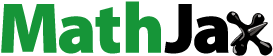
Abstract
The most devastating pest to honey bees (Apis mellifera) worldwide is the parasitic mite Varroa destructor. The development of miticide-resistant mite populations has been a major driver of colony loss in many countries. We investigated the threat Varroa poses to honey bee populations in New Zealand and tested the effectiveness of the two most popular chemical treatments used by beekeepers. Colony losses reported by New Zealand beekeepers have risen over five consecutive years from 2017 to 2021, as have the proportion of losses attributed to Varroa, with this parasite found to be the main driver of colony loss in 2021. Varroa resistance to miticide treatments flumethrin and amitraz was tested. The concentration of flumethrin required to kill 50% of the mites (LC50) was 156 µg/g, 26 times greater than the adjusted LC50 value of 6 µg/g observed in a trial also conducted in New Zealand in 2003, indicating evidence of developing mite resistance to flumethrin in New Zealand. Molecular analyses searching for mutations in the Varroa genome known to be associated with flumethrin resistance found no evidence of such mutations, suggesting that any extant resistance to flumethrin has evolved independently in New Zealand. No evidence of resistance to amitraz was found, as the LC50 value of 12 µg/g was lower than what was observed in the 2003 trial (141 µg/g). Further development of integrated pest management, such as with gene-silencing RNA interference (RNAi) and selective breeding of Varroa-resistant bees, is needed to effectively manage a parasite that threatens global agriculture.
Introduction
The western honey bee, Apis mellifera, plays a critical role in pollination across the globe (Aizen & Harder, Citation2009). Reliance on pollinator-dependent crops has risen over recent decades (Aizen et al., Citation2019) and there is evidence of pollinator limitation in many fruit and vegetable crops as honey bee stocks struggle to keep up with demand (Breeze et al., Citation2014; Reilly et al., Citation2020). The loss of pollination services not only threatens food security but also poses a significant risk to economies that depend on the international trade of pollinated crops (Eilers et al., Citation2011; Vanbergen & Pollinators Initiative, Citation2013; Murphy et al., Citation2022). A reduction in honey bee health and rising hive mortality are the main causes of the discrepancy between the supply and demand of bee colonies for pollination purposes (Dolezal et al., Citation2019; Steinhauer et al., Citation2021). Stressors such as parasites, pesticides and disease are recognised drivers of colony loss (Goulson et al., Citation2015; Di Prisco et al., Citation2016). The most significant threat to the apiculture industry worldwide is frequently cited as the ectoparasite Varroa destructor (Rosenkranz et al., Citation2010; Traynor et al., Citation2020; Jack & Ellis, Citation2021). This parasite feeds on the fat body of adult bees, a tissue crucial to bee health that is involved in immune function, the detoxification of pesticides and winter survival (Amdam et al., Citation2004; Ramsey et al., Citation2019; Han et al., Citation2024). Varroa is also a vector for honey bee viruses, particularly the Deformed wing virus (DWV) (Wilfert et al., Citation2016), which is associated with a wide range of clinical symptoms including crippled wings, cognitive impairment and reduced lifespan (Dainat et al., Citation2012; Francis et al., Citation2013). Both Varroa and DWV are strong predictors of colony collapse during winter (Dainat et al., Citation2012; Francis et al., Citation2013) and infested colonies may typically collapse within three years if they are not treated (Rosenkranz et al., Citation2010).
The most widely used Varroa control methods currently on the market are synthetic or organic miticides. These chemicals are usually applied as impregnated strips which are placed within the beehive and left for a period of four to ten weeks. Of the synthetic miticides, pyrethroid and formamidine based treatments have historically been popular choices for mite control. Pyrethroids specifically act on voltage-gated sodium channels, prolonging the opening of channels, causing paralysis and death (Narahashi, Citation2000; Wang et al., Citation2003). Formamidines are designed to target and stimulate alpha-2 adrenoceptors, interfering with the nervous system, which can result in a variety of outcomes, including impairment of consciousness and convulsions (Dekeyser & Downer, Citation1994). Synthetic miticides are selected and developed based on their ability to control Varroa without killing their bee host.
A key issue with Varroa control is the development of resistance to synthetic chemical miticides (Hernández-Rodríguez et al., Citation2021). Resistance to pyrethroid-based treatments was first reported in Italy around 1991 (Martin, Citation2004), and since then, pyrethroid-resistant mites have been found in the United Kingdom, Europe, Canada and USA (Martin, Citation2004; Mitton et al., Citation2022). Amino acid substitutions in the voltage-gated sodium channel protein at position 925 in particular have been observed in a number of mite populations that demonstrated resistance to pyrethroids (Millán‐Leiva et al., Citation2021). Incidences of resistance to formamidine-based Varroa treatments have been less common, although inefficacy has been detected in some studies (Thompson et al., Citation2002; Kamler et al., Citation2016; Higes et al., Citation2020; Rinkevich, Citation2020; Hernández-Rodríguez et al., Citation2021). A growing number of treatments appear to be becoming ineffective against Varroa, with a lack of effective alternatives available to replace them (Mitton et al., Citation2022).
Varroa was recorded for the first time in New Zealand in the year 2000 (Goodwin, Citation2004). Pyrethroids were the first treatments to be registered for use against Varroa in 2000 and 2001, followed by formamidine treatments in 2004 (Goodwin et al., Citation2005). As resistance had already been reported in numerous countries by this time, a study examining miticide efficacy on Varroa populations in New Zealand was conducted in 2003 by Goodwin et al. (Citation2005). Their study did not find sufficient evidence to conclude that mites were resistant to either flumethrin (a pyrethroid-based treatment) or amitraz (formamidine-based treatment) and beekeepers have continued to use these treatments to control Varroa.
One management tool that has proven useful in identifying the threats to honey bees has been the establishment of a survey whereby beekeepers can report colony losses each winter. Colony loss surveys were first implemented in Canada in 2003 following reports of Varroa resistance to treatments (Currie et al., Citation2010). In the years following, other regions of the world including the USA, Canada, Europe, Asia, the Middle East and Africa began conducting surveys in the wake of increased levels of overwintering colony losses (van Engelsdorp et al., Citation2008; van der Zee et al., Citation2012; Brodschneider et al., Citation2018). In 2008, a standardised survey, now known as the “COLOSS” survey, was developed to make colony loss data comparable internationally (Gray et al., Citation2022). A survey based on the COLOSS questionnaire was first conducted in New Zealand in 2015 and has been undertaken annually since (Brown et al., Citation2018). In addition to overall losses, the New Zealand survey has measured beekeepers’ attributions of losses, including to Varroa and related complications, since 2017. The annual survey also includes detailed questions on Varroa monitoring and treatment.
