Abstract
The in vitro rearing of worker honey bees (Apis mellifera L.) has become an increasingly important method in honey bee research in general, and in pesticide risk assessment specifically. Authorities from the European Organization for Economic Co-operation and Development and the United States Environmental Protection Agency are requesting data on pesticide impacts on immature bee survivorship prior to registering new crop protection products. Those using the current in vitro rearing protocols have had variable success with immature bee survival and protocol repeatability. Here, we present an improved method for the in vitro rearing of worker honey bees from larvae to adult emergence. We have achieved consistently high survival (>95%) in our control and solvent-control rearing trials. Changes in the proportion of diet components, royal jelly source, maintenance of the developing bee, and rearing environment are the main contributors for our high rearing success and are discussed herein. Our in vitro rearing protocol can be implemented as the standard protocol to determine the impact of pesticides on immature bees because of the protocol’s high control survivability, ease in end point determination, and high overall repeatability.
La cría in vitro de las abejas obreras (Apis mellifera L.) se ha convertido en un método cada vez más importante en la investigación de la abeja de la miel en general, y específicamente, en la evaluación del riesgo de los plaguicidas. Las autoridades de la Organización Europea para la Cooperación y el Desarrollo, y la Agencia de Protección Ambiental de los Estados Unidos están solicitando datos sobre los efectos de los plaguicidas en la supervivencia de abejas inmaduras antes de registrar nuevos productos para la protección de los cultivos. Aquellos que utilizan actualmente los protocolos de la cría in vitro han tenido un éxito variable en la supervivencia de abejas inmaduras y la repetitividad del protocolo. Aquí presentamos un método mejorado para la cría in vitro de las abejas obreras a partir de larvas para la eclosión de los adultos. Hemos logrado consistentemente una alta supervivencia (>95%) en nuestro control y en los ensayos de cría con el disolvente del control. Los cambios en la proporción de los componentes de la dieta, la fuente de jalea real, el mantenimiento de las abejas en desarrollo, y el ambiente de cría son los principales constituyentes para nuestro alto éxito de cría y son discutidos en este trabajo. Nuestro protocolo de cría in vitro puede ser implementado como el protocolo estándar para determinar el impacto de los plaguicidas en las abejas inmaduras debido a la alta capacidad de supervivencia del control, la facilidad en la determinación del punto final, y la alta capacidad de repetitividad.
Introduction
The western honey bee (Apis mellifera L.) is an important pollinator species worldwide (Gallai, Salles, Settele, & Vaissière, Citation2009; Klein et al., Citation2007). Pesticides, nutrition, parasites, and/or diseases are thought to be major contributors to recent honey bee declines (Fairbrother, Purdy, Anderson, & Fell, Citation2014; National Agricultural Statistics Service, Citation2015; Neumann & Carreck, Citation2010; Staveley, Law, Fairbrother, & Menzie, Citation2014; Vanbergen et al., Citation2013). However, it is difficult to isolate the adverse impact of most of these stressors on honey bee health, particularly on immature honey bee health, because of the complexity associated with investigating multiple stressors simultaneously and the lack of quality, repeatable bioassays with which to do the work.
Substantial progress has been made with bioassays that can be used to rear immature honey bees in the laboratory over the past few decades (Aupinel et al., Citation2005; Crailsheim et al., Citation2013; Huang, Citation2009; Peng, Mussen, Fong, Montague, & Tyler, Citation1992; Rembold & Lackner, Citation1981; Vandenberg & Shimanuki, Citation1987). The in vitro methodology for workers was initially used to investigate queen/worker differentiation, nutrition, and pathogen impacts on immature bees (Rembold & Lackner, Citation1981; Vandenberg & Shimanuki, Citation1987). Rembold and Lackner (Citation1981) were among the first to feed larvae a balanced diet of sugars, royal jelly, water, and yeast in order to rear larvae successfully within the laboratory. Vandenberg and Shimanuki (Citation1987) further improved the in vitro methodology by optimizing diet composition and the amount of provisioned diet, both of which yielded higher rates of adult emergence and less queen intermediates. Peng et al. (Citation1992) began utilizing the methodology for determining the toxicity of pesticides to developing honey bees. Other rearing strategies have been developed (Hendriksma, Härtel, & Steffan‐Dewenter, Citation2011b; Huang, Citation2009). Most notably, Aupinel et al. (Citation2005) further refined the methodology in a way that is easily adaptable to current regulatory requirements for accessing the toxicity of a target substance to developing honey bees. Their methodology was largely based on Vandenberg and Shimanuki (Citation1987), but further refined the diet composition and amount being provisioned to the larvae, used plastic queen cells, and reduced the handling time by only feeding the larvae once daily.
