Abstract
During the last decades, the beekeeping industry has seen very few changes and improvements on the widely used Langstroth wooden hive. The recent development of new insulation materials with higher proprieties compared to wood could provide better alternatives for the beekeeping industry. In this study, we tested the winter insulation of polyurethane honey bee hives and compare it with the traditional Langstroth wooden hives. Eighteen honey bee colonies, equal in size, composed of nine polyurethane and nine wooden hives were randomly selected and each hive was equipped with a sensor to document the inner hive temperature and humidity. Our results show that polyurethane hives maintained a significantly (P < 0.001) higher overall temperature (10.20 ± 0.04 °C) than wooden hives (9.73 ± 0.05 °C) with a significantly more optimal relative humidity (52.05%) compared to the wooden hives (62.50%). Inner temperature patterns of the wooden hives exhibited pronounced oscillations compared to the polyurethane hives. Both hive groups showed significant differences (P < 0.001) in temperature insulation between day and night cycles. However, the polyurethane hives seem to provide better stability in humidity between days and nights compared to Langstroth wooden hives.
Introduction
The vast majority of modern managed honey bee (Apis mellifera L.) operations host bees in frame-movable hives which slightly varies in their design between models and countries. Up until today, the most popular and widely used hive by professional and migratory beekeepers remains the 10-frame Langstroth hive. Since its establishment in 1852, the design of the Langstroth movable frame hive (Langstroth, Citation1852) has not fundamentally changed. Nevertheless, prior to the establishment of modern hives, honey bees were raised and sheltered in different types of boxes and hives which varied in shape, size, material used, and honeycomb accessibility (Abbé, Citation1948). Despite the differences in cavity size and number of movable frames in the modern hives, the hive material itself remained the same which is the softwood. The widely used Langstroth hive is a simple wooden box, open at the top and can accommodate at the bottom either a screened-bottom board, usually used for Varroa mite monitoring or a plain board which is more common (Langstroth, Citation1852; Mitchell, Citation2019a). The main objective of a hive is to shelter bees and provide them with a cavity which they can efficiently thermoregulate to maintain their brood nest within an optimal and narrow range of temperature (34–35 °C); critical for brood development (Abou-Shaara et al., Citation2017). Langstroth hives are made of soft lumber wood and its wall thickness is approximately 2.3 cm which provides relatively low insulation value for the same thickness compared to other new commercial materials such as polyurethane, fiberglass, and rock wool blown. The insulation value (R-value) is the measurement of a material’s capacity to resist heat flow from one side to the other; also called the effectiveness of insulation for a given material. Therefore, the hive’s material and its R-value are critical as bees have to adjust and maintain both inner hive temperature and humidity at optimal levels in order to produce healthy brood and survive the winter season (Mitchell, Citation2019b). Non optimal temperature or fluctuation in the brood nest could lead to brood damage, adult bee deformation and decline in cognition abilities and development of diseases (Dalmon et al., Citation2019; Jones et al., Citation2005; Meikle et al., Citation2017; Zhu et al., Citation2018). Based on the wall thickness and type of wood used in the Langstroth hive, its insulation value (R) ranges between (1.2–1.3) while other newer material such as polyurethane provides much higher value (6.2) in equivalent thicknesses (University of Washington/R-Value Table).
Monitoring honey bee colony thermoregulation has been conducted for multiple purposes and is usually carried out by placing small sensors in the central brood area (lower chamber) or within honey supers (Alburaki et al., Citation2017; Meikle et al., Citation2016; Stalidzans & Berzonis, Citation2013; Zacepins et al., Citation2016). Hive insulation is crucially important during the winter time when the temperature may drop below freezing. Honey bee winter mortality remains a major contributor to colony losses worldwide. In the USA, winter mortality ranged between 10 to 49% across States in the late survey of 2019/2020 (Bee Informed Partnership). Regardless of the outdoor temperature, honey bees have to maintain their winter cluster at close to optimal temperature (34–35 °C). This requires a significant amount of energy which bees generate via thermoregulatory behaviors such as clustering, fanning, increase in metabolic rate, and locomotor activity (Kronenberg & Heller, Citation1982). Thus, poor hive insulation extended and inclement winter could inevitably lead to starvation and depletion of winter resources available to bees and stored in-hive. In addition, low temperatures during the winter could also be associated with the down-regulation of bee immune response and increased infections (Steinmann et al., Citation2015). Consistent with this possibility, higher Deformed wing virus (DWV) titers have been found in caged bees at low temperatures compared with warmer ones (Di Prisco et al., Citation2011). Although this result has not been yet replicated at colony-level, the effects of hive’s thermal insulation on honey bees’ susceptibility to virus and other pathogens, such as fungus, remains to be evaluated.