The aims of this study were to (1) report the role that Varroa has played in colony losses in New Zealand according to beekeepers that responded to the survey, (2) describe current Varroa management strategies practiced by commercial beekeepers and any changes in this strategy over a five-year period and (3) test for evidence of Varroa resistance to the two most commonly utilised chemical treatments in New Zealand: flumethrin and amitraz.
Materials and methods
Varroa and colony losses in New Zealand
The New Zealand Colony Loss Survey covers topics related to hive management, such as the number of colonies lost over winter, beekeepers’ attributions of losses (including Varroa and related complications) and Varroa control methods used. The current study analysed survey results for the years 2017–2021 only, as “suspected Varroa and related complications” were not included as an attributable cause of losses prior to 2017. In the 2021 survey, beekeepers were also given the opportunity to provide feedback on the perceived effectiveness of the treatments they used to combat Varroa, to give insight into the effectiveness of current treatments and to detect any early signs of developing resistance.
New Zealand beekeepers are legally obligated to register their hives under the Biosecurity Act (Citation1993), and all registered beekeepers were invited to participate in the online colony loss survey. This mandatory registration also allows for the percentage of beekeepers that participated in the survey to be estimated. In 2017, 2,066 beekeepers completed the survey, a response rate of 30.9% of all beekeepers nationwide. In the years following, the number of beekeepers that participated were 3,655 (42.3%), 3,456 (36.7%), 2,863 (32.0%) and 4,355 (49.1%) for the years 2018, 2019, 2020 and 2021, respectively (Stahlmann-Brown et al., Citation2021) (Table S1, Supplemental material). Our investigation into Varroa management strategies differed from previous work by only focusing on responses from commercial beekeepers, defined here as having more than 350 hives at the beginning of winter (according to the definition by New Zealand’s Ministry for Primary Industries (MPI) (Citation2020). These beekeepers manage the majority of hives in the country and therefore their success in controlling Varroa is of the greatest economic interest. Answers to survey questions pertaining to Varroa treatments were mandatory to complete the survey in 2017, 2020 and 2021, but not in 2018 and 2019.
Loss rates from the survey were calculated using standard approaches for estimating colony losses (van der Zee et al., Citation2013) as detailed in Stahlmann-Brown and Robertson (Citation2022). Statistical analyses were conducted in R 4.2.0 (R Development Core Team Citation2022). Overall loss rates and corresponding confidence intervals (CI) were calculated with a quasi-binomial generalised linear model and logit link function. A test of equal or given proportions (“prop.test” function in base R) determined if there was a significant change in the overall loss rates or losses attributed to Varroa over the five-year period of 2017–2021.
Testing for pesticide resistance in mites
Trials were conducted in April and May of 2022 at Victoria University of Wellington, Wellington, New Zealand. The testing protocol from Goodwin et al. (Citation2005) was followed to allow for direct comparison. Analytical standard grade flumethrin (product number 46417, CAS number 69770-45-2; Merck, New Zealand) and amitraz (product number 0506, CAS number 33089-61-1; AK Scientific, USA), were diluted in hexane (Merck, New Zealand). Flumethrin was tested at 0, 10, 20, 40, 80, 160, 320 and 640 µg/g. Amitraz was tested at 0, 2, 5, 10, 25, 50, 100, 200 and 400 µg/g (Table S2, Supplemental material). Petri dishes were prepared with the different concentrations following Goodwin et al. (Citation2005) methodology with some minor modifications, as follows. Fully refined paraffin wax (58 °C melting point, National Candles Ltd., New Zealand) was melted in a microwave and 50 mL was poured into wide-mouth, graduated bottles and weighed. Twenty-five mL of the respective pesticide concentration in hexane was added to the bottles and left in a hot water bath at 60 °C for approximately 8 h until the hexane evaporated, and each bottle returned to the original weight. The mixture of paraffin and pesticide was then swirled and poured into four 35-mm sterile petri dishes (Corning, USA) to a depth of ∼4 mm and kept in a fridge until use.
Mites were collected from hives at Victoria University of Wellington that had not been treated for Varroa for 6 months, since their purchase in September 2021. The sugar shake method was used to collect experimental mites. Approximately 300 bees were placed in a jar with a ¼ cup of icing sugar which was rolled for three minutes before being shaken over a bowl of water. Mites were retrieved from the surface of the water using a soft paintbrush and placed in a petri dish for transfer to the laboratory on-site. The freshly collected mites were counted out into groups of approximately 20 (Table S3, Supplemental material). Each group of mites was transferred to petri dishes containing the treatment (either flumethrin or amitraz of a particular concentration, or a control treatment of plain paraffin), where they were left for one hour. The mites were then transferred to a third dish of the same size, along with 2–3 bee pupae collected from the same colony as the mites and placed in an incubator at 32–34 °C, with 50% relative humidity (RH) for 48 h until the survival assessment (Figure S1, Supplemental material). We would like to note that the following minor adjustments were made to the methodology in the current study to minimize the possibility of experiencing the high variability in mite survival that was observed in the Goodwin et al. (Citation2005) study. The incubator temperature was reduced to a range of 32–34 °C as mites prefer slightly lower temperatures than the typical 35 °C of a beehive (Rosenkranz et al., Citation2010). The current study also chose to use mites collected in April-May as the natural mortality of mites in the Goodwin study during July-August was much higher compared to their trials conducted in May.
To allow for a comparison between Goodwin et al. (Citation2005) and the current study, data was analysed using the Probit regression function in IBM SPSS Statistics for MacOS, version 28 (IBM Corp., Armonk, N.Y., USA) (IBM, Citation2021). As the raw data for the Goodwin study was re-analysed using a different programme, our statistical results differ slightly from what was originally reported (Goodwin et al., Citation2005). Abbott’s correction was used to account for mite mortality in the controls for both the Goodwin et al. (Citation2005) and the 2022 datasets. The adjusted proportion of dead mites for each study was then fitted using a probit regression model on concentration (log scale). The fit of the model to the data was analysed using chi-squared goodness of fit tests for both time periods. The adjusted Lethal Concentration at 50% (LC50) and associated 95% CIs were then estimated for the flumethrin and amitraz treatments.