The advancements in the in vitro rearing protocol have given government, industry, and academia a new tool for accessing the risks pesticides and other chemicals pose to immature honey bee development and survival (Aupinel et al., Citation2005, Citation2007, Citation2009; Crailsheim et al., Citation2013; Hendriksma, Härtel, & Steffan‐Dewenter, Citation2011b; Huang, Citation2009; Peng et al., Citation1992; Zhu, Schmehl, Mullin, & Frazier, Citation2014). Honey bee in vitro rearing protocols have allowed researchers to standardize environmental conditions (e.g. temperature, humidity) in which the immature bees develop, provide a uniform diet to each individual, and reduce pathogen exposure.
There are some examples of successes using the Aupinel et al. (Citation2005) methodology, particularly related to high survival throughout the larval stage of development. Hendriksma, Härtel, and Steffan-Dewenter (Citation2011a) and Hendriksma, Härtel, Babendreier, von der Ohe, and Steffan-Dewenter (Citation2012) demonstrated control bee survival of >90% up to the termination of the test at the prepupal stage of development. Krainer, Brodschneider, Vollmann, Crailsheim, and Riessberger-Gallé (Citation2016) were able to achieve high survival through adult emergence, but this level of success has historically been atypical. Other ad libitum (Huang, Citation2009) and non-grafting (Hendriksma, Härtel, & Steffan‐Dewenter, Citation2011b) rearing protocols yielded near 80% survival from the time of larva inclusion in the study to adult emergence but they have not been adopted for regulatory testing.
Despite advancements in the larval rearing protocol, there continues to be substantial difficulty in achieving consistent survival in untreated individuals and test reproducibility with existing protocols. Total bee survival from 48 h (2 days) must be 85% overall 7 days after grafting and 70% overall to adult emergence per thresholds set by regulatory agencies (OECD, Citation2014). Otherwise the data generated from those studies are not considered valid.
Generally speaking, consistently high control survival has been hard for investigators to achieve. In a 2005 and 2008 ring test, individuals from seven institutions, all with prior larval rearing experience, across five countries only achieved a rate of adult emergence greater than 80% in 43% of their control trials and greater than 90% in 17% of their control trials (Aupinel et al., Citation2009). Furthermore, several recently published studies based upon current protocols (Aupinel et al., Citation2005, Citation2007, Citation2009) noted very high control mortality. Zhu et al. (Citation2014) reported larval control mortality six days after grafting to be over 15%. In our experience, this would translate to a poor rate of adult emergence given the already high rate of larval death which usually correlates with an equally high or higher rate of death in developing pupae. Other investigators experienced up to 60% mortality in controls prior to reaching adult emergence (Lüken et al., Citation2014). The reasons for low control survival and inconsistent results across different laboratories are unclear. Regardless, no in vitro rearing protocol developed to date yields consistent results, making them generally unfit for use as standard methodologies.
Here, we outline an improved protocol for the in vitro rearing bioassay that has allowed us to reach over 95% survival consistently in both our control and solvent treatments. We provide an updated protocol with a revised method in a step-by-step format to outline how to rear honey bee workers artificially. The Crailsheim et al. (Citation2013) COLOSS BEEBOOK publication for the artificial rearing of honey bees provides guidance for understanding the historical context of the methodology and the different rearing approaches that are utilized by various researchers. The “standardized methods” are, however, difficult to follow and interpret for a researcher who is not familiar with the methodology. Additionally, we highlight the crucial points of the rearing process that are necessary to achieve a high percentage of adult emergence with the goal of increasing rearing success worldwide and providing a higher level of resolution between data produced from rearing bees on test diets (pesticides, nutritional supplements, etc.) and control ones.
Figure 1. Plastic Chinese grafting tool (A), modified grafting tool (B), pupal transfer tool (C) and removed components from pupal transfer tool (D).
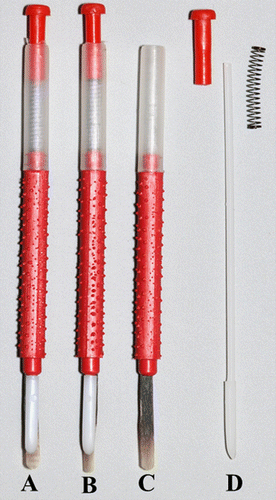
Figure 2. Larval sterile tissue culture plate with a plastic cell cup placed within each well (A), and pupal sterile tissue culture plate with a 2 × 1 cm piece of Kimwipe® placed within each well (B).
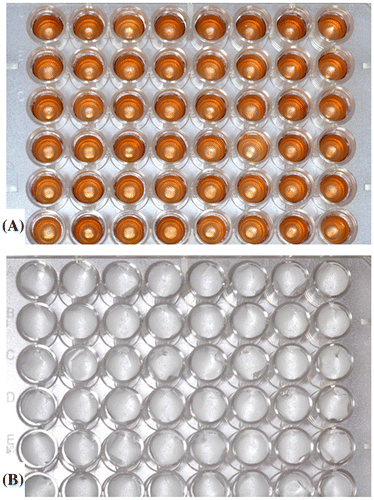
Table 1. Tools and supplies needed for the in vitro rearing protocol.