In order to cope with extremely low winter temperatures, indoor overwintering (McCutcheon, Citation1984) has become an increasingly more common practice. For instance, in 2006, 25% of Alberta (Canada) beekeepers overwintered their colonies indoor (Hartman & Nasr, Citation2007). Despite its advantage in minimizing winter colony loss, indoor overwintering remains an economical burden for beekeepers. Improving beekeeping practices is an effective approach to increase productivity and limit bee mortality. The hive material has remained static for a long time and exploring newer commercially available materials could potentially offer more optimal in-hive conditions for bees. In this study conducted during the winter season, we compared by continuous monitoring of inner temperature and humidity, the thermoregulation of honey bees hosted in two different types of hives (wood and polyurethane) to determine which type of hive materials provides better insulation for honey bees.
Materials and methods
Honey bee hives
Eighteen honey bee colonies, equal in size, were selected from our current stock at the USDA-ARS Bee Research Lab, which is mainly composed of Italian Apis mellifera ligustica and Carniolan Apis mellifera carnica bee subspecies. Nine colonies were transferred to classic Langstroth wooden hives composed of two chambers, plain bottom boards, inner and top covers, and standard entrance doors, and nine others were transferred to newly purchased polyurethane hives (BetterBee, NY, USA) (). The polyurethane hives used in this study, similar to the wooden hives, were composed of two deep10-frame chambers (). All colonies were placed at the same location on the USDA-ARS campus located in Beltsville-Maryland (). This study lasted four months and was conducted during the winter from Dec 2020 to Mar 2021. All experimental colonies received the same standard beekeeping care and were similarly fed sugar syrup solution and prepared for wintering.
Figure 1. Two different types of hives (Polyurethane, wood) used in this study as well as the overall average of both inner hive temperature and humidity for each hive groups. ANOVA was conducted at three levels of significance: *P < 0.05, **P < 0.01, ***P < 0.001.
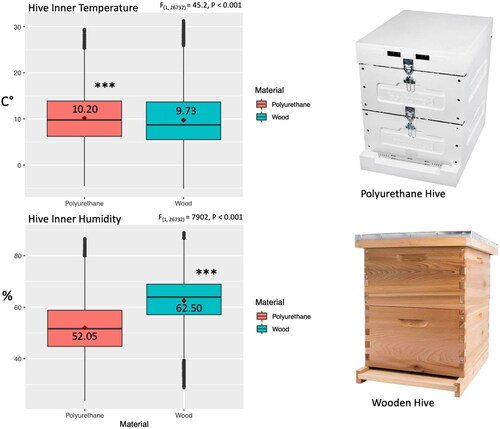
Table 1. Description of the studied hives and groups, numbers, location and inner-hive sensor used for data collection. Location and date of the study are also given.
Humidity and temperature sensor
In each colony, a humidity and temperature sensor, integrated into a new wooden frame, was placed in the center of the brood box. These sensors named (HM-1 monitor) were purchased from SolutionBee LLC (NC, USA) and are specifically designed and adjusted to monitor the thermoregulation of honey bee hives. Sensors were set to record one single read of both humidity and temperature every hour.
Data collection
The data collection was conducted with no disturbance to the colonies through wireless connectivity. Data were collected monthly using an android device on which (B-Ware, version 1.24) app was downloaded. This app is designed and made available for free by the sensor’s manufacturer (Solution Bee, LLC). Data were subsequently uploaded to a cloud and downloaded from the cloud in form of Excel files (.cvs) for further statistical analysis.
Statistical analysis
All statistical analysis and figure generation were performed in R environment (R Core Team, Citation2011). This study was conducted based on 2 treatment groups (polyurethane and wooden hives) and 9 technical replicates for each treatment group (). In order to study the effect of day and night on the hive insulation, the dataset was sorted out into two different categories: (1) Day time: sunshine to sunset (6 am to 6 pm) and (2) Night time: sunset to sunshine (6 pm to 6 am). Figures were generated using the following R packages and libraries: “tidyverse,” “ggplot2” and “ggpubr.” ANOVA was conducted at 95% confidential interval with three levels of significance: *P < 0.05, **P < 0.01, ***P < 0.001. All boxplots displayed the medians, quantiles and no outliers were removed from the dataset for maximum accuracy. In some instances, the mean values were given along with the medians.