Investigating mutations associated with pesticide resistance in mites
We investigated Varroa samples for amino acid residue substitutions located on the Varroa destructor voltage-gated sodium channel (VGSC) gene. For example, two substitutions at nucleotide positions 1689–1691 (residue substitution M918L) (Rinkevich et al., Citation2013) and 1710–1712 (residue substitution L925V/M/I) are known to be associated with flumethrin resistance in Varroa elsewhere (González-Cabrera et al., Citation2013; González-Cabrera et al., Citation2016; Millán‐Leiva et al., Citation2021). We aligned Varroa RNA-Seq reads obtained in another study (Lester et al., Citation2022) onto the Varroa VGSC KC152655 FASTA file using HISAT 2.0 with default parameters (Kim et al., Citation2015). The resulting BAM files were visually inspected in Geneious 11.1.5 (http://www.geneious.com) to check for nucleotide polymorphisms.
The RNA-Seq analysis indicated putative substitutions around the amino acid residues M918L and L925V/M/I in the VGSC gene (Figure S2, Supplemental material) prompting us to further examine these nucleotide positions via Sanger sequencing for confirmation. We extracted DNA from 32 mites collected throughout the country, including mites from the experimental hives in Wellington (Table S4, Supplemental material). Each individual mite was placed in a 2 mL microtube (Sarstedt, Germany). Five 3.2 mm stainless steel beads (Next Advance Inc., USA), 500 µL of GENEzol DNA Plant Reagent (Geneaid Biotech, Taiwan) and 2.5 µL of β-mercaptoethanol (Sigma Aldrich, USA) were added to the tube. Samples were homogenised for one cycle of 20 s each at 8,000 rpm in a Precellys Evolution homogeniser (Bertin, France). DNA and RNA was simultaneously isolated with a 24:1 chloroform–isoamyl alcohol mixture (BioUltra, Sigma Aldrich, USA), followed by isopropanol precipitation (Sigma Aldrich, USA), and an ethanol purification step (VWR Chemicals, UK). DNA/RNA was then eluted in 15 µL of nuclease-free water (Ambion, Life Technologies, USA), quantified using a NP80 NanoPhotometer (Implen, Germany) and kept at −80 °C until use.
We designed a set of primers that amplified 615 base pairs (bp) of the VGSC gene using the Geneious Prime 2023.2.1 primer design tool, VGSC-VarroaF (AAGCCGCCATTGTTACCAGA) and VGSC-VarroaR (GAGGTCACAGGGAAGCTGAC). PCR amplifications were performed in 15 µL reactions, with 1 x MyTaq Red Mix (Meridian Bioscience, USA), 0.5 µM of each primer, 1 µL Bovine Serum Albumin (Sigma Aldrich, USA) and 2 µL of template DNA. Thermal cycling conditions were an initial denaturation at 95 °C for 5 min followed by 40 cycles of 15 sec at 95 °C, 15 sec at 59 °C and 30 sec at 72 °C, with a final 72 °C for 7 min step and a hold step at 4 °C indefinitely. PCR products were resolved by 2% agarose gel electrophoresis (89 V for 30 min, Figure S3, Supplemental material), and visualised using SYBR Safe DNA gel stain (Invitrogen/ThermoFisher Scientific, USA). Products were cleaned up using ExoSAP-IT PCR Product Cleanup Reagent (Applied Biosystems/ThermoFisher Scientific, USA) following manufacturer guidelines. Sequencing was performed on an ABI 3130 × 1 Genetic Analyzer (Applied Biosystems, USA) at Massey Genome Service (Palmerston North, New Zealand). We reconciled and aligned the forward and reverse gene sequences of the same mite using the default alignment algorithm implemented in Geneious Prime. Base calls in each sequence were manually corroborated and sequences trimmed to a total length of 555 bp. The 32 sequences were aligned to a sequence from a Varroa mite collected in the Czech Republic (GenBank accession number KC152655).
Results
Varroa and colony losses in New Zealand
The first goal of this study was to report losses attributed to Varroa based on the responses of beekeepers in the New Zealand Colony Loss Survey. Total colony loss rates have increased significantly during 2017–2021 [2 = 3622.6, df = 4, p < 0.0001], from 9.70% [95% CI: 9.36% − 10.04%] to 13.59% [95% CI: 13.23% − 14.01%] (). Among beekeepers who lost colonies in these years, the proportion of losses attributed to Varroa has also increased significantly [
2 = 8215.5, df = 4, p < 0.0001], from 16.9% [95% CI: 15.3% − 18.1%] to 38.9% [95% CI: 37.7% − 40.0%]. According to beekeepers that participated in the questionnaire survey in 2022, Varroa was the main driver of colony loss over the winter 2021. A similar trend in colony losses was observed for the subgroup of commercial beekeepers only (see Table S5, Supplemental material).
Figure 1. The proportion of total colonies lost (± 95% CI) and colonies that beekeepers suspected were lost due to Varroa (± 95% CI) for the years 2017–2021 in New Zealand. Results are based on reports from all beekeepers that participated in the annual New Zealand colony loss survey. The number of bee colonies reported on each year ranged between 242,926 and 381,148.
