Abstract
In New Zealand, the introduced honey bee (Apis mellifera) is a valuable production animal, providing pollination services for horticultural crops and significant export volumes of honey, especially mānuka honey. Honey bees in New Zealand are free from a number of significant diseases and pests such as European foulbrood, acarine disease, small hive beetle, Israeli acute paralysis virus and tropilaelaps mites. We sought to determine the health status of honey bees in New Zealand using a longitudinal study that followed 60 beekeepers over 2.5 years, ascertaining disease and pest status in their elected study apiary and interviewing them every spring and autumn. Participant beekeepers accounted for the management of approximately 12% of the beehives registered in New Zealand. Differences in beekeeping practices were observed between the North Island and the South Island. Nosema ceranae was found almost exclusively on the North Island and did not displace Nosema apis over the course of the study. Lotmaria passim showed a reverse-phase seasonality to nosema, peaking in autumn at near 100% prevalence. The prevalence of Varroa in apiaries varied seasonally between 45.0% and 46.7% in spring and between 65.0% and 69.5% in autumn, with most infestation rates below 3 mites per 100 bees. The detection rate of symptomatic American foulbrood disease during our hive inspections was very low, between 0.00% and 0.85% hive-level prevalence dependent on the season. This study sets a foundation for understanding honey bee health in New Zealand.
Keywords:
Introduction
Honey bees (Apis mellifera) are an important economic species in New Zealand, and were introduced to the country in 1839 (Gillingham, Citation2008). They provide a substantial export of 27,000 tonnes of honey per annum and pollination for horticultural crops such as kiwifruit, avocado, apples, carrots and onions (Ministry for Primary Industries, Citation2020). Apiculture in New Zealand is a rapidly-expanding industry, with 2019 recording the highest number of registered beekeepers in New Zealand’s history; 9,828 beekeepers in total (Phiri & Rich, Citation2019). New Zealand has a relatively high density of beehives compared to other countries—there are 918,026 registered hives (Phiri & Rich, Citation2019) in a land area of 268,021 square kilometres, giving an approximate stocking rate of 3.4 hives per square kilometre, and not all of the land are viable for honey bees due to mountainous or inaccessible terrain.
New Zealand has stringent biosecurity regulations that restrict the importation of bees, bee products and used-beekeeping equipment (Biosecurity New Zealand, Citation2015). As a result, many pathogens and pests are absent from New Zealand (Ministry for Primary Industries, Citation2015); which results in increased market access for bee products of New Zealand origin (Iwasaki et al., Citation2015).
Various pathogens and pests have, however, established in New Zealand. American foulbrood (AFB, Paenibacillus larvae) and Varroa mite (Varroa destructor) are present and pose major challenges for New Zealand beekeepers (Brown et al., Citation2018). P. larvae is thought to have arrived with the initial importation of A. mellifera (Gillingham, Citation2008) and is subject to legislation that aims to eliminate it from New Zealand (Biosecurity (National American Foulbrood Pest Management Plan) Order, Citation1998). Varroa was first detected in 2000 and now affects the entire population of New Zealand honey bees with the exception of the Chatham Islands; 650 km distant from mainland New Zealand. The arrival of Varroa caused the near-total extirpation of feral colonies (Office of the Auditor-General New Zealand, Citation2002). Only managed honey bee colonies now persist.
Epidemiological surveys of New Zealand bee pathogens and pests have been previously undertaken (Todd et al., Citation2007; Mondet et al., Citation2014). A notable change in the viral ecology of A. mellifera was observed when Varroa moved into New Zealand (Mondet et al., Citation2014; Iwasaki et al., Citation2015) as has been observed in other regions (Martin et al., Citation2012; Loope et al., Citation2019). Deformed wing virus (DWV) may have been introduced by the Varroa mite, and then proliferated to become one of the predominant viruses affecting New Zealand honey bees (Mondet et al., Citation2014). Other endemic viruses include: Kashmir bee virus (KBV), Chronic bee paralysis virus (CBPV), Black queen cell virus (BQCV), Sacbrood virus (SBV), and also the fungus chalkbrood Ascosphaera apis. Notable organisms that have not been detected in New Zealand honey bees are European foulbrood Melissococcus plutonius (Pharo, Citation2006), Israeli acute paralysis virus (McFadden et al., Citation2014), tracheal mite Acarapis woodi, and small hive beetle Aethina tumida, amongst others (New Zealand Government, Citation2016; Biosecurity New Zealand, Citation2020).
A survey of New Zealand beekeepers that documents winter colony loss has been undertaken every year from 2015 (Brown et al., Citation2018), in accordance with international protocols (van der Zee et al., Citation2013; Jacques et al., Citation2017). It is a voluntary survey that relies on beekeepers reporting factors they consider having caused colony loss. Surveillance for exotic pests and pathogens is also conducted every year (Phiri & Rich, Citation2019). However, it has been nearly a decade since Mondet et al. (Citation2014) conducted their cross-sectional study of endemic disease in New Zealand honey bees, which only focussed on Varroa and viruses and did not record details on colony losses, productivity, management, nosema disease or American foulbrood. There have been no longitudinal studies of honey bee health in New Zealand. This type of work has been shown to be of importance in other countries because it provides inferences on the relationship between pathogens, pests and their impact on colony health (Genersch et al., Citation2010; Steinhauer et al., Citation2014; Castillo et al., Citation2017).
We conducted a longitudinal study over two-and-a-half years involving 60 participant beekeepers from all over New Zealand; each participant allowed us access to an elected study apiary. We sought to describe the apicultural management practices used in New Zealand, and determine the baseline prevalence and seasonality of pathogens and pests of New Zealand honey bees.
Materials and methods
Study participants
Sixty beekeepers throughout New Zealand were recruited into the study, and each beekeeper nominated an apiary for inclusion in the study. An apiary was defined as eight or more colonies in a single location, under the management of a beekeeper or beekeeping enterprise. The proportion of beekeepers enrolled per region reflected the density of registered beekeepers across New Zealand i.e. more beekeepers were enrolled from regions that had a higher proportion of the national number of beekeepers. Nine apicultural inspectors were recruited to perform interviews, hive inspections and sample collection. Inspectors provided local contact with potential participants because of the inspectors’ knowledge of beekeeping operations within their regions. This approach was taken to ensure sustained participation rates were attained over the course of the study. The consent we obtained from the beekeepers required that their identities and exact apiary locations are not disclosed.
The study began in September 2016 (spring in the southern hemisphere). Beekeepers were interviewed, and their apiaries inspected and sampled every six months—specifically taking place over five rounds, those being spring 2016, autumn 2017, spring 2017, autumn 2018 and spring 2018. Beekeepers were given a report about the results from their apiaries after each round—this incentivised their participation. Beekeepers could potentially have changed their management practices in response to the interim reports they received, so they were asked at the beginning of the study to make sure they continued with their usual beekeeping practices e.g. hive movements, pollination, re-queening, colony splitting, supplementary feeding, Varroa treatments; and to avoid being influenced by the study results. One participant left the study, in round 5 (spring 2018), due to ceasing beekeeping operations. One participant could not provide an interview or access to their apiary in round 4 (autumn 2018), and another participant could not provide for an interview or access to their apiary in round 5 (spring 2018).
Apiary inspections
At each six-monthly apiary visit, the inspectors would randomly select eight hives for health inspection. Inspectors would remove and visually inspect every frame from the brood box(es) of each colony. Inspectors visually checked and recorded the presence of American foulbrood, deformed wings on adult bees, or the presence of Varroa mites on adult bees. Inspectors also visually checked for the presence of the exotic pests and diseases, specifically European foulbrood and small hive beetle, which are not known to be present in New Zealand. Other observations were recorded by the inspectors but are not included in this paper.
Interviews with beekeepers
Beekeepers were interviewed every round during the hive inspections to obtain information on apicultural management practices, productivity and disease. The questionnaire was developed in consultation with beekeepers. It was similar in design to the method outlined by the Bee Informed Partnership (Steinhauer et al., Citation2014) but was specifically adapted for a New Zealand beekeeping context. The questionnaire included questions on: beekeeping experience (years), total colony number, staff employment, apiary purpose, local floral resources, Varroa-monitoring and management and supplementary feeding. Inspectors also asked the beekeeper if they thought there were too many hives close to their apiary site, that being described as overcrowding. Interviews were conducted onsite using portable electronic tablets. Data from apiary inspections and beekeeper interviews were entered into a survey created using the Survey123 for ArcGIS application (https://survey123.arcgis.com/).