Results
Inner hive temperature
The overall average of the inner hive temperature was significantly higher in the polyurethane hives compared to the wooden hives; (10.20 ± 0.04 °C) versus (9.73 ± 0.05 °C) respectively (F(1,26732)=45.2, P < 0.001) (). The longitudinal temperature patterns of two randomly selected colonies (polyurethane 174 and Wood 169) showed more pronounced oscillations in the wooden hive compared to the polyurethane (). A similar finding was observed for the overall average temperature of the two groups (). Both polyurethane and wooden hives exhibited significant differences (P < 0.001) in the inner temperature between day and night ().
Figure 2. Longitudinal display of temperature patterns for two randomly selected colonies; one from each studied group (Polyurethane and wooden hives). Temperature variations between day (sunshine to sunset) and night are displayed in boxplots for each hive type. ANOVA shows significant differences in hive inner temperature between day and night (P < 0.001) in both studied categories.
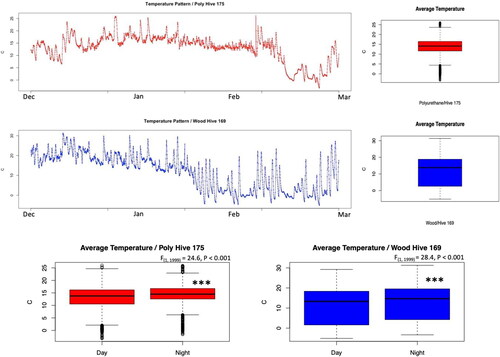
Inner hive humidity
Polyurethane hives maintained a significantly lower overall inner hive humidity compared to the wooden hives, (52.05%) and (62.50%) respectively, (F(1,26732)= 7902, P < 0.001) (). Overall humidity data, displayed hourly, shows constant elevation in the inner humidity of the wooden hives throughout the winter (). The hive materials seem to have a different effect on the humidity when data is treated by day versus night. Despite obtaining significant differences in the hive inner humidity between day and night in both hive types, it is clear that polyurethane hives offered less day-night humidity variation (F(1,1998)= 4.6, P < 0.05) compared to the wooden hives (F(1,1999)= 12.6, P < 0.001) ().
Figure 3. Humidity patterns (Dec to Mar) for two randomly selected colonies (same colonies of ); one from each studied group (Polyurethane and wooden hives). Overall average humidity is also given for each hive. In-hive humidity variations between day (sunshine to sunset) and night are displayed in boxplots for each of the two hive types. Statistical analysis (ANOVA) was conducted at 95% confidential interval and shows significant differences in inner hive humidity between day and night.
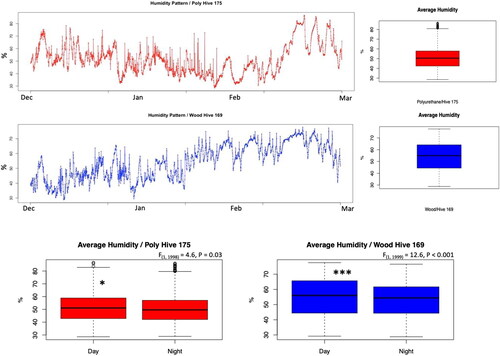
Outdoor temperature
Hive outdoor temperatures were recorded at the apiary location during the time of this experiment. They showed significant fluctuations and ranged between −10 °C and 20 °C (). However, the average outdoor temperature during this period was 0 °C ().