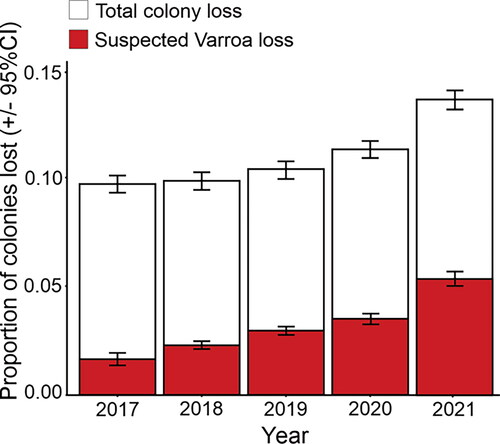
To find possible explanations for the observed increase in overall colony losses attributed to Varroa, the management strategies of commercial beekeepers were investigated. In the years 2017, 2020 and 2021, beekeepers were required to answer the question on Varroa treatments to complete the survey, therefore, the majority of participants responded to the question. In the other two years studied, however, beekeepers were able to skip the question, so only 107/170 and 90/163 commercial beekeepers reported their treatment regimes in the respective 2018 and 2019 surveys (). According to beekeepers that participated in the colony loss survey, amitraz and flumethrin were the two most commonly utilised Varroa treatments in New Zealand in each of the five years analysed. Amitraz was the most popular choice, used annually by 84–92% of commercial beekeepers over the 2017–2021 period (). Flumethrin was used annually by 68–81% of commercial beekeepers as part of their hive treatment against Varroa over that time (). The majority of commercial beekeepers used both amitraz and flumethrin in the same year (63–75%). The other common control treatments utilised were oxalic and formic acid, which are organic miticides. The use of oxalic acid has increased steadily over the five-year period, with 41.8% of beekeepers reporting its use against Varroa in 2021, whereas in 2017 it was used by only 19.7% (). Formic acid use has fluctuated year to year, with 11.2–19.1% of beekeepers applying it to hives annually.
Table 1. The most commonly utilised chemical treatments for Varroa as reported by commercial beekeepers in the annual colony loss survey in New Zealand from 2017–2021. The category “Other” contains control methods that aren’t already listed, such as thymol, fogging, drone brood removal and hyperthermia that were presented as treatment options in the New Zealand Colony Loss Survey. The number of beekeepers that answered the question on Varroa treatments is included in the table, as is the number of beekeepers who participated in the survey but chose not to answer the question.
Of the beekeepers that used amitraz treatments in the 2020/2021 season, 27.6% found the treatment to be “completely successful” against Varroa, with 64.3% finding it to be “mostly successful” (). Only 8.1% reported amitraz to be either “partly” or “not at all” successful. For flumethrin, 17.9% found the treatment to be completely successful, 63.4% thought it was mostly successful and 18.8% of beekeepers reported the pyrethroid-based control to be only partly or not at all successful in controlling Varroa (16.1% and 2.7%, respectively).
Table 2. Efficacy of flumethrin and amitraz, the two most commonly utilised chemical treatments for Varroa, as reported by commercial beekeepers in the annual colony loss survey in New Zealand in 2021. Efficacy in controlling Varroa was categorised as either “completely successful”, “mostly successful”, “partly successful” or “not at all successful”. The responses are displayed as a proportion of all commercial beekeepers that reported using that chemical. For amitraz, 121 beekeepers reported on the success of the treatment, while 101 reported on the success of flumethrin.
Testing for pesticide resistance in mites
The experiment testing for pesticide resistance in New Zealand populations of mites found a much higher concentration of flumethrin was required to kill mites compared to the concentration required in 2003 (Goodwin et al., Citation2005). Our analyses found the LC50 value for flumethrin in 2003 to be 6 µg/g [95% CI = 3 - 17] (). The chi squared test found the model to be a good fit for the data [2= 1194.2, df = 102, p < 0.001]. In 2022, the LC50 value had increased to 156 µg/g [95% CI = 115 - 219], which was also found to be a good fit [
2= 181.8, df = 30, p < 0.001]. The concentration of flumethrin required to reach an average mite mortality of 50% in 2022 was 26-fold higher compared to what was observed in 2003 (). For amitraz, the LC50 value in 2003 was 141 µg/g [95% CI = 71 - 445] with a chi-squared value of 241.0 [df = 61, p < 0.001], indicating a good fit of the model (). In 2022, the LC50 had decreased to 12 µg/g [95% CI = 10 - 16] with a chi-squared value of 65.7 [df = 30, p < 0.001].
Figure 2. Comparison of the average proportion (±1 SE) of Varroa killed at each chemical concentration in 2003 (Goodwin et al., Citation2005) and the current study for (a) flumethrin and (b) amitraz.
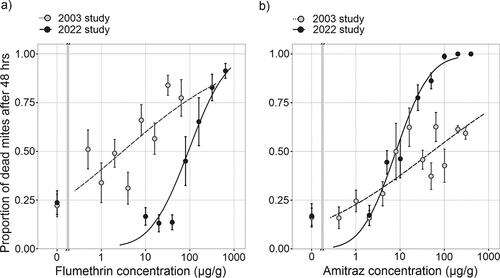
Table 3. Lethal concentrations for 50% mite mortality (LC50) with 95% confidence intervals (CI) of flumethrin and amitraz from the Goodwin study in 2003 and the current study. The results from the Goodwin study in 2003 were rerun and analysed in SPSS using an Abbott’s correction alongside the 2022 data, so differ slightly to what was reported in Goodwin et al. (Citation2005). The fit of the model was also analysed using chi-squared goodness of fit tests for both time periods.
We note that in the 2003 experiment, there was high variability in the proportion of mites that died for the flumethrin treatment. For example, at a concentration of 1 µg/g, Goodwin et al. (Citation2005) present observed mortality ranging from 0–99.7% in different replicates. This level of variability was not observed in the 2022 trial. It is also worth highlighting that the data plotted in the amitraz treatment in the 2003 experiment was unable to achieve an average mortality rate above 65% at any concentration, whereas 100% mortality was consistently achieved for the highest concentration examined in the 2022 study.
Investigating mutations associated with pesticide resistance in mites
RNA-Seq data from a previous study comprised of Varroa samples from throughout New Zealand (Lester et al., Citation2022) was examined for gene mutations associated with pesticide resistance. None of the reads that mapped to the Varroa VGSC (0–50 reads per sample, average 16.5) showed residue substitutions known to be associated with flumethrin resistance at amino acid positions M918L and L925V/M/I (Figure S2, Supplemental material) but there were potential substitutions observed in the aligned reads in this portion of the gene (Figure S3, Supplemental material), which we investigated further via Sanger sequencing of additional samples. The VGSC gene of 32 mites from across New Zealand presented no evidence of mutations in either amino acid residues M918L or L925V/M/I, or elsewhere in the 555 bp of amplified DNA using Sanger sequencing. All 32 mites were homozygous for the stretch of sequence analysed and identical to the Czech Republic mite retrieved from GenBank (sequences are archived in GenBank under accession numbers OR509455–OR509486, Table S4, Supplemental material).
