetic stimulation to myofascial trigger points of neck and shoulder muscles - a randomized trial. Sci Rep. 2020;10(1):5954.
- Vadalà M, Palmieri B, Malagoli A, et al. High-power magnetotherapy: a new weapon in urinary incontinence? Low Urin Tract Symptoms. 2018;10(3):266–270.
- Massé-Alarie H, Beaulieu LD, Preuss R, et al. Repetitive peripheral magnetic neurostimulation of multifidus muscles combined with motor training influences spine motor control and chronic low back pain. Clin Neurophysiol. 2017;128(3):442–453.
- Momosaki R, Abo M, Watanabe S, et al. Functional magnetic stimulation using a parabolic coil for dysphagia after stroke. Neuromodulation. 2014;17(7):637–641; discussion 41.
- Krewer C, Hartl S, Müller F, et al. Effects of repetitive peripheral magnetic stimulation on upper-limb spasticity and impairment in patients with spastic hemiparesis: a randomized, double-blind, sham-controlled study. Arch Phys Med Rehabil. 2014;95(6):1039–1047.
- Wang Q, Zhang D, Zhao Y-Y, et al. Effects of high-frequency repetitive transcranial magnetic stimulation over the contralesional motor cortex on motor recovery in severe hemiplegic stroke: a randomized clinical trial. Brain Stimul. 2020;13(4):979–986.
- Li F, Wu Y, Li X. Test-retest reliability and inter-rater reliability of the Modified Tardieu Scale and the Modified Ashworth Scale in hemiplegic patients with stroke. Eur J Phys Rehabil Med. 2014;50(1):9–15.
- Beaulieu LD, Schneider C. Effects of repetitive peripheral magnetic stimulation on normal or impaired motor control. A review. Neurophysiol Clin. 2013;43(4):251–260.
- Childs JD, Piva SR, Fritz JM. Responsiveness of the numeric pain rating scale in patients with low back pain. Spine (Phila PA 1976). 2005;30(11):1331–1334.
- Cohen J. Statistical power analysis for the behavioral sciences. Hillsdale (NJ): L Erlbaum Associates; 1998.
- Gu YD, Zhang GM, Chen DS, et al. Seventh cervical nerve root transfer from the contralateral healthy side for treatment of brachial plexus root avulsion. J Hand Surg. 1992;17(5):518–521.
- Wang M, Li ZY, Xu WD, et al. Sensory restoration in cortical level after a contralateral C7 nerve transfer to an injured arm in rats. Neurosurgery. 2010;67(1):136–143.
- Alfieri V. Electrical treatment of spasticity. Reflex tonic activity in hemiplegic patients and selected specific electrostimulation. J Scandinavian J Rehabil Med. 1982;14(4):177.
- Chen S, Li Y, Shu X, et al. Electroencephalography Mu rhythm changes and decreased spasticity after repetitive peripheral magnetic stimulation in patients following stroke. Front Neurol. 2020;11:546599.
- Pandyan AD, Gregoric M, Barnes MP, et al. Spasticity: clinical perceptions, neurological realities and meaningful measurement. Disabil Rehabil. 2005;27(1–2):2–6.
- Heldmann B, Kerkhoff G, Struppler A, et al. Repetitive peripheral magnetic stimulation alleviates tactile extinction. Neuroreport. 2000;11(14):3193–3198.
- Kretzschmar M, Reining M, Schwarz MA. Three-year outcomes after dorsal root ganglion stimulation in the treatment of neuropathic pain after peripheral nerve injury of upper and lower extremities. Neuromodulation. 2021;24(4):700–707.
- Lovaglio AC, Socolovsky M, Di Masi G, et al. Treatment of neuropathic pain after peripheral nerve and brachial plexus traumatic injury. Neurol India. 2019;67(Supplement):S32–S37.
- Pfannmöller J, Strauss S, Langner I, et al. Investigations on maladaptive plasticity in the sensorimotor cortex of unilateral upper limb CRPS I patients. Restor Neurol Neurosci. 2019;37(2):143–153.
- Lotze M. Maladaptive plasticity in chronic and neuropathic pain. Schmerz. 2016;30(2):127–133.
- Kumru H, Albu S, Vidal J, et al. Effectiveness of repetitive trancranial or peripheral magnetic stimulation in neuropathic pain. Disabil Rehabil. 2017;39(9):856–866.
- Thomson AC, Kenis G, Tielens S, et al. Transcranial magnetic stimulation-induced plasticity mechanisms: TMS-related gene expression and morphology changes in a human neuron-like cell model. Front Mol Neurosci. 2020;13:528396.
- Hosomi K, Sugiyama K, Nakamura Y, For the TEN-P11-01 Investigators, et al. A randomized controlled trial of 5 daily sessions and continuous trial of 4 weekly sessions of repetitive transcranial magnetic stimulation for neuropathic pain. Pain. 2020;161(2):351–360.
- Mesquita RC, Faseyitan OK, Turkeltaub PE, et al. Blood flow and oxygenation changes due to low-frequency repetitive transcranial magnetic stimulation of the cerebral cortex. J Biomed Opt. 2013;18(6):067006.
- Tremblay S, Beaulé V, Proulx S, et al. Relationship between transcranial magnetic stimulation measures of intracortical inhibition and spectroscopy measures of GABA and glutamate + glutamine. J Neurophysiol. 2013;109(5):1343–1349.
- Zhao X, Li Y, Tian Q, et al. Repetitive transcranial magnetic stimulation increases serum brain-derived neurotrophic factor and decreases interleukin-1β and tumor necrosis factor-α in elderly patients with refractory depression. J Int Med Res. 2019;47(5):1848–1855.
- Choi GS, Chang MC. Effects of high-frequency repetitive transcranial magnetic stimulation on reducing hemiplegic shoulder pain in patients with chronic stoke: a randomized controlled trial. Int J Neurosci. 2018;128(2):110–116.
- Malavera A, Silva FA, Fregni F, et al. Repetitive transcranial magnetic stimulation for phantom limb pain in land mine victims: a double-blinded, randomized, sham-controlled trial. J Pain. 2016;17(8):911–918.
- Quesada C, Pommier B, Fauchon C, et al. Robot-guided neuronavigated repetitive transcranial magnetic stimulation (rTMS) in Central neuropathic pain. Arch Phys Med Rehabil. 2018;99(11):2203–2215.e1.
- Lee J, Lee M, Kim DS, et al. Functional reorganization and prediction of motor recovery after a stroke: a graph theoretical analysis of functional networks. Restor Neurol Neurosci. 2015;33(6):785–793.
- Lee J, Park E, Lee A, et al. Recovery-related indicators of motor network plasticity according to impairment severity after stroke. Eur J Neurol. 2017;24(10):1290–1299.
- Barany DA, Revill KP, Caliban A, et al. Primary motor cortical activity during unimanual movements with increasing demand on precision. J Neurophysiol. 2020;124(3):728–739.
- Bundy DT, Leuthardt EC. The cortical physiology of ipsilateral limb movements. Trends Neurosci. 2019;42(11):825–839.