Collection of adult honey bees for pathogen and Varroa detection
Adult honey bees were collected from the same eight hives that underwent hive inspection at each apiary. Approximately 100 adult bees (a 60 mL volume) were taken from each of the eight hives and then combined to form a composite sample. Live bees were shipped to the laboratory, where the bees were euthanised using dry ice, stored at −80 °C and later subjected to qPCR testing. An identical composite sample was preserved in 150 mL of 70% ethanol, and used to assess Varroa mite infestation.
Quantification of Varroa by microscopy
Bees preserved in ethanol were emptied into a 1 L strainer-container within its detachable basket, and submerged in 500 mL of warm water. The container was shaken on a Ratek EOM5 Medium Orbital Shaker (Ratek, Melbourne, Australia) at 175 rpm for 20 min to allow external mites to be dislodged. A strainer-insert containing washed bees was removed, and the remaining fluid was poured through 40 μm filter stainless steel mesh. The mesh, containing mites and other occlusions, was then washed with 90% ethanol. Varroa mites were counted under a dissecting microscope, as previously described (Fan et al., Citation2018). Varroa infestation rate was expressed as the number of mites per 100 bees. Apiaries were recorded as positive for Varroa if a single mite was detected during the test. Varroa infestation was categorised as high or low, based on a threshold of 3 mites per 100 bees; noting that the Varroa were counted from a composite sample of eight hives. This arbitrary threshold was selected as it reflects a general estimate in the literature at which damage to a colony could occur (Currie & Gatien, Citation2006; Goodwin & Van Eaton, Citation2001; Lee et al., Citation2010).
Detection and quantification of pathogens by qPCR
Fifty frozen worker bees were placed into 10 mL of lysis buffer [5.25 M guanidine thiocyanate, 50 mM Tris, 20 mM EDTA, 1.3% Triton-X 100, 1% antifoam B emulsion (Sigma-Alrich, St Louis MO)] and subjected to maceration using a Geno/Grinder® (SPEX® SamplePrep, Metuchen NJ). Maceration was carried out at 1500 rpm for three minutes inside a 50 mL conical tube (Labcon, Petaluma CA) containing four 9.5 mm stainless steel ball bearings. The sample was then strained through the mesh of a Bioreba extraction bag (Bioreba AG, Reinach, Switzerland) to remove exoskeleton fragments and 100 µL of the lysate was used directly for RNA extraction. DNA extraction required a further processing step to ensure complete disruption of endospores and microsporidians, where 200 µL of the lysate was subjected to disruption by bead-beating in a MagNA Lyser instrument (Roche, Basel, Switzerland) at 6,500 rpm for 60 seconds.
RNA extraction was performed using the Qiagen RNeasy kit (Qiagen, Hilden, Germany), including the following modifications: 100 µL of lysate was combined with 600 µL of RLT buffer and then subjected to centrifugation through a QIAShredder column (Qiagen, Hilden, Germany) for 2 minutes at 9,000 x g, 700 µL of 70% ethanol was added to the supernatant, and then 700 µL of the combined volume was carried forward into the RNA extraction as per the manufacturer’s instructions. RNA was eluted into 50 µL of UltraPure™ DNase/RNase-Free Distilled Water (Thermo Fisher Scientific, Waltham MA) and diluted 1:5 for use as a template RNA in qPCR.
DNA was extracted using the Qiagen DNeasy Blood & Tissue Kit (Qiagen, Hilden, Germany) with some modifications, as follows: 20 µL of proteinase K was added to 200 µL of lysate and then incubated at 56 °C for two hours. The lysate was subjected to centrifugation through a QIAShredder column for 2 minutes at 9,000 x g, and then processed through the kit as per the manufacturer’s instructions. The DNA was eluted into 100 µL of RNA-free water and used at neat concentration for qPCR.qPCR assays were performed using methods previously described, with modifications described in Supplementary material, Table 1: Black queen cell virus (BQCV; Chantawannakul et al., Citation2006), Chronic bee paralysis virus (CBPV; Blanchard et al., Citation2007), Deformed wing virus (DWV; Chantawannakul et al., Citation2006), Kashmir bee virus (KBV; vanEngelsdorp et al., Citation2009), Israeli acute paralysis virus (IAPV; Locke et al., Citation2012), European foulbrood (Mellissococcus plutonius; Budge et al., Citation2010; Roetschi et al., Citation2008), Nosema apis (Bourgeois et al., Citation2010), Nosema ceranae (Chen et al., Citation2008) and the trypanosomatids; Lotmaria passim and Crithidia mellificae (Runckel et al., Citation2014). The qPCR assay that was used to detect trypanosomatids detects both Lotmaria passim and Crithidia mellificae but does not distinguish between them (Runckel et al., Citation2014). A quantitative PCR for P. larvae was performed using a commercially-available qPCR assay: the AFBduo qPCR kit (dnature diagnostics & research Ltd, Gisborne, New Zealand) where a positive detection of P. larvae DNA was only recorded if both gene targets in the kit showed qPCR amplification. Two additional honey bee genes were tested using qPCR, to provide an endogenous control, and to normalise the qPCR results between samples, as previously described (Chen et al., Citation2005; Lourenço et al., Citation2008) with modifications (). The RP49 gene was used as a control qPCR assay (Lourenço et al., Citation2008) when testing for the RNA viruses BQCV, CPBV, DWV and KBV. The β-actin gene provided a control qPCR assay (Chen et al., Citation2005) when testing for nosemas, trypanosomatids, and P. larvae.
Table 1. Purpose and characteristics of the study apiaries, as described during the first sampling round in spring 2016.
Statistics
The 95% confidence intervals for prevalence values were calculated using the Wilson Score confidence interval method implemented by the R package epiR (epiR: Tools for the Analysis of Epidemiological Data. R package version 2.0.17. https://CRAN.R-project.org/package=epiR, accessed 19 March 2021). The two-proportion z-test was used to compare the observed proportions of apiary characteristics between the North Island and South Island.
Results
Beekeeping practices in New Zealand
The sixty participant beekeepers were representative of a diverse cross-section of apiculture with their experience ranging from 1 to 62 years. At enrolment in spring 2016, the median size of the study apiaries was 20 colonies, but ranged from a minimum of eight colonies to a maximum of 400 colonies. However, participant beekeepers managed other apiaries not enrolled in the study, as part of their normal business enterprise. In subsequent seasons, there were eight instances (2.6% of apiary visits) where study apiaries had less than eight colonies present at the time of inspection, but their data were still included in our analyses. The enrolled beekeepers managed between 12.2% and 12.9% of all registered colonies in New Zealand (Supplementary material, Table 2). The median number of colonies operated by a beekeeper over the study period was 940 (lower quartile 450, upper quartile 2000), although a few larger operations had over 10,000 colonies. Forty study apiaries were located in the North Island and 20 in the South Island (), spanning 12 degrees of latitude from 34°S to 46°S – the country being approximately 1,600 kilometres in length. The participant’s apiary locations generally reflected the density of colonies throughout the country (https://apinz.org.nz/wp-content/uploads/2018/06/heatmap-of-New-Zealand-apiaries-March-2016.pdf accessed 4 March 2021). Five apiaries changed location during the study but this did not affect the geographic distribution shown in .
Figure 1. Schematic figure showing the geographic distribution of study apiaries across New Zealand; aggregated site data is presented to protect the privacy of the participant beekeepers.
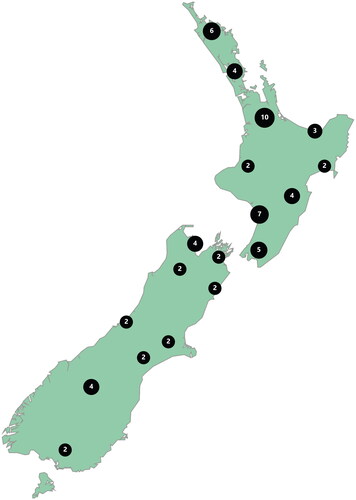
Table 2. Supplementary feeding activity reported by participant beekeepers across the five sampling rounds, from 2016 to 2018.
The most common activity for a study apiary was the production of honey or associated bee products (viz. royal jelly, bee venom, pollen, propolis.; ). Nearly, half of the apiaries were for overwintering—some may have been used solely for this purpose and thus providing an explanation why bee products were not produced at the apiary (16.7% of study apiaries did not produce bee products; ). In New Zealand, an overwintering apiary is a site that is easily accessible for beekeepers and has sufficient floral resources to forage on during winter. It should be noted that some apiaries had multiple purposes.