Discussion
The role of Varroa in colony loss
Colony loss rates over winter rose significantly in New Zealand between 2017 and 2021. During this same time period, attributions of losses to Varroa increased sharply, with beekeepers reporting this parasite to be the biggest driver of colony loss during winter 2021. These findings are unsurprising as Varroa has also been found to be the main cause of winter colony losses for the United States (Kulhanek et al., Citation2017; Steinhauer et al., Citation2021) and a major cause of colony losses in Europe, South Africa and Canada (Clermont et al., Citation2014; Pirk et al., Citation2014; Morawetz et al., Citation2019; Ferland et al., Citation2021). As honey exports are of great economic value in New Zealand, worth $482 million in 2021 (Stahlmann-Brown et al., Citation2022b), it would be beneficial for the apiculture industry to better understand why colony losses to Varroa have increased. Whilst the majority of commercial beekeepers were satisfied with the efficacy of flumethrin, approximately 19% of beekeepers in the survey indicated that flumethrin had failed to successfully control Varroa in their hives. Failure to control Varroa is consistent with emerging miticide resistance, although there are other potential mechanisms for miticide failure. Stahlmann-Brown and Robertson (Citation2022), for example, report that significantly underdosing flumethrin was a common practice by New Zealand beekeepers during the 2020–2021 season. Even so, some commercial beekeepers communicated with the study authors that they felt flumethrin had become less effective in recent years; many of these beekeepers reported that they followed flumethrin treatments with other treatments such as oxalic acid. Indeed, treatment with oxalic acid has risen during the study period, and it is noted that oxalic acid can be applied during the honey flow if a spring treatment fails. Oxalic acid may often not be ideal either, however, as sub-lethal stress effects to honey bees have been reported (Gunes et al., Citation2017; Rademacher et al., Citation2017).
Pesticide resistance in mites and associated mutations
Our experiments testing for flumethrin resistance in New Zealand Varroa populations suggest resistance is developing when compared to the study conducted by Goodwin et al. (Citation2005). The LC50 for flumethrin (156 µg/g) was found to be 26 times higher (6 µg/g) than in 2003. Issues with the variability of results from 2003, however, mean that a degree of caution is needed when drawing comparisons between the two studies. There was far greater variation in mite mortality in the 2003 study than was observed in 2022, particularly in the replicates for the lower concentrations of flumethrin. Preliminary data for the 2022 study found subtle differences in temperature and humidity affected mortality, which is a possible explanation for the variation observed in the 2003 study. In the 2022 trials, mortality rates were consistent throughout. Our consistent results make the current study a good baseline for comparison in future research testing the sensitivity of Varroa to flumethrin and amitraz in New Zealand.
The methodology used by Goodwin et al. (Citation2005) was based on research conducted by Milani (Citation1995) assessing the susceptibility of Varroa to flumethrin, fluvalinate and acrinathrin in Italy. This study also dissolved chemicals into paraffin wax, making their results comparable to the current study. For the flumethrin trials, the LC50 values observed for two non-resistant mite populations in the Italian study were 0.28 µg/g and 0.36 µg/g, substantially lower than the LC50 of 156 µg/g we observed in 2022. Additionally, the LC50 values in our current study were seven times greater than the LC50 values (11.4 µg/g and 20.5 µg/g) for the two flumethrin-resistant Varroa populations in Italy (Milani, Citation1995). This difference further suggests that Varroa populations in New Zealand are likely to have developed a degree of resistance to flumethrin, although further research involving the use of a known susceptible population of Varroa is needed to test this hypothesis.
In contrast to the flumethrin results, trials assessing the efficacy of amitraz found no evidence of mites developing resistance since 2003. In fact, the estimated LC50 value for amitraz in the current study (12 µg/g) was much lower than in 2003 (141 µg/g). The apparent drop in LC50 value is likely not due to mites becoming more susceptible to the treatment but was likely due to issues with the 2003 trials. In 2003 the average mite mortality represented in the figure did not exceed 65%, even for the highest concentration of 300 µg/g, which should have achieved 100% mortality as it far exceeds the maximum concentration needed to achieve this in other studies (Thompson et al., Citation2002; Maggi et al., Citation2008). At the time, it was suggested that this unexpected result was due to the chemical not being sufficiently mixed into the wax. It may be possible that the mites in Goodwin et al. (Citation2005) had inconsistent exposure to the pesticide, or that there were other methodological issues with their setup. Their study, however, represents the best available data we have for comparison. Whilst there is some question about the amitraz results in 2003, the LC50 in 2022 suggests that amitraz is at least as effective as it was, so we are less concerned about the efficacy of this pesticide than we are for flumethrin.
A direct comparison of our amitraz results to other research is limited, as the experiment conducted by Milani (Citation1995) did not test for amitraz resistance, and other published studies of resistance to this chemical used different methodologies such as direct exposure of the mites to treatment strips or vials of evaporated solution rather than amitraz-impregnated paraffin wax (Milani, Citation1995, Thompson et al., Citation2002, Maggi et al., Citation2008). This difference prevents us from drawing comparisons to other findings, but the current study establishes a baseline of amitraz susceptibility in New Zealand Varroa populations for future research. Examining the feedback from beekeepers that completed the New Zealand Colony Loss Survey, ∼28% found amitraz to be completely successful in treating Varroa, and less than 10% found amitraz to be partly successful or not at all successful. Amitraz was thus considered more effective than flumethrin by New Zealand’s commercial beekeepers. It remains possible that undetected resistance to amitraz is developing in New Zealand mite populations as there has been evidence of resistance in other countries (Kamler et al., Citation2016; Almecija et al., Citation2020; Rinkevich, Citation2020). Yet, for the most part, amitraz seems to still be a popular and effective treatment against Varroa in many countries, even after decades of use (Ferland et al., Citation2021; Hernández-Rodríguez et al., Citation2021).