Figure 3. Incubator containing desiccators used to house larval and pupal sterile tissue culture plates (A), and positive flow hood used for in vitro larval grafting, feeding, and monitoring for mortality (B).
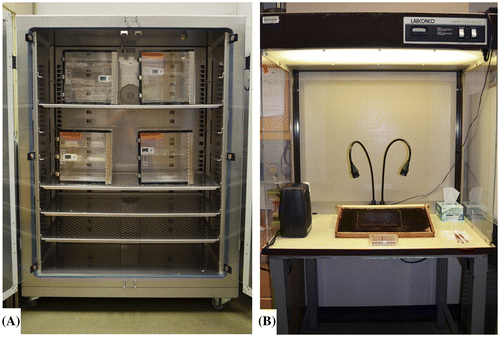
Figure 4. Push-in zinc queen excluder cage (A) and position of queen excluder cage on a honey bee hive frame (B).
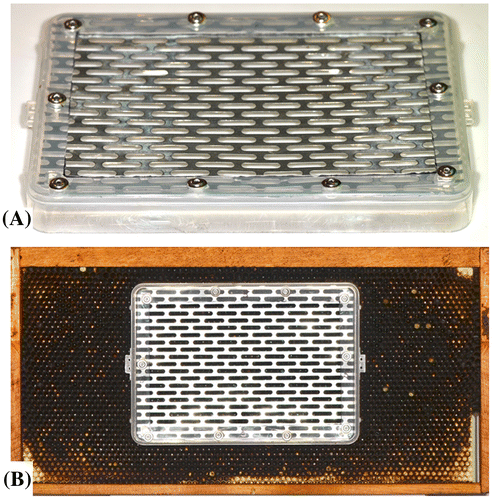
Table 2. Amount and percentage of diet components in the larval diet necessary to feed approximately 400 larvae.
Table 3. In vitro rearing time reference points.
Table 4. Schedule of larval feeding during the in vitro protocol.
Figure 5. Larval development from the day of grafting (A, t = 3.625 ± 0.5 d), one day (B, t = 4.625 ± 0.5 d), two days (C, t = 5.625 ± 0.5 d), three days (D, t = 6.625 ± 0.5 d), four days post graft (E, t = 7.625 ± 0.5 d), five days (F, t = 8.625 ± 0.5 d), and six days (G, t = 9.625 ± 0.5 d) post grafting. The prepupa in G has fully consumed its diet and is ready to be transferred into the pupal plate. All pictures were taken prior to any scheduled feedings.
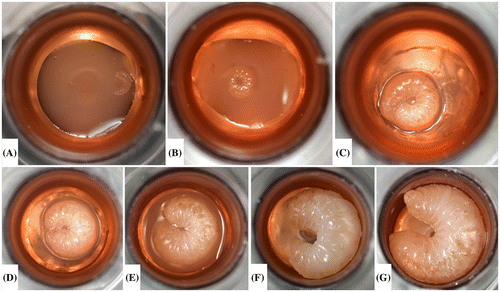
Figure 6. Young larvae in frame prior to grafting (A), larva on the filament tip of a Chinese grafting tool while grafting from the frame to the cell cup (B) and larva within cell cup after grafting (C).
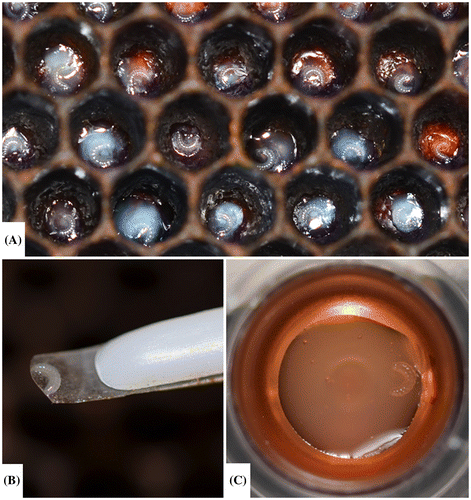
Figure 7. The appearance of a dead larva has a characteristic sunken in appearance and excess diet within the well.
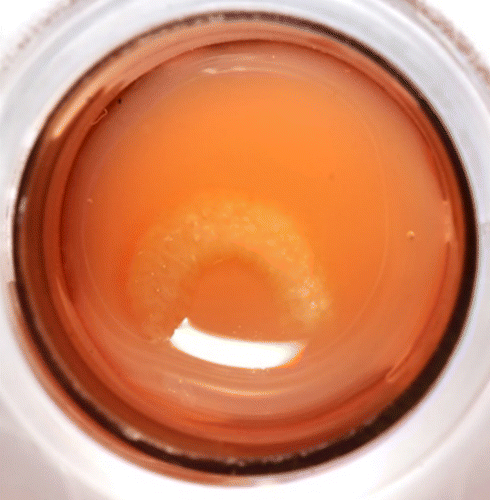
Figure 8. Pupal development from immediately after the pupal transfer (A) through adult emergence (H). Some days during the pupal development stage are not pictured. Note the pigmented color of the eyes and the darkening cuticle as the pupating bee continues to develop within the pupal STCP. A piece of Kimwipe® lines the bottom of each well.