Discussion
Our study was conducted to address a growing body of evidence related to the poor insulation of the widely used Langstroth wooden hives (Langstroth, Citation1852). The wall thickness (∼2.3 cm) of this wooden hive may not provide enough insulation (R = 1.2) compared to newer polyurethane hives (R = 6.2) designed with better insulating material (Cook et al., Citation2021). Indeed, our data conducted on 18 honey bee colonies equally divided into 2 groups of polyurethane and wooden hives, showed that polyurethane hives maintained a significantly higher inner temperature and lower humidity than the wooden hives. The insulation factor mentioned above is of great importance as, regardless of the external conditions, honey bees must maintain optimal or close to optimal nest temperature and humidity (Becher et al., Citation2009; Fahrenholz et al., Citation1989; Sadler & Nieh, Citation2011). In the case of our study, the average outside temperature was close to 0 °C while inner hive temperature averaged 10.2 °C and 9.73 °C for polyurethane and wooden hives respectively. It is important to mention that our recorded temperatures are not those of the honey bee clusters but rather those of the inner hive cavities. The temperature in the center of an overwintering cluster of bees was estimated to range between (12–33.5) °C with an average of 21.3 °C (Fahrenholz et al., Citation1989), which is higher than the average cavity temperatures recorded in this study. Besides the insulation factor of the hive material, a recent study showed that honey harvest or the process of removing honeycombs from the hives would lead to loss of considerable thermal mass in the hive reducing the internal thermal stability (Cook et al., Citation2021). Such thermal loss will require significant energy to be replaced as well as the reheating of the extracted honeycombs when placed back in the colony. Although these observations are not applicable on the current study which was conducted in the winter time, they should be considered in other seasons. Both types of hives showed significant variation in their inner temperature between day and night. Nonetheless, the polyurethane provided a more stable humidity between day and night compared to the wooden hives. The overall data showed lower and more optimal relative humidity in the polyurethane hives (52.05%) compared to the wooden hives (62.5%), and this is mainly due to better aeration in the polyurethane hives which are equipped with top and bottom vents while the wooden Langstroth hives are fully sealed. Moreover, the wood's ability to absorb humidity and release it at higher temperatures could have contributed to elevating the overall inner humidity. This argument is well supported by the data showing significantly higher humidity in the wooden hives during the day compared to the night time (). In the contrary, polyurethane as a material seems to show less interaction with the humidity of the cavities providing more stability between day and night (). Honey bees use different thermoregulatory behaviors to adjust non-optimal conditions within the hive (Kronenberg & Heller, Citation1982). During the winter time, clustering and increase in the metabolic rate are the main remedies to counter cold temperature (Allen, Citation1959). This behavior requires significant energy obtained via higher honey consumption by bees. Thus, better hive insulation can play a crucial role to minimize the amount of energy required to thermoregulate the hive cavity and subsequently, decrease honey consumption which, if depleted, can lead to colony winter starvation and death.
In conclusion, this study shows that small changes and improvements in beekeeping practices and equipment, including hive types and materials, can contribute in reducing honey bee mortality rates, particularly winter mortality. Our data shows that hives made of polyurethane provide better insulation than hives made of softwood. This study evaluated overwintering colonies and it remains to be seen whether similar conclusions can be drawn during other seasons and higher outdoor temperatures.
Acknowledgments
We would like to thank Dr. Wissam Alburaki for proofreading the final version of this manuscript and Javier Maradiaga Melara for assistance in the field.
Disclosure statement
The authors declare no conflict of interest.
Funding
This research was funded by the USDA-ARS.
References
- Abbé, W. (1948). 2, Cours Sablon - Clermont-Ferrand, p. 293.
- Abou-Shaara, H. F., Owayss, A. A., Ibrahim, Y. Y., & Basuny, N. K. (2017). A review of impacts of temperature and relative humidity on various activities of honey bees. Insectes Sociaux, 64(4), 455–463. https://doi.org/10.1007/s00040-017-0573-8
- Alburaki, M., Steckel, S. J., Williams, M. T., Skinner, J. A., Tarpy, D. R., Meikle, W. G., Adamczyk, J., & Stewart, S. D. (2017). Agricultural landscape and pesticide effects on honey bee (Hymenoptera: Apidae) biological traits. Journal of Economic Entomology, 110(3), 835–847. https://doi.org/10.1093/jee/tox111
- Allen, M. D. (1959). Respiration rates of worker honeybees of different ages and at different temperatures. Journal of Experimental Biology, 36(1), 92–101. https://doi.org/10.1242/jeb.36.1.92
- Becher, M. A., Scharpenberg, H., & Moritz, R. F. A. (2009). Pupal developmental temperature and behavioral specialization of honeybee workers (Apis mellifera L.). Journal of Comparative Physiology. A, Neuroethology, Sensory, Neural, and Behavioral Physiology, 195(7), 673–679. https://doi.org/10.1007/s00359-009-0442-7
- Cook, D., Blackler, A., McGree, J., & Hauxwell, C. (2021). Thermal impacts of apicultural practice and products on the honey bee colony. Journal of Economic Entomology, 114(2), 538–546. https://doi.org/10.1093/jee/toab023
- Dalmon, A., Peruzzi, M., Le Conte, Y., Alaux, C., & Pioz, M. (2019). Temperature-driven changes in viral loads in the honey bee Apis mellifera. Journal of Invertebrate Pathology, 160, 87–94. https://doi.org/10.1016/j.jip.2018.12.005
- Di Prisco, G., Zhang, X., Pennacchio, F., Caprio, E., Li, J., Evans, J. D, Degrandi-Hoffman, G., Hamilton, M., & Chen, Y. P. (2011). Dynamics of persistent and acute deformed wing virus infections in honey bees, Apis mellifera. Viruses, 3, 2425–2441.