There were indications of differences in apiary characteristics between the North and South Islands, although none approached statistical significance (). The South Island had a higher proportion of apiaries involved in live bee production than the North Island (). Overcrowding was also perceived to be less of a problem in the South Island ().
The replacement of brood frames appears to be commonplace in New Zealand—the majority of beekeepers indicated that they aim to replace ≥10% of brood frames annually (), but a proportion (33.5%) indicated they did not have a brood frame replacement policy.
Figure 2. The brood frame replacement policies of participant beekeepers, as reported at the beginning of the study.
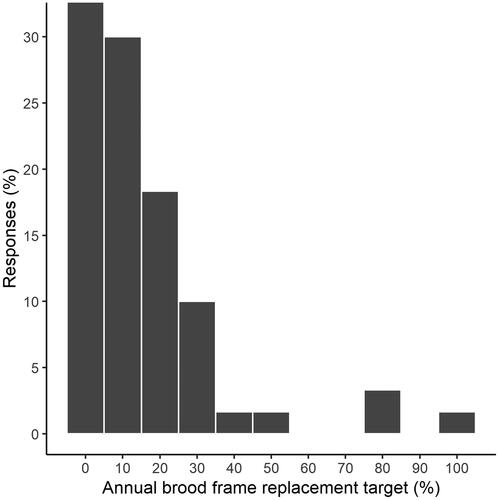
A diversity of pollen and nectar sources were recognised by beekeepers as being close to their apiary. Clover (e.g. Trifolium repens), willow (Salix sp.), general pasture, gorse (Ulex europaeus) and mānuka (Leptospermum scoparium) were cited by beekeepers as the top-five most-common floral sources in proximity (). Notable differences between the North and South Islands are to be expected, and were confirmed. Mānuka was not mentioned in the top-fifteen floral sources for the South Island apiaries, but the related myrtle species of kānuka (Kunzea ericoides) was.
Figure 3. The fifteen most-common floral sources perceived by the participant beekeepers to be in proximity to their apiary. (A) All participants, nationwide across New Zealand. (B) North Island participants only. (C) South Island participants only.
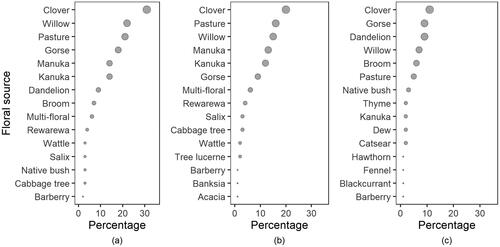
Supplementary feeding was commonplace during the study; reported as 76.7–88.3% of the interview responses across the sampling rounds (). The majority of supplementary feeding was with sugar syrup, and this did not vary between spring and autumn (). Less pollen substitute was used in autumn (16.7–25.4%) as compared to spring (50.0–55.2%; ).
Seasonal prevalence of pathogens and pests of New Zealand honey bees
IAPV and Melissococcus plutonius were not detected in any of the samples. This result was expected given they are considered to be exotic organisms to New Zealand (Jamaludin et al., Citation2002; McFadden et al., Citation2014; Biosecurity New Zealand, Citation2020).
Known endemic viruses (Mondet et al., Citation2014) were found to be common amongst New Zealand honey bees (). Both BQCV and DWV predominate, approaching near 100% prevalence in the apiaries sampled. N. apis and N. ceranae were also common. N. ceranae was almost exclusively found in the North Island, it was only observed in the South Island on two occasions over the course of the study (data not shown).
Figure 4. Seasonal prevalence of viruses in New Zealand honey bees during 2016–2018. Error bars indicate 95% confidence intervals. BQCV: Black queen cell virus. CBPV: Chronic bee paralysis virus. DWV: Deformed wing virus. KBV: Kashmir bee virus. Deformed wings: visual sighting of deformed wings during hive inspection.
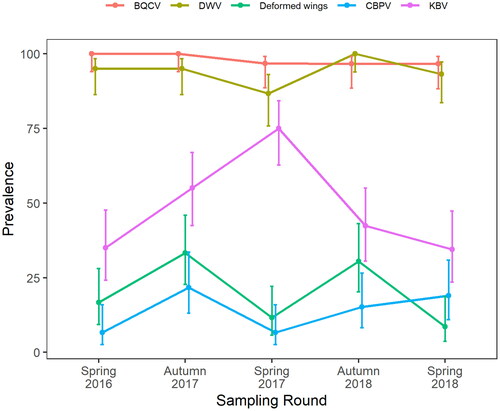
Viruses showed no overt seasonality (), with the exception of a slight increase in prevalence in autumn for DWV. KBV showed a marked increase in prevalence in spring 2017 (). CBPV persisted at a mean prevalence below 20% (). Inspectors observed more deformed wings in autumn than spring ().
N. apis and N. ceranae had an apparent seasonality (). Peak occurrence of nosema (both species) was in the spring. N. apis and N. ceranae were often present within the same apiary, as evidenced where 149/298 (50%) of honey bee samples collected over the course of the study were positive for both. N. ceranae did not displace N. apis during the study.
Figure 5. Seasonal prevalence of pathogens and pests in New Zealand honey bees during 2016–2018 for: (A) Nosema ceranae, N. apis and trypanosomatids (viz. Lotmaria passim), as detected using qPCR; and (B) Varroa destructor detected by either laboratory alcohol-wash, or by visual sighting of Varroa mites on live adult bees within a hive. Error bars indicate 95% confidence intervals.
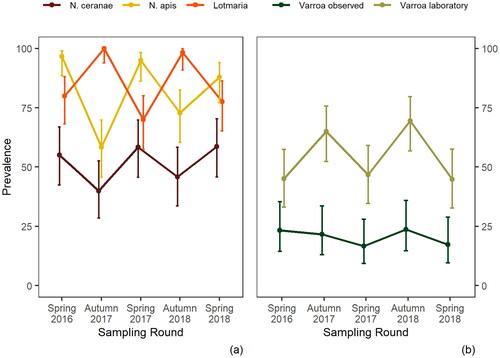
Trypanosomatid prevalence approached nearly 100% every autumn (). There is no evidence for the presence of Crithidia mellificae in New Zealand (Waters, Citation2018) so all detections were considered to be of Lotmaria passim.
Varroa mites are highly prevalent in New Zealand apiaries and were detected in 65.0–69.5% of study apiaries in the autumn, and 45.0–46.7% of study apiaries in the spring (). The autumnal preponderance of Varroa is expected, as mite numbers build up over the spring and summer when substantial brood-rearing occurs. In contrast to laboratory inspection, visual inspection revealed Varroa occurrence in only 16.7–23.7% of apiaries across the seasons (). For the majority of apiary visits over the duration of the study, the Varroa counts were under the 3 mites per 100 bees damage-threshold ().
Figure 6. Varroa infestation rates in apiaries, by season. Dashed line indicates the threshold of 3 mites per 100 bees. Lower graph shows statistics for apiaries with less than five mites per 100 bees. Upper graph shows all data.
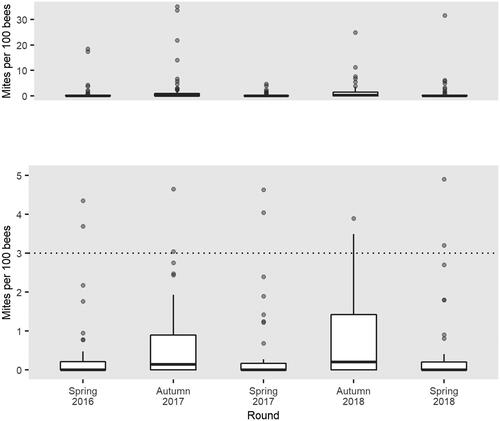
Both synthetic and organic miticide treatments were commonly used by beekeepers to treat Varroa infestation (). Between 56.7% and 72.9% of participant beekeepers reported that they had been monitoring for Varroa infestation, dependent upon sampling round (). Visual screening was the most common form of Varroa monitoring.
Table 3. Varroa monitoring and treatment reported by participant beekeepers across the five sampling rounds, from 2016 to 2018.
The most-favoured treatment methods were amitraz and flumethrin (), both synthetic miticides. Less utilised were tau-fluvalinate, and the organic miticides of oxalic acid and formic acid ().
The hive-level prevalence of American foulbrood in the study apiaries at the time of inspection ranged between 0.00% and 0.85%, dependent upon season (). The apiary-level prevalence of symptomatic American foulbrood at the time of inspection varied from 0.0% to 5.0% across the seasons (). Over the course of the study there were seven of the 60 apiaries that had symptomatic AFB present during an inspection: two of these apiaries had symptomatic AFB in 2/5 seasons, five of the apiaires had symptomatic AFB in 1/5 seasons, and 53 apiaries never had symptomatic AFB present during an inspection.