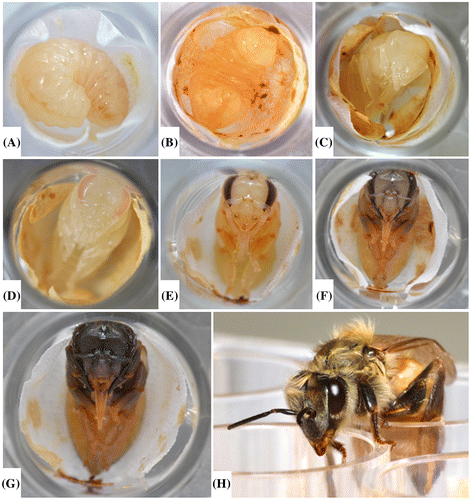
Figure 9. Survival plots of honey bee workers reared in vitro and fed just the diet or the diet containing solvents (water and acetone) used to dissolve pesticides. The survival of larvae moved prior to and after defecation was evaluated. Differences in the survival between bees moved prior to and after defecation were detected using a LOG RANK test. Survival curves grouped by the same vertical bar on the right are not significantly different per Holm-Sidak’s test (p > 0.05).
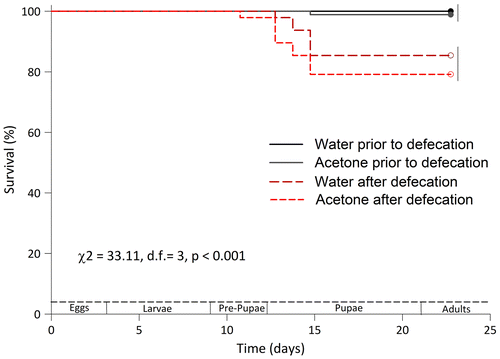
Protocol
(1) | Prepping tools/supplies- A list of all equipment and supplies is provided in Table . They must be acquired and prepared as discussed below before initiating the in vitro rearing protocol.
| ||||||||||||||||||||||||||||||||||||||||||||||||||||||||||||||||||||||||||||||||||||||||||||||||||||||||||||||||||||||||||||||||||||||||||||||||||||||||||||||||
(2) | Preparing the larval diet
| ||||||||||||||||||||||||||||||||||||||||||||||||||||||||||||||||||||||||||||||||||||||||||||||||||||||||||||||||||||||||||||||||||||||||||||||||||||||||||||||||
(3) | Collection of larvae
| ||||||||||||||||||||||||||||||||||||||||||||||||||||||||||||||||||||||||||||||||||||||||||||||||||||||||||||||||||||||||||||||||||||||||||||||||||||||||||||||||
(4) | Larval grafting and maintenance (Figure )
| ||||||||||||||||||||||||||||||||||||||||||||||||||||||||||||||||||||||||||||||||||||||||||||||||||||||||||||||||||||||||||||||||||||||||||||||||||||||||||||||||
(5) | Pupae transfer and maintenance (Figure )
| ||||||||||||||||||||||||||||||||||||||||||||||||||||||||||||||||||||||||||||||||||||||||||||||||||||||||||||||||||||||||||||||||||||||||||||||||||||||||||||||||
(6) | Adult emergence
| ||||||||||||||||||||||||||||||||||||||||||||||||||||||||||||||||||||||||||||||||||||||||||||||||||||||||||||||||||||||||||||||||||||||||||||||||||||||||||||||||
(7) | Measurable endpoints for risk assessment
|
Discussion
With our methods, we have optimized the success of honey bee in vitro larval rearing to adult emergence. From our experiences, we detail below five critical areas for achieving low mortality rates and high reproducibility: (1) larval diet source and composition; (2) grafting environment; (3) plate conditions; (4) rearing environment; and (5) prepupal transfer. We did not measure the individual contribution of each step, but the collective sum translated into excellent survival and adult emergence rates of >95%, neither of which has been achieved consistently before, the latter being indicated in published reports where the various in vitro rearing protocols were used.
Larval diet source and composition
The most important considerations with the larval diet are quality components, the percentage of each ingredient in the diet, and a properly vetted royal jelly source and batch. One of the main differences between our larval diet recipe and those listed in previous protocols (Aupinel et al., Citation2005; Crailsheim et al., Citation2013) are the increase in the water content and a reduction in the royal jelly content of diets A and B. We found that the diets noted in the literature (Aupinel et al., Citation2005; Crailsheim et al., Citation2013) were prone to drying out in the experimental conditions under which we reared larvae. Consequently, we increased the amount of water added to our diets over that recommended by Aupinel et al. (Citation2005) and Crailsheim et al. (Citation2013). Larvae fed the new, modified diet never appeared to develop into queen-worker intermediate castes and the diet never appeared to dry, however we did not conduct any detailed caste analyses. Geometric morphometrics, which extracts spatial information from morphological structures (De Souza et al., Citation2015) could be used in future studies to confirm that the in vitro workers were indeed workers and not intercastes.