Molecular analyses conducted on the pyrethroid-susceptible sodium channel gene in Varroa found no evidence of mutations known to be associated with flumethrin resistance. This result was surprising as numerous studies on Varroa populations with known resistance to pyrethroids have been observed to possess mutations within this gene (González-Cabrera et al., Citation2013; González-Cabrera et al., Citation2018). Some authors hypothesize that the resistance of Varroa to pyrethroids has only evolved once or twice, initially arising in mite populations from Italy before dispersing to other regions via the movement of bee colonies (Martin, Citation2004; Mitton et al., Citation2022). Mitochondrial gene analysis of Varroa indicate only one introduction of this parasite into New Zealand (Lester et al., Citation2022). It is therefore likely that the Varroa introduced to New Zealand did not already possess known pyrethroid-resistant mutations. Varroa in New Zealand may, however, exhibit novel mutations that would similarly confer flumethrin resistance. Indeed, a new mutation was detected recently at position 918 by Millán-Leiva et al. (Citation2021) in Varroa samples from Spain, so it is not unreasonable to suggest selection pressures could give rise to novel mutations in New Zealand populations. In addition to point mutations, various alternative mechanisms could also account for the development of pyrethroid resistance in mites, such as heightened enzymatic activity. Esterase enzymes, for example, have been hypothesised to be important for detoxification processes. Increased levels of esterase activity have been linked to the resistance of Varroa against flumethrin (Sammataro et al., Citation2005). Although we were unable to identify the cause, and further research is needed, the findings of our trials in conjunction with reports from beekeepers suggest mites may be developing resistance to one of the most popular Varroa treatments in New Zealand.
Future directions
It is important to detect and mitigate miticide resistance early, as resistant genes are able to spread swiftly through mite populations due to haplo-diploid sex determination and the high level of inbreeding involved in Varroa reproduction (Beaurepaire et al., Citation2017; González-Cabrera et al., Citation2018). The ability of Varroa to rapidly develop resistance to chemical treatments highlights how crucial it is to investigate new control strategies. An additional motivator for new management approaches is the detrimental effects miticides have on the honey bees themselves (Tihelka, Citation2018). One new strategy currently being investigated is the breeding of Varroa-resistant traits in honey bees, such as hygienic behaviour, grooming and shorter brood development times (Spivak & Gilliam, Citation1998; Mondet et al., Citation2020; van Alphen & Fernhout, Citation2020). These approaches may be more sustainable than pesticides as they are not detrimental to bees or the environment. There have been challenges in attempts to maintain mite resistant traits within bee populations though, due to the heritability of these traits, genetic variability within hives, and a poor understanding of the combination of traits required to achieve natural resistance (Mondet et al., Citation2020). Another strategy currently under development that shows more promise is the utilisation of RNA interference technology (RNAi) against Varroa (Garbian et al., Citation2012). This method has been observed to reduce mite populations (Garbian et al., Citation2012; Huang et al., Citation2019) and is thought to be species-specific to Varroa, likely making it harmless to honey bees and other non-target species (Tan et al., Citation2016; Krishnan et al., Citation2021).
Current management strategies are providing a degree of protection for honey bee populations. There is, however, a need for resistance management to ensure chemicals including flumethrin remain effective (Lester, Citation2023). Alternating mite control treatments might help prevent the development of resistance and is a management strategy that the majority of commercial beekeepers in New Zealand utilise, according to the findings of our study. There is still concern that not all beekeepers are practicing correct resistance management, as 13% of beekeepers that participated in the 2021 survey (which included hobbyists) reported solely using flumethrin to treat for Varroa (Stahlmann-Brown et al., Citation2022a). The ability of mites to develop resistance to chemical treatments highlights the need for more effective Varroa control methods to protect honey bees, and to help prevent severe economic losses and threats to food security globally.
Supplementary material.docx
Download MS Word (2.6 MB)Disclosure statement
The authors report there are no competing interests to declare.
Data availability statement
Data from the New Zealand Colony Loss Survey are not publicly available due to privacy concerns and potential commercial sensitivities. Data from the experiments testing for chemical resistance presented in this study are available on request from the corresponding author.
Additional information
Funding
References
- Aizen, M. A., Aguiar, S., Biesmeijer, J. C., Garibaldi, L. A., Inouye, D. W., Jung, C., Martins, D. J., Medel, R., Morales, C. L., Ngo, H., Pauw, A., Paxton, R. J., Sáez, A., & Seymour, C. L. (2019). Global agricultural productivity is threatened by increasing pollinator dependence without a parallel increase in crop diversification. Global Change Biology, 25(10), 3516–3527. https://doi.org/10.1111/gcb.14736
- Aizen, M. A., & Harder, L. D. (2009). The global stock of domesticated honey bees is growing slower than agricultural demand for pollination. Current Biology, 19(11), 915–918. https://doi.org/10.1016/j.cub.2009.03.071
- Almecija, G., Poirot, B., Cochard, P., & Suppo, C. (2020). Inventory of Varroa destructor susceptibility to amitraz and tau-fluvalinate in France. Experimental & Applied Acarology, 82(1), 1–16. https://doi.org/10.1007/s10493-020-00535-w
- Amdam, G. V., Hartfelder, K., Norberg, K., Hagen, A., & Omholt, S. W. (2004). Altered physiology in worker honey bees (Hymenoptera: Apidae) infested with the mite Varroa destructor (Acari: Varroidae): A factor in colony loss during overwintering? Journal of Economic Entomology, 97(3), 741–747. https://doi.org/10.1093/jee/97.3.741
- Beaurepaire, A. L., Krieger, K. J., & Moritz, R. F. (2017). Seasonal cycle of inbreeding and recombination of the parasitic mite Varroa destructor in honey bee colonies and its implications for the selection of acaricide resistance. Infection, Genetics and Evolution, 50, 49–54. https://doi.org/10.1016/j.meegid.2017.02.011
- Biosecurity Act (1993). New Zealand Legislation, Biosecurity Act 1993. Ministry for Primary Industries.