- Fahrenholz, L., Lamprecht, I., & Schricker, B. (1989). Thermal investigations of a honey bee colony: Thermoregulation of the hive during summer and winter and heat production of members of different bee castes. Journal of Comparative Physiology B, 159(5), 551–560. https://doi.org/10.1007/BF00694379
- Hartman, M., & Nasr, M. (2007). Alberta Beekeepers Overwintering Loss Survey 2007 agricgovabca.
- Jones, J. C., Helliwell, P., Beekman, M., Maleszka, R., & Oldroyd, B. P. (2005). The effects of rearing temperature on developmental stability and learning and memory in the honey bee, Apis mellifera. Journal of Comparative Physiology. A, Neuroethology, Sensory, Neural, and Behavioral Physiology, 191(12), 1121–1129. https://doi.org/10.1007/s00359-005-0035-z
- Kronenberg, F., & Heller, C. H. (1982). Colonial thermoregulation in honey bees (Apis mellifera). Journal of Comparative Physiology B, 148(1), 65–76. https://doi.org/10.1007/BF00688889
- Langstroth, L. (1852). Beehive. USA patent.
- McCutcheon, D. M. (1984). Indoor wintering of hives. Bee World, 65(1), 19–37. https://doi.org/10.1080/0005772X.1984.11098768
- Meikle, W. G., Weiss, M., & Stilwell, A. R. (2016). Monitoring colony phenology using within-day variability in continuous weight and temperature of honey bee hives. Apidologie, 47(1), 1–14. https://doi.org/10.1007/s13592-015-0370-1
- Meikle, W. G., Weiss, M., Maes, P. W., Fitz, W., Snyder, L. A., Sheehan, T., Mott, B. M., & Anderson, K. E. (2017). Internal hive temperature as a means of monitoring honey bee colony health in a migratory beekeeping operation before and during winter. Apidologie, 48(5), 666–680. https://doi.org/10.1007/s13592-017-0512-8
- Mitchell, D. (2019a). Nectar, humidity, honey bees (Apis mellifera) and varroa in summer: A theoretical thermofluid analysis of the fate of water vapour from honey ripening and its implications on the control of Varroa destructor. Journal of the Royal Society Interface, 16(156), 20190048. https://doi.org/10.1098/rsif.2019.0048
- Mitchell, D. (2019b). Thermal efficiency extends distance and variety for honeybee foragers: Analysis of the energetics of nectar collection and desiccation by Apis mellifera. Journal of the Royal Society Interface, 16(150), 20180879. https://doi.org/10.1098/rsif.2018.0879
- R Core Team. (2011). R: A language and environment for statistical computing. Austria: R Foundation for Statistical Computing.
- Sadler, N., & Nieh, J. C. (2011). Honey bee forager thoracic temperature inside the nest is tuned to broad-scale differences in recruitment motivation. The Journal of Experimental Biology, 214(Pt 3), 469–475. https://doi.org/10.1242/jeb.049445
- Stalidzans, E., & Berzonis, A. (2013). Temperature changes above the upper hive body reveal the annual development periods of honey bee colonies. Computers and Electronics in Agriculture, 90, 1–6. https://doi.org/10.1016/j.compag.2012.10.003
- Steinmann, N., Corona, M., Neumann, P., & Dainat, B. (2015). Overwintering is associated with reduced expression of immune genes and higher susceptibility to virus infection in honey bees. PLoS One, 10(6), e0129956. https://doi.org/10.1371/journal.pone.0129956
- Zacepins, A., Kviesis, A., Stalidzans, E., Liepniece, M., & Meitalovs, J. (2016). Remote detection of the swarming of honey bee colonies by single-point temperature monitoring. Biosystems Engineering, 148, 76–80. https://doi.org/10.1016/j.biosystemseng.2016.05.012
- Zhu, X., Xu, X., Zhou, S., Wang, Q., Chen, L., Hao, Z., & Zhou, B. (2018). Low temperature exposure (20 °C) during the sealed brood stage induces abnormal venation of honey bee wings. Journal of Apicultural Research, 57(3), 458–465. https://doi.org/10.1080/00218839.2017.14125