Table 4. American foulbrood (AFB) in study apiaries.
A formative comparison of a qPCR test for P. larvae was made, comparing it to apiary-level symptomatic American foulbrood (). The number of American foulbrood symptomatic-apiaries was not large enough to be informative on the diagnostic parameters of sensitivity or specificity for the qPCR test.
Table 5. Frequency of qPCR assay detection of P. larvae DNA in composite samples of adult bees taken from apiaries, compared the frequency of symptomatic American foulbrood at apiaries during hive inspection.
Discussion
The beekeepers and apiaries in this study can be considered to be a cross-section of apicultural practices in New Zealand. Study sites spanned 12 degrees of latitude, from 34°S to 46°S, and apiaries were engaged in a variety of purposes including pollination, over-wintering, bee products, queen production, and the sale of live bees. Previous work on honey bee health in New Zealand has been either the diagnostic detection of pathogens and pests in hives (Mondet et al., Citation2014), or voluntary questionnaire surveys about colony loss (Brown et al., Citation2018). To the best of our knowledge, there has been no previous longitudinal study of New Zealand honey bee health, although such studies do take place in other countries (Castillo et al., Citation2017; Steinhauer et al., Citation2014).
Longitudinal studies can provide better inferences on factors affecting honey bee health compared to cross-sectional studies because they account for seasonal variation and can reveal trends over time. Participant beekeepers could have altered their management practices during the study, because we provided them with reports at the end of each season. However, the issuance of reports each season kept the participation rate very high as only one participant withdrew from the study during the final season (spring 2018, round 5), and two participants could not provide access to their apiaries for a single season (see Methods). The study cohort was largely reflective of the apicultural practices reported in the New Zealand Colony Loss Surveys (2015–2018), where 40% of New Zealand beekeepers respond to that annual survey (Brown & Robertson, Citation2018). However, it should be noted that there is a potential for recruitment bias in our study as only beekeepers who volunteered could be included, and inspectors were also involved in identifying willing participants.
There were seasonal and geographic differences in beekeeping practices and disease prevalence, although they did not achieve statistical significance. The South Island participants were less inclined to mention overcrowding as a problem, and they were more involved in live bee production. South Island participant beekeepers did not engage in pollen supplementation in spring, whereas such practice was commonplace for the North Island participants. Furthermore, the South Island beekeepers did not mention mānuka in the top fifteen floral sources near their apiary, whereas mānuka was the fourth most-commonly listed floral source by North Island beekeepers. None of these results met statistical thresholds for significance, but they are in strong agreement with the regional differences that have been reported during the New Zealand Colony Loss survey (Brown & Robertson, Citation2018). Much of the recent growth in New Zealand apiculture can be attributed to the demand for mānuka honey, because of its documented antimicrobial effects (Girma et al., Citation2019; Johnston et al., Citation2018) that have driven international demand (Van Eaton, Citation2014). Exports of mānuka honey are valued at over $425 million NZD (Ministry for Primary Industries, Citation2020). Mean annual temperatures in the South Island can be 6 °C lower than those of the North Island and there are many climatic and biotic (e.g. floral) differences between the islands (Goldberg et al., Citation2008; Singers & Rogers, Citation2014; NIWA, Citation2020). Mānuka nectar flows are reported as being less common in the lower South Island, overcrowding is cited as a cause of colony loss in the upper North Island, and protein supplementation levels between the North and South Islands have been shown to be different (81.4% North Island, 59.2% South Island; Brown & Robertson, Citation2018). The proportion of apiaries engaged in pollination in the present study (13.3%) is less than that reported in the New Zealand Colony Loss Survey (35.3%), but our longitudinal study may have a selection bias towards apiaries with more stability i.e. overwintering sites, compared to the colony loss survey that examines annual winter-losses for beekeepers from all their apiaries, including transient apiaries such as pollination sites.
The annual replacement of frames (comb) within a hive has been shown to reduce pesticide levels within a hive (Lodesani et al., Citation2008; Wu et al., Citation2011, Citation2012) and may reduce nosema disease (Fries, Citation1988). Beekeepers regard frame replacement as a way to decrease disease burden and increase productivity (Fries, Citation1988; de Guzman et al., Citation2019; Leahy, Citation2019). The majority of participants in this study sought to replace at least 10% of their brood frames each year, and some sought to replace 100%. Similar findings are presented in the New Zealand Colony Loss Survey where commercial beekeepers with more than 250 colonies report an average for brood-frame replacement of 16.4% annually (Brown & Robertson, Citation2018). There is now the potential for multivariable analyses on the dataset presented in this study to evaluate the association of brood frame replacement policies with disease, productivity and other aspects of apicultural practice.
Varroa destructor was first detected in New Zealand in 2000 (Iwasaki et al., Citation2015). In our study, 45.0–46.7% of apiaries had Varroa mite infestations in the spring, but the prevalence increased in autumn to 65.0–69.5% (dependent on sampling round)—a seasonal characteristic of Varroa reproduction, as the mite benefits from the increased brood-rearing over spring and summer (Rosenkranz et al., Citation2010; Giacobino et al., Citation2016; Traynor et al., Citation2016). The apiary-level prevalence of Varroa in our study is consistent with that reported for other countries such as the USA (Traynor et al., Citation2016) and Austria (Morawetz et al., Citation2019) although apiary-level Varroa prevalence can vary greatly within countries e.g. within Mongolia, regional prevalence varied from 4% to 100% between apiaries in summer due to climatic, geospatial and treatment factors (Tsevegmid et al., Citation2016). Prevalence of Varroa in New Zealand honey bee colonies had been reported to be 85–100% during autumn, but that study measured Varroa before seasonal treatments were applied to colonies, and only in 11 apiaries (Mondet et al., Citation2014). Our sampling occurred irrespective of the state of Varroa treatment at the study apiaries, because it was not possible to time our sampling events with the variety of treatment regimes that beekeepers used, particulary given the number of beekeepers involved, and their widespread geographic distribution.
Many beekeepers reported that they did not monitor for Varroa, this suggests that beekeepers may show a preference for ‘treatment by the calendar’, where they assume Varroa is present and undertake miticide treatments outside of the honey flow. Approximately one-third of the participant beekeepers used the Varroa-monitoring methods that have been recommended as best-practice: alcohol-wash, sugar-shake or uncapping drone brood (Dietemann et al., Citation2013). Monitoring of Varroa infestation levels is recommended to ensure that applied treatment regimens are effective (Goodwin & Van Eaton, Citation2001). Consistent monitoring may be useful in comparing alternative treatments, or the timing of application (Goodwin & Van Eaton, Citation2001). Visual monitoring for phoretic Varroa mites on adult bees was the most-common method of Varroa monitoring reported by beekeepers in our study. However, visual inspection for Varroa mites on live adult bees may have only speculative value. Ramsey et al. (Citation2019) report, when surveying four colonies, that 95.2% of Varroa mites on adult bees were located on the ventral surface of the metasoma, underneath the abdominal plates, and therefore concealed from view. We asked our trained inspectors to note the presence of Varroa mites on adult bees, and we compared their reports to the laboratory measurement using the alcohol-wash method. We found a gross difference in the prevalence reported between the two methods, where inspector-reported prevalence was 30% lower than the laboratory-reported prevalence. Beekeepers should consider that although visual detection of Varroa mites on adult bees is useful, it is not a reliable method for determining if Varroa is present.
Most beekeepers in the study had Varroa infestation rates in their apiaries well under control, with the majority keeping infestation rates below 3 mites per 100 bees. Many factors are known to affect the threshold at which Varroa mite infestation becomes grievously injurious to the colony or has an economic impact that would compel treatment e.g. season, location, colony population size, nutrition (Goodwin & Van Eaton, Citation2001; Currie & Gatien, Citation2006; Lee et al., Citation2010). Some colonies may be able to tolerate higher Varroa mite infestations before sustaining damage. Consequently, there is no scientific consensus as to the Varroa mite infestation level that warrants treatment or intervention. In our study, an arbitrary infestation rate of 3 mites per 100 bees (3% infestation) was selected to distinguish between apiary visits where there were high or low Varroa-infestations—although the rate of 3 mites per 100 bees is in general agreement with published damage-thresholds for Varroa (Goodwin & Van Eaton, Citation2001; Currie & Gatien, Citation2006; Lee et al., Citation2010).