- Breeze, T. D., Vaissière, B. E., Bommarco, R., Petanidou, T., Seraphides, N., Kozák, L., Scheper, J., Biesmeijer, J. C., Kleijn, D., Gyldenkærne, S., Moretti, M., Holzschuh, A., Steffan-Dewenter, I., Stout, J. C., Pärtel, M., Zobel, M., & Potts, S. G. (2014). Agricultural policies exacerbate honey bee pollination service supply-demand mismatches across Europe. PloS One, 9(1), e82996. https://doi.org/10.1371/journal.pone.0082996
- Brodschneider, R., Gray, A., Adjlane, N., Ballis, A., Brusbardis, V., Charrière, J.-D., Chlebo, R., Coffey, M. F., Dahle, B., de Graaf, D. C., Maja Dražić, M., Evans, G., Fedoriak, M., Forsythe, I., Gregorc, A., Grzęda, U., Hetzroni, A., Kauko, L., Kristiansen, P., … Danihlík, J. (2018). Multi-country loss rates of honey bee colonies during winter 2016/2017 from the COLOSS survey. Journal of Apicultural Research, 57(3), 452–457. https://doi.org/10.1080/00218839.2018.1460911
- Brown, P., Newstrom-Lloyd, L. E., Foster, B. J., Badger, P. H., & McLean, J. A. (2018). Winter 2016 honey bee colony losses in New Zealand. Journal of Apicultural Research, 57(2), 278–291. https://doi.org/10.1080/00218839.2018.1430980
- Clermont, A., Eickermann, M., Kraus, F., Georges, C., Hoffmann, L., & Beyer, M. (2014). A survey on some factors potentially affecting losses of managed honey bee colonies in Luxembourg over the winters 2010/2011 and 2011/2012. Journal of Apicultural Research, 53(1), 43–56. https://doi.org/10.3896/IBRA.1.53.1.04
- Currie, R. W., Pernal, S. F., & Guzmán-Novoa, E. (2010). Honey bee colony losses in Canada. Journal of Apicultural Research, 49(1), 104–106. https://doi.org/10.3896/IBRA.1.49.1.18
- Dainat, B., Evans, J. D., Chen, Y. P., Gauthier, L., & Neumann, P. (2012). Predictive markers of honey bee colony collapse. PloS One, 7(2), e32151. https://doi.org/10.1371/journal.pone.0032151
- Dekeyser, M. A., & Downer, R. G. (1994). Biochemical and physiological targets for miticides. Pesticide Science, 40(2), 85–101. https://doi.org/10.1002/ps.2780400202
- Di Prisco, G., Annoscia, D., Margiotta, M., Ferrara, R., Varricchio, P., Zanni, V., Caprio, E., Nazzi, F., & Pennacchio, F. (2016). A mutualistic symbiosis between a parasitic mite and a pathogenic virus undermines honey bee immunity and health. Proceedings of the National Academy of Sciences of the United States of America, 113(12), 3203–3208.
- Dolezal, A. G., Carrillo-Tripp, J., Judd, T. M., Allen Miller, W., Bonning, B. C., & Toth, A. L. (2019). Interacting stressors matter: Diet quality and virus infection in honey bee health. Royal Society Open Science, 6(2), 181803. https://doi.org/10.1098/rsos.181803
- Eilers, E. J., Kremen, C., Smith Greenleaf, S., Garber, A. K., & Klein, A.-M. (2011). Contribution of pollinator-mediated crops to nutrients in the human food supply. PloS One, 6(6), e21363. https://doi.org/10.1371/journal.pone.0021363
- Ferland, J., Nasr, M., Wilson, G., Jordan, C., Kempers, M., Kozak, P., Lafreniere, R., Maund, C., Pernal, S., Sproule, J. (2021). Canadian Association of Professional Apiculturists statement on honey bee wintering losses in Canada (2021). https://capabees.com/shared/CAPA-Statement-on-Colony-Losses-2021-2022-fv.
- Francis, R. M., Nielsen, S. L., & Kryger, P. (2013). Varroa-virus interaction in collapsing honey bee colonies. PloS One, 8(3), e57540. https://doi.org/10.1371/journal.pone.0057540
- Garbian, Y., Maori, E., Kalev, H., Shafir, S., & Sela, I. (2012). Bidirectional transfer of RNAi between honey bee and Varroa destructor: Varroa gene silencing reduces Varroa population. PLoS Pathogens, 8(12), e1003035. https://doi.org/10.1371/journal.ppat.1003035
- González-Cabrera, J., Bumann, H., Rodríguez-Vargas, S., Kennedy, P. J., Krieger, K., Altreuther, G., Hertel, A., Hertlein, G., Nauen, R., & Williamson, M. S. (2018). A single mutation is driving resistance to pyrethroids in European populations of the parasitic mite, Varroa destructor. Journal of Pest Science, 91(3), 1137–1144. https://doi.org/10.1007/s10340-018-0968-y
- González-Cabrera, J., Davies, T. E., Field, L. M., Kennedy, P. J., & Williamson, M. S. (2013). An amino acid substitution (L925V) associated with resistance to pyrethroids in Varroa destructor. PloS One, 8(12), e82941. https://doi.org/10.1371/journal.pone.0082941
- González-Cabrera, J., Rodríguez-Vargas, S., Davies, T. E., Field, L. M., Schmehl, D., Ellis, J. D., Krieger, K., & Williamson, M. S. (2016). Novel mutations in the voltage-gated sodium channel of pyrethroid-resistant Varroa destructor populations from the Southeastern USA. PloS One, 11(5), e0155332. https://doi.org/10.1371/journal.pone.0155332
- Goodwin, R. M. (2004). Introduction and spread of Varroa in New Zealand. Bee World, 85(2), 26–28. https://doi.org/10.1080/0005772X.2004.11099614
- Goodwin, R., Taylor, M., McBrydie, H., & Cox, H. (2005). Base levels of resistance to common control compounds by a New Zealand population of Varroa destructor. New Zealand Journal of Crop and Horticultural Science, 33(4), 347–352. https://doi.org/10.1080/01140671.2005.9514369
- Goulson, D., Nicholls, E., Botías, C., & Rotheray, E. L. (2015). Bee declines driven by combined stress from parasites, pesticides, and lack of flowers. Science (New York, N.Y.), 347(6229), 1255957. https://doi.org/10.1126/science.1255957