One of the difficulties in including royal jelly in a standardized larval rearing protocol is that royal jelly composition varies from one royal jelly source and batch to the next (Sabatini, Marcazzan, Caboni, Bogdanov, & Almeida-Muradian, Citation2009; Zheng, Hu, & Dietemann, Citation2010). We discovered that the source of royal jelly is critical in larval rearing success. In pilot experiments (data not shown), royal jelly sourced from different companies yielded different rates of immature bee survival to adult emergence. Survivorship of the developing bees in our study using the same protocol but otherwise different batches of royal jelly varied from 25 to 100%. In our case, we achieved high levels of reproducibility and adult emergence using Stakich brand royal jelly which is sourced and sold in Michigan, USA. It is crucial to compare the survival rates from immature honey bees to adult emergence using different batches of royal jelly sourced from different companies. We set aside a small amount of royal jelly from a Stakich batch that yielded high immature bee survivability rates. We, then, compared survival rates resulting from larvae feeding on all royal jelly we acquired for future studies to the batch we knew produced high rates of survival.
Be sure the royal jelly is maintained properly during transit and storage. All royal jelly should be shipped via overnight delivery, placed in 30–50 ml aliquots upon arrival, and stored at −20 °C. This may have to be negotiated with the supplier as most royal jelly is sold for human consumption that, otherwise, does not have the same shipping requirements. After receiving and opening the royal jelly, we recommend splitting the batch among several small (~30–50 ml) vials. This will limit the freeze/thaw cycle associated with using royal jelly from a single batch when needed. This way, only the number of vials needed can be thawed for use. The vial of working royal jelly stock, i.e. the royal jelly made ready for immediate use, can be maintained within the fridge at 4 °C for up to a month.
Grafting environment
It is important to maintain the right grafting environment to ensure high survivorship using the protocol. First instar larvae are incredibly sensitive to temperature fluctuations and other disturbances when they are transported into the laboratory for grafting. We recommend ensuring that the area where grafting will occur is heated sufficiently (~31 °C). We speculate that the introduction of pathogens is limited by grafting in a positive-pressure clean hood. The grafter always should be mindful of sterility and wear a dust mask and gloves while grafting.
Plate conditions
Plate conditions also are an important part of obtaining high survivorship using the protocol. The plate conditions dictate the environment encountered by the bees during their development. Always keep the cover on the larval and pupal STCPs except when the STCPs are in the clean hood. Keeping the STCPs closed helps prevent contamination and maintain temperature and humidity. Furthermore, the introduction of pathogens into the rearing environment is reduced by keeping the lid on the larval STCP while moving the plate between the hood and the incubator. It is not necessary to apply glycerol solution to the cotton placed in the plates, below the cell cups, though others have suggested that it is necessary to maintain humidity within the larval STCP (Aupinel et al., Citation2005, Citation2009; Crailsheim et al., Citation2013; OECD, Citation2014).
Rearing environment
A controlled rearing environment prevents the developing bees from being adversely affected by shock throughout their development into an adult bee. An incubator with less than 1.0 °C fluctuation over a 24-h period will maintain a consistent rearing temperature and is especially necessary if one is using the rearing protocol to measure the impacts of pesticides on bees. Medrzycki et al. (Citation2010) demonstrated that small fluctuations in temperature can impact a pesticide’s toxicity to developing bees. Temperature and relative humidity are controlled further by placing the STCP within desiccator chambers. Removing the desiccator chambers from the incubator while a test is being performed is an unnecessary disturbance and may reduce bee survival substantially.
Prepupal transfer
The fifth critical consideration for achieving low mortality rates and high reproducibility with the in vitro rearing protocol is the timing of prepupal transfer. The larvae are transferred to a new pupal STCP once the larval diet is consumed completely (approximately t = 9.625 ± 0.5 d, or 6 days after grafting). Our results indicate that leaving the pupae in the same STCP in which they develop may decrease the rate of adult emergence and stunt bee growth. We believe that it is important for bee survival to transfer the developing bee to the pupal STCP after the bee fully consumes the diet but before she defecates. After transferring the larva to the pupal STCP, the bee defecates while it rests on the Kimwipe. The Kimwipe quickly absorbs the fecal waste and reduces contact between the bee and its waste. Increased mortality in the pupal stage of development noted using previous protocols (Aupinel et al., Citation2005; Crailsheim et al., Citation2013) may be associated with the contact between the developing bee and its feces. Furthermore, bees reared using our protocol did not display any adverse morphological deformations such as stunted growth or shortened abdomens, as has been reported by others (Brodschneider, Riessberger-Gallé, & Crailsheim, Citation2009; Riessberger-Gallé, Vollmann, Brodschneider, Aupinel, & Crailsheim, Citation2008). We saw no indication of a reduction in bee survivorship when manually transferring the bees to a pupal STCP at the end of their larval development.