Nosema apis and N. ceranae co-infections were common; the latter not documented to have occurred in New Zealand until 2010 (Frazer et al., Citation2015; Murray & Lester, Citation2015). In our study, N. ceranae was found almost exclusively in the North Island, with only two detections in the South Island being observed over the study period. There has been some conjecture in the literature that N. ceranae may displace N. apis (Klee et al., Citation2007; Fries, Citation2010; Yoshiyama & Kimura, Citation2011) but this hypothesis does not appear to be supported in our study. Instead, we observed similar results to a 12-year longitudinal study in Germany where both nosema species were found to co-exist within 230 managed colonies (Gisder et al., Citation2017). We also observed an apparent seasonal effect for both nosema species, both having higher prevalence in the spring, as has been reported in other temperate countries (Traver et al., Citation2012; Gisder et al., Citation2017; Ondrus, Citation2017). The preponderance of N. ceranae in the North Island (and to an extent, that of N. apis) may be explained, in part by the warmer temperatures experienced in the northern latitudes of New Zealand. Warmer climate may account for increased infectivity or spread of these organisms (Gisder et al., Citation2010). It is not yet clear what other factors may have driven the entrenchment of N. ceranae in the North Island, and its near-absence from the South Island. Certainly, Varroa spread to all corners of New Zealand (with the exclusion of the Chatham Islands and Stewart Island) within approximately ten years. The movement of hives across Cook Strait (separating North and South Islands) does occur frequently (pers. com. Darren Bainbridge, My Apiary), so it appears that N. ceranae may not take hold in the south.
Trypanosomatid parasites were present at very high prevalence, peaking at 100% in autumn 2017. The qPCR used in the study cannot distinguish between Lotmaria passim and Crithidia mellificae, but given that C. mellificae has not been detected in New Zealand (Waters, Citation2018) it can be reasonably assumed that the detections in this study are therefore of L. passim. Lotmaria passim was only identified as a species in 2015 (Schwarz et al., Citation2015), and so studies before 2015 that use the term C. mellificae could be refering to either L. passim or C. mellificae. Seasonality in L. passim infestation has been observed previously, where higher trypanosomatid parasite levels occurred in bees in winter (Runckel et al., Citation2011; Vejnovic et al., Citation2018), and higher apiary-level prevalence was in winter (D’Alvise et al., Citation2019). Moreover, infection loads of N. ceranae and L. passim in bees have been shown to be positively-correlated in some studies (Runckel et al., Citation2011; Vejnovic et al., Citation2018) but not in others (Tritschler et al., Citation2017; Castelli et al., Citation2019). Ravoet et al. (Citation2013) also reported that in summer, the detection of C. mellificae (likely to be L. passim) contributed to increased winter colony losses, and co-infection with N. ceranae led to greater winter mortalities because of a synergistic effect. We directly observed higher apiary-level prevalence of trypanosomatid parasites (viz. L. passim) in apiaries throughout New Zealand during autumn. This is a reverse-phase seasonality to that observed for the nosema species in our study, which have peak apiary-level prevalence in spring. Despite the very high prevalence of L. passim recorded in our study (nearly 100% in autumn) and the high prevalence of N. ceranae (76.3% in the North Island), New Zealand still reported, and continues to report, relatively low rates of winter colony losses (approx. 10%) compared to other countries (Steinhauer et al., Citation2014; Chauzat et al., Citation2016; Brown & Robertson, Citation2018). The pathogenicity of L. passim, and its interaction with N. ceranae should be the focus of further investigation.
The prevalence of viral agents in New Zealand bees has remained largely unchanged since the last comprehensive survey completed by Mondet et al. (Citation2014)—DWV and BQCV remain highly prevalent, near 100%. Some care must be exercised when comparing our results to that of Mondet et al. (Citation2014) because their study used decapitated bees, whereas we used whole intact bees. Mondet et al. (Citation2014) also excluded 44% of colonies in their study, based on those that had high or low Varroa infestations, whereas we included all colonies sampled, in our viral analysis. The viral prevalence data we report is similar to that in other countries where Varroa is present (Amiri et al., Citation2015), with the exception of IAPV that we did not detect, and has been shown to be absent from New Zealand (McFadden et al., Citation2014). No explanation can be found as to the apparent upsurge in prevalence of KBV in spring 2017. A multivariable analysis of the dataset may reveal possible explanations. No strong seasonal effect was evident for viruses, with suggestive minor peaks in prevalence for DWV in autumn. However, strong seasonal components to viral prevalence, particularly DWV, IAPV and ABPV, have been found in other countries (Tentcheva et al., Citation2004; Traynor et al., Citation2016). The prevalence of deformed wings observed during hive inspections did show a seasonal trend, with more deformed wings being observed in the autumn (). DWV certainly causes deformed wings in honey bees, with this symptom requiring the presence of Varroa mites in order to manifest (de Miranda & Genersch, Citation2010). In our study, it appears that DWV infections are likely to be maintained in most apiaires throughout the year, but the increase in Varroa mite infestations in autumn then triggers the overt symptom of deformed wings in adult bees in autumn.
American foulbrood is controlled by legislation in New Zealand that requires beekeepers to undertake regular hive inspections, and destroy infected colonies within seven days of being discovered. We report the prevalence of American foulbrood at the hive-level in our study so that it can be compared with the prevalence reported annually by the agency that controls American foulbrood in New Zealand (King, Citation2020). Despite the frequency at which apiaries were affected, beekeepers were managing to keep symptomatic infections at a very low level of 0.0–0.9%, similar to that reported previously (Brown & Robertson, Citation2018; King, Citation2020). The seasonal apiary-level prevalence of symptomatic AFB at the time of inspection (that ranged from 0.0%-5.0%) is similar to that observed in other studies, where Morawetz et al. (Citation2019) report an apiary-level prevalence of symptomatic AFB of <1% in 189 apiaries in Austria, and Chauzat et al. (Citation2016) report an apiary-level prevalence of symptomatic AFB of between 1.88%-2.36% in >3000 apiaries across 17 European member states (Chauzat et al., Citation2016). It should also be noted that antibiotic use is strictly prohibited in beehives in New Zealand, and antibiotic-use is known to mask the presence of symptomatic AFB (Locke et al., Citation2019), which may therefore affect prevalence estimates of symptomatic AFB in other countries where antibiotic use is permitted.
Longitudinal studies are valuable for establishing baseline data on infection rates, and for examining the interaction of pathogens and pests with other factors such as climate, hive density, beekeeping practices, colony loss and productivity. Longitudinal studies are useful for examining complex and confounding relationships between study variables in a way that is not possible with cross-sectional studies. Taking multiple measurements on the same individuals or groups of individuals increases the confidence in the results obtained, and provides robust evidence that beekeepers can then apply to their own apiaries.
This study provides some contrasting examples of disease seasonality compared to that observed in other countries, as well as providing the most in-depth description of beekeeping practices and pathogen/pest prevalence in New Zealand. The collection of stored honey bees and metadata will likely furnish future epidemiological, biosecurity and genetic studies of international interest.
Supplementary Tables
Download MS Word (24.2 KB)Acknowledgements
We would like to recognise the time, professionalism and expertise of the apicultural inspectors who contributed to this research: Dan Lambert, Kim Kneijber, Trevor Bryant, John Maynard, Stephen Black, Allan Richards, Norbert Klose, Phil Sutton, and Grant Hayes. We are also grateful for the technical advice and guidance provided by John Mackay (dnature, New Zealand), Peter Ferris, Barry Foster, John McLean, Nigel Press, Frank Lindsay and Mary-Ann Lindsay. Our sincere thanks to the National Bee Diagnostic Centre (Alberta, Canada) and the Bee Informed Partnership (Maryland, USA) for their guidance and advice. Our thanks also to our colleagues at the Ministry for Primary Industries Animal Health Laboratory and Plant Health and Environment Laboratory who have provided technical support, and our thanks to our LIMS and Information Technology colleagues also. Thank you to Evan Brenton-Rule for his comments on the manuscript. This project was funded by the New Zealand Ministry for Primary Industries Operational Research Programme. Finally, we would like to express our gratitude to the beekeepers who opened up their apiaries and beehives, and participated in this research.
Disclosure Statement
No potential conflict of interest was reported by the author(s).
Supplementary material
Supplementary Tables are available via the ‘Supplementary’ tab on the article’s online page (http://dx.doi.org/10.1080/00218839.2021.1936422).
Additional information
Funding
References
- Amiri, E., Meixner, M., Nielsen, S. L., & Kryger, P. (2015). Four categories of viral infection describe the health status of honey bee colonies. PLoS ONE, 10(10), e0140272. https://doi.org/https://doi.org/10.1371/journal.pone.0140272
- Biosecurity (National American Foulbrood Pest Management Plan) order (1998). New Zealand. http://www.legislation.govt.nz/regulation/public/1998/0260/latest/whole.html1 (accessed 27 March 2020)
- Biosecurity New Zealand. (2020). Absence of specified animal diseases from New Zealand. 1 September 2020. Retrieved September 15, 2020, from https://www.biosecurity.govt.nz/dmsdocument/10466-Statement-Absence-of-specified-diseases-from-New-Zealand
- Blanchard, P., Ribière, M., Celle, O., Lallemand, P., Schurr, F., Olivier, V., Iscache, A. L., & Faucon, J. P. (2007). Evaluation of a real-time two-step RT-PCR assay for quantitation of chronic bee paralysis virus (CBPV) genome in experimentally-infected bee tissues and in life stages of a symptomatic colony. Journal of Virological Methods, 141(1), 7–13. https://doi.org/https://doi.org/10.1016/j.jviromet.2006.11.021
- Bourgeois, A. L., Rinderer, T. E., Beaman, L. D., & Danka, R. G. (2010). Genetic detection and quantification of Nosema apis and N. ceranae in the honey bee. Journal of Invertebrate Pathology, 103(1), 53–58. https://doi.org/https://doi.org/10.1016/j.jip.2009.10.009
- Brown, P., Newstrom-Lloyd, L. E., Foster, B. J., Badger, P. H., & McLean, J. A. (2018). Winter 2016 honey bee colony losses in New Zealand. Journal of Apicultural Research, 57(2), 278–291. https://doi.org/https://doi.org/10.1080/00218839.2018.1430980
- Brown, P., Robertson, T. (2018). Report on the 2018 New Zealand Colony Loss Survey (MPI Technical Paper No: 2019/02). Retrieved March 27, 2020, from https://www.mpi.govt.nz/dmsdocument/33663-2018-bee-colony-loss-survey-report
- Budge, G. E., Barrett, B., Jones, B., Pietravalle, S., Marris, G., Chantawannakul, P., Thwaites, R., Hall, J., Cuthbertson, A. G. S., & Brown, M. A. (2010). The occurrence of Melissococcus plutonius in healthy colonies of Apis mellifera and the efficacy of European foulbrood control measures. Journal of Invertebrate Pathology, 105(2), 164–170. https://doi.org/https://doi.org/10.1016/j.jip.2010.06.004
- Castelli, L., Branchiccela, B., Invernizzi, C., Tomasco, I., Basualdo, M., Rodriguez, M., Zunino, P., & Antúnez, K. (2019). Detection of Lotmaria passim in Africanized and European honey bees from Uruguay, Argentina and Chile. Journal of Invertebrate Pathology, 160, 95–97. https://doi.org/https://doi.org/10.1016/j.jip.2018.11.004
- Castillo, C., Wolf Veiga, P., Martin, J. L., Curran, C., & Pernal, S. F. (2017). Canadian national honey bee health survey. Proceedings of the 2017 American Bee Research Conference., 12–13 Jan, Bee World. 93(4): 106.
- Chantawannakul, P., Ward, L., Boonham, N., & Brown, M. (2006). A scientific note on the detection of honeybee viruses using real-time PCR (TaqMan) in Varroa mites collected from a Thai honeybee (Apis mellifera) apiary. Journal of Invertebrate Pathology, 91(1), 69–73. https://doi.org/https://doi.org/10.1016/j.jip.2005.11.001
- Chauzat, M. P., Jacques, A., Laurent, M., Bougeard, S., Hendrikx, P., Ribière, ‐Chabert, M., Epilobee Consortium. (2016). Risk indicators affecting honey bee colony survival in Europe, one year of surveillance. Apidologie, 4, 3-3.
- Chen, Y. P., Higgins, J. A., & Feldlaufer, M. F. (2005). Quantitative real-time reverse transcription-PCR analysis of deformed wing virus infection in the honeybee. (Apis mellifera L.). Applied and Environmental Microbiology 71(1), 436–441. https://doi.org/https://doi.org/10.1128/AEM.71.1.436-441.2005
- Chen, Y., Evans, J. D., Smith, I. B., & Pettis, J. S. (2008). Nosema ceranae is a long-present and wide-spread microsporidian infection of the European honey bee (Apis mellifera) in the United States. Journal of Invertebrate Pathology, 97(2), 186–188. https://doi.org/https://doi.org/10.1016/j.jip.2007.07.010
- Currie, R. W., & Gatien, P. (2006). Timing acaricide treatments to prevent Varroa destructor (Acari: Varroidae) from causing economic damage to honey bee colonies. The Canadian Entomologist, 138(2), 238–252. https://doi.org/https://doi.org/10.4039/n05-024
- D’Alvise, P., Seeburger, V., Gihring, K., Kieboom, M., & Hasselmann, M. (2019). Seasonal dynamics and co-occurrence patterns of honey bee pathogens revealed by high-throughout RT-qPCR analysis. Ecology and Evolution, 9(18), 10241–10252. https://doi.org/https://doi.org/10.1002/ece3.5544
- de Guzman, L. I., Simone-Finstrom, M., Frake, A. M., & Tokarz, P. (2019). Comb irradiation has limited, interactive effects on colony performance or pathogens in bees, Varroa destructor and wax based on two honey bee stocks. Insects, 10(1), 15. https://doi.org/https://doi.org/10.3390/insects10010015
- de Miranda, J. R., & Genersch, E. (2010). Deformed wing virus. Journal of Invertebrate Pathology, 103, S48–S61. Suppl 1: https://doi.org/https://doi.org/10.1016/j.jip.2009.06.012
- Dietemann, V., Nazzi, F., Martin, S. J., Anderson, D., Locke, B., Delaplane, K. S., Wauquiez, Q., Tannahill, C., Frey, E., Ziegelmann, B., Rosenkranz, P., & Ellis, J. D. (2013). Standard methods for Varroa research. Journal of Apicultural Research, 52(1), 1–4. In: Dietemann V, Ellis JD, Neumann P (Eds). The COLOSS BEEBOOK, Volume II: standard methods for Apis mellifera pest and pathogen research. https://doi.org/https://doi.org/10.3896/IBRA.1.52.1.09
- Fan, Q., Parmar, P., George, S., & Gallagher, L. (2018). An improved technique for quantifying infestation level of external mites (acari) on honey bees. Journal of Apicultural Research, 57(2), 317–320. https://doi.org/https://doi.org/10.1080/00218839.2017.1386347
- Frazer, J. L., Tham, K.-M., Reid, M., van Andel, M., McFadden, A. M. J., Forsgren, E., Pettis, J. S., & Pharo, H. (2015). First detection of Nosema ceranae in New Zealand honey bees. Journal of Apicultural Research, 54(4), 358–365. https://doi.org/https://doi.org/10.1080/00218839.2016.1161962
- Fries, I. (1988). Comb replacement and nosema disease (Nosema apis Z.) in honey bee colonies. Apidologie, 19(4), 343–354. https://doi.org/https://doi.org/10.1051/apido:19880402
- Fries, I. (2010). Nosema ceranae in European honey bees (Apis mellifera). Journal of Invertebrate Pathology, 103 (Suppl 1), S73–S79. https://doi.org/https://doi.org/10.1016/j.jip.2009.06.017
- Genersch, E., von der Ohe, W., Kaatz, H., Schroeder, A., Otten, C., Büchler, R., Berg, S., Ritter, W., Mühlen, W., Gisder, S., Meixner, M., Liebig, G., & Rosenkranz, P., (2010). The German bee monitoring project: a long term study to understand periodically high winter losses of honey bee colonies. Apidologie, 41(3), 332–352. https://doi.org/https://doi.org/10.1051/apido/2010014
- Giacobino, A., Molineri, A., Bulacio Cagnolo, N., Merke, J., Orellano, E., Bertozzi, E., Masciangelo, G., Pietronave, H., Pacini, A., Salto, C., & Signorini, M. (2016). Key management practices to prevent high infestation levels of Varroa destructor in honey bee colonies at the beginning of the honey yield season. Preventive Veterinary Medicine, 131, 95–102. https://doi.org/https://doi.org/10.1016/j.prevetmed.2016.07.013
- Gillingham, A. (2008). Beekeeping. Te Ara—the Encyclopaedia of New Zealand, Ministry for Culture & Heritage, New Zealand. Retrieved March 27, 2020, from http://www.TeAra.govt.nz/en/beekeeping/page-1
- Girma, A., Seo, W., & She, R. C. (2019). Antibacterial activity of varying UMF-graded Manuka honeys. PLoS ONE, 14(10), e0224495. https://doi.org/https://doi.org/10.1371/journal.pone.0224495