Additional considerations
The selection of suitable colonies in the apiary can improve the success of the rearing methods. We have preliminary data that suggest survivorship in the in vitro rearing protocol may correlate partially with the colony from which the larvae were grafted. Only colonies that have functional laying queens that are producing solid brood patterns (<10% empty cells in the pattern), are free of symptomatic diseases or pests, and are not undergoing any treatments for pests/pathogens at protocol initiation should be used for acquiring young larvae.
Queens occasionally escape or do not lay eggs while caged. The type of push-in cage used in our proposed method works best with plastic foundation to reduce the possibility of an escaped queen. Regardless, for time-sensitive trials, it is advisable to cage multiple queens in the event that one fails to lay eggs or otherwise escapes.
Our proposed method produced a consistent rate of survival over 95% in both our control and solvent groups. The validity criteria for the proposed OECD repeated-exposure (chronic) larval test guideline in Europe is 85% survival from 72 h (3 d) after grafting until 168 h (7 d) after grafting and 70% survival to adult emergence (OECD, Citation2014). These high levels of mortality are problematic when resolving potential toxic effects of a test compound on bee larvae. Furthermore, the OECD guidelines permit the tester to replace any dead larvae with living ones at 48 h (2 d) after grafting, immediately before administering diet B (OECD, Citation2014). We did not have to do this as the survivorship in our larvae was high throughout the study. Thus, our protocol permits the user to begin administering pesticides to the very first diet provided to the grafted larvae rather than 2–3 d after grafting the larvae as required via other protocols. We believe this makes our protocol useful for testing the chronic impact of pesticides on developing bees.
The use of alternative in vitro rearing methods has yielded variable rates of success (Hendriksma, Härtel, & Steffan‐Dewenter, Citation2011b; Huang, Citation2009). Non-grafting methods (queen eggs laid directly into artificial comb within the hive that are used throughout the rearing protocol) have resulted in mortality rates between 2 and 8% in larvae and adult emergence rates near 80% (Hendriksma, Härtel, & Steffan‐Dewenter, Citation2011b). While limiting direct human interference with developing bees may yield a higher rate of survival than Aupinel et al. (Citation2007) experienced using their protocols, our results indicate that human interference does not cause a reduction in bee survival directly given that we transferred our post-food consumption larvae to new plates. Huang (Citation2009) suggests ad libitum feeding during the larval development stage. They experienced an adult emergence rate of approximately 80% from the time of grafting. However, ad libitum feeding makes it impossible to determine the level of bee exposure to a test substance provided in the larval diet. Our current protocol allows for the dose of a test solution to be calculated for bees consuming the total diet provided, i.e. 160 μl of diet. The dose cannot be determined for bees that fail to survive until the end of the larval stage since the diet is not consumed completely by the larvae prior to death.
High mortality rates and difficulty reproducing quality results within and between laboratories may not be related entirely to methods, but be attributed to experimental grafting error, temporal and spatial effects, genetic variation in the bee source, pathogens, and larval size at grafting. Furthermore, there are several unknowns in key parts of any in vitro rearing protocol including royal jelly quality and the functionality of in vitro reared workers. Royal jelly has some remarkable biological activities (Viuda-Martos, Ruiz-Navajas, Fernández-López, & Pérez-Álvarez, Citation2008), but the percentage of certain royal jelly components may vary across different geographic regions (Wei et al., Citation2013). It is necessary to import royal jelly in some countries and customs may require the irradiation of royal jelly which may alter any potential beneficial microbe community within the diet or otherwise impact diet quality.
Few have studied the functionality of in vitro reared worker bees (Brodschneider et al., Citation2009). Some protocols (Huang, Citation2009) are prone to producing worker-queen intercastes which may not be functional in the hive. Brodschneider et al. (Citation2009) observed similar flight performances between artificially reared and colony-reared honey bees, but in the future, investigators using our improved protocol can explore the functionality of in vitro reared workers by comparing anatomical, behavioral, and physiological parameters of in vitro- and hive-reared worker honey bees.
We have discussed an improved protocol for the in vitro rearing of larval worker honey bees to adult emergence. Our rearing protocol produces high survivorship in control individuals and can be used to assess the potential risks of crop protection products to, the contribution of nutrition to, and the effects of brood diseases on bee health. In conclusion, our in vitro rearing protocol can be implemented as the standard protocol used to determine the impact of stressors on immature bees because of the protocol’s high control survivability, ease in end point determination, and high overall repeatability.