- Gisder, S., Hedtke, K., MöCkel, N., Frielitz, M.-C., Linde, A., & Genersch, E. (2010). Five-year cohort study of Nosema spp. in Germany: Does climate shape virulence and assertiveness of Nosema ceranae? Applied and Environmental Microbiology, 76(9), 3032–3038. https://doi.org/https://doi.org/10.1128/AEM.03097-09
- Gisder, S., Schüler, V., Horchler, L. L., Groth, D., & Genersch, E. (2017). Long-term temporal trends of Nosema spp. infection prevalence in northeast Germany: Continuous spread of Nosema ceranae, an emerging pathogen of honey bees (Apis mellifera), but no general replacement of Nosema apis. Frontiers in Cellular and Infection Microbiology, 7, 301. https://doi.org/https://doi.org/10.3389/fcimb.2017.00301
- Goldberg, J., Trewick, S. A., & Paterson, A. M. (2008). Evolution of New Zealand's terrestrial fauna: A review of molecular evidence. Philosophical Transactions of the Royal Society B: Biological Sciences, 363(1508), 3319–3334. https://doi.org/https://doi.org/10.1098/rstb.2008.0114
- Goodwin, R. M., & Van Eaton, C. (2001). Control of Varroa: A guide for New Zealand beekeepers. New Zealand Ministry of Agriculture and Forestry. https://doi.org/https://doi.org/10.1098/rstb.2008.0114
- Iwasaki, J., Barratt, B., Lord, J., Mercer, A. R., & Dickinson, K. J. M. (2015). The New Zealand experience of Varroa invasion highlights research opportunities for Australia. Ambio, 44(7), 694–704. https://doi.org/https://doi.org/10.1007/s13280-015-0679-z
- Jacques, A., Laurent, M., Ribière-Chabert, M., Saussac, M., Bougeard, S., Budge, G. E., Hendrikx, P., & Chauzat, M.-P., EPILOBEE Consortium (2017). A pan-European epidemiological study reveals honey bee colony survival depends on beekeeper education and disease control. PLoS ONE, 12(3), e0172591. https://doi.org/https://doi.org/10.1371/journal.pone.0172591
- Jamaludin, R., Hansen, M. F., Humphrey, S., & Tham, K. M. (2002). First isolation of Melissococcus plutonius in New Zealand. Surveillance, 29(3), 20–21.
- Johnston, M., McBride, M., Dahiya, D., Owusu-Apenten, R., & Nigam, P. S. (2018). Antibacterial activity of Manuka honey and its components: An overview. AIMS Microbiology, 4(4), 655–664. https://doi.org/https://doi.org/10.3934/microbiol.2018.4.655
- King, C. (2020). American foulbrood. Surveillance, 47(3), 42.
- Klee, J., Besana, A. M., Genersch, E., Gisder, S., Nanetti, A., Tam, D. Q., Chinh, T. X., Puerta, F., Ruz, J. M., Kryger, P., Message, D., Hatjina, F., Korpela, S., Fries, I., & Paxton, R. J. (2007). Widespread dispersal of the microsporidian Nosema ceranae, an emergent pathogen of the western honey bee, Apis mellifera. Journal of Invertebrate Pathology, 96(1), 1–10. https://doi.org/https://doi.org/10.1016/j.jip.2007.02.014
- Leahy, R. (2019). Getting the most out of our bees. The New Zealand Beekeeper, 27(3), 63–67.
- Lee, K., Reuter, G., & Spivak, M. (2010). Standardized sampling plan to detect Varroa density in colonies and apiaries. American Bee Journal, 150, 1151–1155.
- Locke, B., Forsgren, E., Fries, I., & de Miranda, J. R. (2012). Acaricide treatment affects viral dynamics in Varroa destructor infested honey bee colonies via both host physiology and mite control. Applied and Environmental Microbiology, 78(1), 227–235. https://doi.org/https://doi.org/10.1128/AEM.06094-11
- Locke, B., Low, M., & Forsgren, E. (2019). An integrated management strategy to prevent outbreaks and eliminate infection pressure of American foulbrood disease in a commercial beekeeping operation. Preventive Veterinary Medicine, 167, 48–52. https://doi.org/https://doi.org/10.1016/j.prevetmed.2019.03.023
- Lodesani, M., Costa, C., Serra, G., Colombo, R., & Sabatini, A. (2008). Acaricide residues in beeswax after conversion to organic beekeeping methods. Apidologie, 39(3), 324–333. https://doi.org/https://doi.org/10.1051/apido:2008012
- Loope, K. J., Baty, J. W., Lester, P. J., Wilson Rankin, E. E. (2019). Pathogen shifts in a honeybee predator following the arrival of the Varroa mite. Proceedings of the Royal Society B: Biological Sciences 286(1894): 20182499.
- Lourenço, A., Mackert, A., Cristino, A., & Simões, Z. (2008). Validation of reference genes for gene expression studies in the honey bee, Apis mellifera, by quantitative real-time RT-PCR. Apidologie, 39(3), 372–385. https://doi.org/https://doi.org/10.1051/apido:2008015
- Martin, S. J., Highfield, A. C., Brettell, L., Villalobos, E. M., Budge, G. E., Powell, M., Nikaido, S., & Schroeder, D. C. (2012). Global honey bee viral landscape altered by a parasitic mite. Science, 336(6086), 1304–1306. https://doi.org/https://doi.org/10.1126/science.1220941
- McFadden, A. M. J., Tham, K., Stevenson, M., Goodwin, M., Pharo, H., Taylor, B., Munro, G., Owen, K., Peacock, L., Stanslawek, W. L., & Stone, M. (2014). Israeli acute paralysis virus not detected in Apis mellifera in New Zealand in a national survey. Journal of Apicultural Research, 53(5), 520–527. https://doi.org/https://doi.org/10.3896/IBRA.1.53.5.03
- Ministry for Primary Industries. (2015). Import health standard for specified processed bee products; updated 22 December 2015. New Zealand Government. Retrieved March 27, 2020, from https://www.mpi.govt.nz/dmsdocument/1176/direct
- Ministry for Primary Industries (2020). Apiculture – Ministry for Primary Industries, Apiculture Monitoring Programme. Retrieved March 18, 2021, from https://www.mpi.govt.nz/dmsdocument/44068-Apiculture-Moniotoring-Report-2020
- Mondet, F., de Miranda, J. R., Kretzschmar, A., Le Conte, Y., & Mercer, A. R. (2014). On the front line: Quantitative virus dynamics in honeybee (Apis mellifera L.) colonies along a new expansion front of the parasite Varroa destructor. PLoS Pathogens, 10(8), e1004323. https://doi.org/https://doi.org/10.1371/journal.ppat.1004323
- Morawetz, L., Köglberger, H., Griesbacher, A., Derakhshifar, I., Crailsheim, K., Brodschneider, R., & Moosbeckhofer, R. (2019). Health status of honey bee colonies (Apis mellifera) and disease-related risk factors for colony losses in Austria. PLoS ONE, 14(7), e0219293. https://doi.org/https://doi.org/10.1371/journal.pone.0219293
- Murray, Z. L., & Lester, P. J. (2015). Confirmation of Nosema ceranae in New Zealand and a phylogenetic comparison of Nosema spp. Journal of Apicultural Research, 54(2), 101–104. https://doi.org/https://doi.org/10.1080/00218839.2015.1101240
- New Zealand Government. (2016). Biosecurity (Notifiable Organisms) Order 2016: Order in Council (LI 2016/73). Retrieved March 28, 2020, from https://www.mpi.govt.nz/dmsdocument/6403/direct
- NIWA. (2020). National Institute of Water and Atmospheric Research: Overview of New Zealand's climate. Retrieved March 28, 2020, from https://niwa.co.nz/education-and-training/schools/resources/climate/overview climate
- Office of the Auditor-General New Zealand (2002). Case Study 4 – Response to the Incursion of the Varroa Bee Mite. In Report of the Controller and Auditor-General. Management of biosecurity risks: Case studies. Retrieved March 28, 2020, from https://oag.parliament.nz/2002/biosecurity-case-studies
- Ondrus, P. (2017). The seasonality, thermal dependence, and spread of Nosema ceranae in new locales. Bee World, 94(4), 105–109. https://doi.org/https://doi.org/10.1080/0005772X.2017.1382041
- Pharo, H. J. (2006). Risk of introduction of European foulbrood in honey bee products imported into New Zealand. International Symposia on Veterinary Epidemiology and Economics Proceedings., ISVEE 11: Proceedings of the 11th Symposium of the International Society for Veterinary Epidemiology and Economics (Theme 5 - Evaluation of animal disease: Risk analysis session), p. 615.