Disclosure statement
No potential conflict of interest was reported by the authors.
Funding
This work was supported by the Minas Gerais State Foundation for Research Aid (FAPEMIG), the National Council of Scientific and Technological Development (CNPq), the Coordenação de Aperfeiçoamento de Pessoal de Nível Superior (CAPES), and the University of Florida.
Acknowledgments
We thank Mike Bentley and Lyle Buss (University of Florida) for providing the photographs used in the manuscript, and Chris Mullin and Julia Fine (Penn State University) for providing preliminary feedback on the method.
References
- Aupinel, P., Fortini, D., Dufour, H., Tasei, J.-N., Michaud, B., Odoux, J.-F., & Pham-Delegue, M. (2005). Improvement of artificial feeding in a standard in vitro method for rearing Apis mellifera larvae. Bulletin of Insectology, 58, 107–111.
- Aupinel, P., Fortini, D., Michaud, B., Marolleau, F., Tasei, J.-N., & Odoux, J.-F. (2007). Toxicity of dimethoate and fenoxycarb to honey bee brood (Apis mellifera), using a new in vitro standardized feeding method. Pest Management Science, 63, 1090–1094. doi:10.1002/ps.1446
- Aupinel, P., Fortini, D., Michaud, B., Medrzycki, P., Padovani, E., Przygoda, D., & Riessberger-Galle, U. (2009). Honey bee brood ring-test: Method for testing pesticide toxicity on honey bee brood in laboratory conditions. Julius-Kühn-Archiv, 25, 96–100.
- Brodschneider, R., Riessberger-Gallé, U., & Crailsheim, K. (2009). Flight performance of artificially reared honey bees (Apis mellifera). Apidologie, 40, 441–449. doi:10.1051/apido/2009006
- Crailsheim, K., Brodschneider, R., Aupinel, P., Behrens, D., Genersch, E., Vollmann, J., & Riessberger-Gallé, U. (2013). Standard methods for artificial rearing of Apis mellifera larvae. In V. Dietemann, J. D. Ellis & P. Neumann (Eds.), The COLOSS BEEBOOK, Volume I: Standard methods for Apis mellifera research. Journal of Apicultural Research, 52(1), 1–16. doi:10.3896/IBRA.1.52.1.05.
- De Souza, D. A., Wang, Y., Kaftanoglu, O., De Jong, D., Amdam, G. V., Gonçalves, L. S., & Francoy, T. M. (2015). Morphometric identification of queens, workers and intermediates in in vitro reared honey bees (Apis mellifera). PLoS ONE, 10, e0123663. doi:10.1371/journal.pone.0123663
- Fairbrother, A., Purdy, J., Anderson, T., & Fell, R. (2014). Risks of neonicotinoid insecticides to honey bees. Environmental Toxicology and Chemistry, 33, 719–731. doi:10.1002/etc.2527
- Gallai, N., Salles, J.-M., Settele, J., & Vaissière, B. E. (2009). Economic valuation of the vulnerability of world agriculture confronted with pollinator decline. Ecological Economics, 68, 810–821. doi:10.1016/j.ecolecon.2008.06.014
- Hendriksma, H. P., Härtel, S., Babendreier, D., von der Ohe, W., & Steffan-Dewenter, I. (2012). Effects of multiple Bt proteins and GNA lectin on in vitro-reared honey bee larvae. Apidologie, 43, 549–560. doi:10.1007/s13592-012-0123-3
- Hendriksma, H. P., Härtel, S., & Steffan-Dewenter, I. (2011a). Testing pollen of single and stacked insect-resistant Bt-maize on in vitro reared honey bee larvae. PLoS ONE, 6(12), e28174. doi:10.1371/journal.pone.0028174.