- Phiri, B. J., & Rich, C. L. (2019). Honey bee exotic pest and disease surveillance report. Surveillance, 46(3), 38–39.
- Ramsey, S. D., Ochoa, R., Bauchan, G., Gulbronson, C., Mowery, J. D., Cohen, A., Lim, D., Joklik, J., Cicero, J. M., Ellis, J. D., Hawthorne, D., & vanEngelsdorp, D. (2019). Varroa destructor feeds primarily on honey bee fat body tissue and not hemolymph. Proceedings of the National Academy of Sciences, 116(5), 1792–1801. https://doi.org/https://doi.org/10.1073/pnas.1818371116
- Ravoet, J., Maharramov, J., Meeus, I., De Smet, L., Wenseleers, T., Smagghe, G., & de Graaf, D. C. (2013). Comprehensive bee pathogen screening in Belgium reveals Crithidia mellificae as a new contributory factor to winter mortality. PLoS ONE, 8(8), e72443. https://doi.org/https://doi.org/10.1371/journal.pone.0072443
- Roetschi, A., Berthoud, H., Kuhn, R., & Imdorf, A. (2008). Infection rate based on quantitative real-time PCR of Melissococcus plutonius, the causal agent of European foulbrood, in honey bee colonies before and after apiary sanitation. Apidologie, 39(3), 362–371. https://doi.org/https://doi.org/10.1051/apido:200819
- Rosenkranz, P., Aumeier, P., & Ziegelmann, B. (2010). Biology and control of Varroa destructor. Journal of Invertebrate Pathology, 103, S96–S119. Suppl 1: https://doi.org/https://doi.org/10.1016/j.jip.2009.07.016
- Runckel, C., Derisi, J. L., & Flenniken, M. (2014). A draft genome of the honey bee trypanosomatid parasite Crithidia mellificae. PLoS ONE, 9(4), e95057. https://doi.org/https://doi.org/10.1371/journal.pone.0095057
- Runckel, C., Flenniken, M. L., Engel, J. C., Ruby, J. G., Ganem, D., Andino, R., & DeRisi, J. L. (2011). Temporal analysis of the honey bee microbiome reveals four novel viruses and seasonal prevalence of known viruses, Nosema, and Crithidia. PLoS ONE, 6(6), e20656. https://doi.org/https://doi.org/10.1371/journal.pone.0020656
- Schwarz, R. S., Bauchan, G. R., Murphy, C. A., Ravoet, J., de Graaf, D. C., & Evans, J. D. (2015). Characterization of Two Species of Trypanosomatidae from the Honey Bee Apis mellifera: Crithidia mellificae Langridge and McGhee, and Lotmaria passim n. gen., n. sp. Journal of Eukaryotic Microbiology, 62(5), 567–583. https://doi.org/https://doi.org/10.1111/jeu.12209
- Singers, N. J. D., & Rogers, G. M. (2014). A classification of New Zealand’s terrestrial ecosystems. Science for Conservation 325. Department of Conservation, 87p.
- Steinhauer, N. A., Rennich, K., Wilson, M. E., Caron, D. M., Lengerich, E. J., Pettis, J. S., Rose, R., Skinner, J. A., Tarpy, D. R., Wilkes, J. T., vanEngelsdorp, D., & the Bee Informed Partnership. (2014). A national survey of managed honey bee 2012-2013 annual colony losses in the USA: Results from the Bee Informed Partnership. Journal of Apicultural Research, 53(1), 1–18. https://doi.org/https://doi.org/10.3896/IBRA.1.53.1.01
- Tentcheva, D., Gauthier, L., Zappulla, N., Dainat, B., Cousserans, F., Colin, M. E., & Bergoin, M. (2004). Prevalence and seasonal variations of six bee viruses in Apis mellifera L. and Varroa destructor mite populations in France. Applied and Environmental Microbiology, 70(12), 7185–7191. https://doi.org/https://doi.org/10.1128/AEM.70.12.7185-7191.2004
- Todd, J., De Miranda, J., & Ball, B. (2007). Incidence and molecular characterization of viruses found in dying New Zealand honey bee (Apis mellifera) colonies infested with Varroa destructor. Apidologie, 38(4), 354–367. https://doi.org/https://doi.org/10.1051/apido:2007021
- Traver, B. E., Williams, M. R., & Fell, R. D. (2012). Comparison of within hive sampling and seasonal activity of Nosema ceranae in honey bee colonies. Journal of Invertebrate Pathology, 109(2), 187–193. https://doi.org/https://doi.org/10.1016/j.jip.2011.11.001
- Traynor, K. S., Rennich, K., Forsgren, E., Rose, R., Pettis, J., Kunkel, G., Madella, S., Evans, J., Lopez, D., & vanEngelsdorp, D. (2016). Multiyear survey targeting disease incidence in US honey bees. Apidologie, 47(3), 325–347. https://doi.org/https://doi.org/10.1007/s13592-016-0431-0
- Tritschler, M., Retschnig, G., Yañez, O., Williams, G. R., & Neumann, P. (2017). Host sharing by the honey bee parasites Lotmaria passim and Nosema ceranae. Ecology and Evolution, 7(6), 1850–1857. https://doi.org/https://doi.org/10.1002/ece3.2796
- Tsevegmid, K., Neumann, P., & Yañez, O. (2016). The honey bee pathosphere of Mongolia: European viruses in central Asia. PLoS ONE, 11(3), e0151164. https://doi.org/https://doi.org/10.1371/journal.pone.0151164
- van der Zee, R., Gray, A., Holzmann, C., Pisa, L., Brodschneider, R., Chlebo, R., Coffey, M. F., Kence, A., Kristiansen, P., Mutinelli, F., Nguyen, B. K., Noureddine, A., Peterson, M., Soroker, V., Topolska, G., Vejsnaes, F., & Wilkins, S. (2013). Standard survey methods for estimating colony losses and explanatory risk factors in Apis mellifera. Journal of Apicultural Research, 52(4), 1–36. https://doi.org/https://doi.org/10.3896/IBRA.1.52.4.18
- Van Eaton, C. (2014). Manuka: the biography of an extraordinary honey. Exisle Publishing.
- vanEngelsdorp, D., Evans, J. D., Saegerman, C., Mullin, C., Haubruge, E., Nguyen, B. K., Frazier, M., Frazier, J., Cox-Foster, D., Chen, Y., Underwood, R., Tarpy, D. R., & Pettis, J. S. (2009). Colony collapse disorder: A descriptive study. PLoS ONE, 4(8), e6481. https://doi.org/https://doi.org/10.1371/journal.pone.0006481
- Vejnovic, B., Stevanovic, J., Schwarz, R. S., Aleksic, N., Mirilovic, M., Jovanovic, N. M., & Stanimirovic, Z. (2018). Quantitative PCR assessment of Lotmaria passim in Apis mellifera colonies co-infected naturally with Nosema ceranae. Journal of Invertebrate Pathology, 151, 76–81. https://doi.org/https://doi.org/10.1016/j.jip.2017.11.003
- Waters, T. L. (2018). The distribution and population dynamics of the honey bee pathogens Crithidia mellificae and Lotmaria passim in New Zealand [Master’s thesis]. School of Biological Sciences, Victoria University of Wellington.
- Wu, J. Y., Anelli, C. M., & Sheppard, W. S. (2011). Sub-lethal effects of pesticide residues in brood comb on worker honey bee (Apis mellifera) development and longevity. PLoS ONE., 6(2), e14720. https://doi.org/https://doi.org/10.1371/journal.pone.0014720
- Wu, J. Y., Smart, M. D., Anelli, C. M., & Sheppard, W. S. (2012). Honey bees (Apis mellifera) reared in brood combs containing high levels of pesticide residues exhibit increased susceptibility to nosema (microsporidia) infection. Journal of Invertebrate Pathology, 109(3), 326–329. https://doi.org/https://doi.org/10.1016/j.jip.2012.01.005
- Yoshiyama, M., & Kimura, K. (2011). Distribution of Nosema ceranae in the European honey bee, Apis mellifera in Japan. Journal of Invertebrate Pathology, 106(2), 263–267. https://doi.org/https://doi.org/10.1016/j.jip.2010.10.010