- Hendriksma, H. P., Härtel, S., & Steffan-Dewenter, I. (2011b). Honey bee risk assessment: New approaches for in vitro larvae rearing and data analyses. Methods in Ecology and Evolution, 2, 509–517. doi:10.1111/j.2041-210X.2011.00099.x
- Huang, Z. (2009). A standardized procedure for the in vitro rearing of honey bee larvae. Retrieved from www.cdpr.ca.gov/docs/registration/reevaluation/larval_protocol.pdf
- Klein, A.-M., Vaissiere, B. E., Cane, J. H., Steffan-Dewenter, I., Cunningham, S. A., Kremen, C., & Tscharntke, T. (2007). Importance of pollinators in changing landscapes for world crops. Proceedings of the Royal Society B: Biological Sciences, 274, 303–313. doi:10.1098/rspb.2006.3721
- Krainer, S., Brodschneider, R., Vollmann, J., Crailsheim, K., & Riessberger-Gallé, U. (2016). Effect of hydroxymethylfurfural (HMF) on mortality of artificially reared honey bee larvae (Apis mellifera carnica). Ecotoxicology, 25, 320–328. doi:10.1007/s10646-015-1590-x
- Lüken, D., Janke, M., Lienau, F.-W., Gerdel, K., von der Ohe, W., & Forster, R. (2014). Optimisation of an in vitro larvae test to test the effects of plant protection products on honey bee brood (Apis mellifera L.). Journal für Verbraucherschutz und Lebensmittelsicherheit, 9, 157–166. doi:10.1007/s00003-014-0865-0
- Medrzycki, P., Sgolastra, F., Bortolotti, L., Bogo, G., Tosi, S., Padovani, E., & Sabatini, A. G. (2010). Influence of brood rearing temperature on honey bee development and susceptibility to poisoning by pesticides. Journal of Apicultural Research, 49, 52–59. doi:10.3896/IBRA.1.49.1.07
- National Agricultural Statistics Service. (2015). Honey production. US Department of Agriculture. Retrieved April 16, 2015, from http://www.nass.usda.gov
- Neumann, P., & Carreck, N. L. (2010). Honey bee colony losses. Journal of Apicultural Research, 49(1), 1–6. doi:10.3896/IBRA.1.49.1.01
- OECD. (2014). Honey bee (Apis mellifera) larval toxicity test, repeated exposure. OECD Draft Guidance Document http://www.oecd.org/chemicalsafety/testing/Draft_GD_honeybee_larval_tox_repeated_exposure_25_February_2014.pdf.
- Peng, Y.-S., Mussen, E., Fong, A., Montague, M. A., & Tyler, T. (1992). Effects of chlortetracycline of honey bee worker larvae reared in vitro. Journal of Invertebrate Pathology, 60, 127–133. doi:10.1016/0022-2011(92)90085-I
- Rembold, H., & Lackner, B. (1981). Rearing of honey bee larvae in vitro: Effect of yeast extract on queen differentiation. Journal of Apicultural Research, 20, 165–171. doi:10.1080/00218839.1981.11100492
- Riessberger-Gallé, U., Vollmann, J., Brodschneider, R., Aupinel, P., & Crailsheim, K. (2008). Improvement in the pupal development of artificially reared honey bee larvae. Apidologie, 39, 595. doi:10.1051/apido/2009006
- Sabatini, A. G., Marcazzan, G., Caboni, M. F., Bogdanov, S., & Almeida-Muradian, L Bd (2009). Quality and standardisation of royal jelly. Journal of ApiProduct and ApiMedical Science, 1, 16–21. doi:10.3896/IBRA.4.01.1.04
- Staveley, J. P., Law, S. A., Fairbrother, A., & Menzie, C. A. (2014). A causal analysis of observed declines in managed honey bees (Apis mellifera). Human and Ecological Risk Assessment: An International Journal, 20, 566–591. doi:10.1080/10807039.2013.831263
- Vanbergen, A. J., Baude, M., Biesmeijer, J. C., Britton, N. F., Brown, M. J. F., Brown, M., & Carvell, C. (2013). Threats to an ecosystem service: Pressures on pollinators. Frontiers in Ecology and the Environment, 11, 251–259. doi:10.1890/120126
- Vandenberg, J. D., & Shimanuki, H. (1987). Technique for rearing worker honey bees in the laboratory. Journal of Apicultural Research, 26, 90–97. doi:10.1080/00218839.1987.11100743
- Viuda-Martos, M., Ruiz-Navajas, Y., Fernández-López, J., & Pérez-Álvarez, J. A. (2008). Functional properties of honey, propolis, and royal jelly. Journal of Food Science, 73, R117–R124. doi:10.1111/j.1750-3841.2008.00966.x
- Wei, W.-T., Hu, Y.-Q., Zheng, H.-Q., Cao, L.-F., Hu, F.-L., & Hepburn, H. R. (2013). Geographical influences on content of 10-hydroxy-trans-2-decenoic acid in royal jelly in China. Journal of Economic Entomology, 106, 1958–1963. doi:10.1603/EC13035
- Williams, G. R., Alaux, C., Costa, C., Csáki, T., Doublet, V., Eisenhardt, D., … Brodschneider, R. (2013). Standard methods for maintaining adult Apis mellifera in cages under in vitro laboratory conditions. In V. Dietemann, J. D. Ellis & P. Neumann (Eds.), The COLOSS BEEBOOK, Volume I: Standard methods for Apis mellifera research. Journal of Apicultural Research, 52(1), 1–36. doi:10.3896/IBRA.1.52.1.04
- Zheng, H.-Q., Hu, F.-L., & Dietemann, V. (2010). Changes in composition of royal jelly harvested at different times: consequences for quality standards. Apidologie 42, 39–47. doi:10.1051/apido/2010033.
- Zhu, W., Schmehl, D. R., Mullin, C. A., & Frazier, J. L. (2014). Four common pesticides, their mixtures and a formulation solvent in the hive environment have high oral toxicity to honey bee larvae. PLoS ONE, 9(1), e77547. doi:10.1371/journal.pone.0077547