- Gray, A., Adjlane, N., Arab, A., Ballis, A., Brusbardis, V., Bugeja Douglas, A., Cadahía, L., Charrière, J.-D., Chlebo, R., Coffey, M. F., Cornelissen, B., Costa, C. A. D., Danneels, E., Danihlík, J., Dobrescu, C., Evans, G., Fedoriak, M., Forsythe, I., Gregorc, A., … Brodschneider, R. (2022). Honey bee colony loss rates in 37 countries using the COLOSS survey for winter 2019–2020: The combined effects of operation size, migration and queen replacement. Journal of Apicultural Research, 62(2), 204–210. https://doi.org/10.1080/00218839.2022.2113329
- Gunes, N., Aydın, L., Belenli, D., Hranitz, J. M., Mengilig, S., & Selova, S. (2017). Stress responses of honey bees to organic acid and essential oil treatments against Varroa mites. Journal of Apicultural Research, 56(2), 175–181. https://doi.org/10.1080/00218839.2017.1291229
- Han, B., Wu, J., Wei, Q., Liu, F., Cui, L., Rueppell, O., & Xu, S. (2024). Life-history stage determines the diet of ectoparasitic mites on their honey bee hosts. Nature Communications, 15(1), 725. https://doi.org/10.1038/s41467-024-44915-x
- Hernández-Rodríguez, C. S., Marín, Ó., Calatayud, F., Mahiques, M. J., Mompó, A., Segura, I., Simó, E., & González-Cabrera, J. (2021). Large-scale monitoring of resistance to coumaphos, amitraz, and pyrethroids in Varroa destructor. Insects, 12(1), 27. https://doi.org/10.3390/insects12010027
- Higes, M., Martín-Hernández, R., Hernández-Rodríguez, C. S., & González-Cabrera, J. (2020). Assessing the resistance to acaricides in Varroa destructor from several Spanish locations. Parasitology Research, 119(11), 3595–3601. https://doi.org/10.1007/s00436-020-06879-x
- Huang, Z. Y., Bian, G., Xi, Z., & Xie, X. (2019). Genes important for survival or reproduction in Varroa destructor identified by RNAi. Insect Science, 26(1), 68–75. https://doi.org/10.1111/1744-7917.12513
- IBM (2021). What’s New in SPSS Statistics 28. https://community.ibm.com/community/user/ai-datascience/blogs/brent-gardiner/2021/05/24/whats-new-in-spss-statistics-28.
- Jack, C. J., & Ellis, J. D. (2021). Integrated pest management control of Varroa destructor (Acari: Varroidae), the most damaging pest of (Apis mellifera L. (Hymenoptera: Apidae)) colonies. Journal of Insect Science (Online), 21(5), 6. https://doi.org/10.1093/jisesa/ieab058
- Kamler, M., Nesvorna, M., Stara, J., Erban, T., & Hubert, J. (2016). Comparison of tau-fluvalinate, acrinathrin, and amitraz effects on susceptible and resistant populations of Varroa destructor in a vial test. Experimental & Applied Acarology, 69(1), 1–9. https://doi.org/10.1007/s10493-016-0023-8
- Kim, D., Langmead, B., & Salzberg, S. L. (2015). HISAT: A fast spliced aligner with low memory requirements. Nature Methods, 12(4), 357–360. https://doi.org/10.1038/nmeth.3317
- Krishnan, N., Hall, M. J., Hellmich, R. L., Coats, J. R., & Bradbury, S. P. (2021). Evaluating toxicity of Varroa mite (Varroa destructor)-active dsRNA to monarch butterfly (Danaus plexippus) larvae. PloS One, 16(6), e0251884. https://doi.org/10.1371/journal.pone.0251884
- Kulhanek, K., Steinhauer, N., Rennich, K., Caron, D. M., Sagili, R. R., Pettis, J. S., Ellis, J. D., Wilson, M. E., Wilkes, J. T., Tarpy, D. R., Rose, R., Lee, K., Rangel, J., & vanEngelsdorp, D. (2017). A national survey of managed honey bee 2015–2016 annual colony losses in the USA. Journal of Apicultural Research, 56(4), 328–340. https://doi.org/10.1080/00218839.2017.1344496
- Lester, P. J. (2023). Integrated resistance management for acaricide use on Varroa destructor. Frontiers in Bee Science, 1, 1297326. https://doi.org/10.3389/frbee.2023.1297326
- Lester, P. J., Felden, A., Baty, J. W., Bulgarella, M., Haywood, J., Mortensen, A. N., Remnant, E. J., & Smeele, Z. E. (2022). Viral communities in the parasite Varroa destructor and in colonies of their honey bee host (Apis mellifera) in New Zealand. Scientific Reports, 12(1), 8809. https://doi.org/10.1038/s41598-022-12888-w
- Maggi, M. D., Ruffinengo, S. R., Gende, L. B., Eguaras, M. J., & Sardella, N. H. (2008). LC50 baseline levels of amitraz, coumaphos, fluvalinate and flumethrin in populations of Varroa destructor from Buenos Aires Province, Argentina. Journal of Apicultural Research, 47(4), 292–295. https://doi.org/10.1080/00218839.2008.11101477
- Martin, S. J. (2004). Acaricide (pyrethroid) resistance in Varroa destructor. Bee World, 85(4), 67–69. https://doi.org/10.1080/0005772X.2004.11099632
- Milani, N. (1995). The resistance of Varroa jacobsoni Oud to pyrethroids: A laboratory assay. Apidologie, 26(5), 415–429. https://doi.org/10.1051/apido:19950507
- Millán‐Leiva, A., Marín, Ó., Christmon, K., vanEngelsdorp, D., & González‐Cabrera, J. (2021). Mutations associated with pyrethroid resistance in Varroa mite, a parasite of honey bees, are widespread across the United States. Pest Management Science, 77(7), 3241–3249. https://doi.org/10.1002/ps.6366
- Millán-Leiva, A., Marín, Ó., De la Rúa, P., Muñoz, I., Tsagkarakou, A., Eversol, H., Christmon, K., vanEngelsdorp, D., & González-Cabrera, J. (2021). Mutations associated with pyrethroid resistance in the honey bee parasite Varroa destructor evolved as a series of parallel and sequential events. Journal of Pest Science, 94(4), 1505–1517. https://doi.org/10.1007/s10340-020-01321-8
- Ministry for Primary Industries (MPI). (2020). Ministry for Primary Industries 2020 apiculture monitoring programme. https://www.mpi.govt.nz/dmsdocument/44068-Apiculture-Moniotoring-Report-2020.
- Mitton, G. A., Meroi Arcerito, F., Cooley, H., Fernández de Landa, G., Eguaras, M. J., Ruffinengo, S. R., & Maggi, M. D. (2022). More than sixty years living with Varroa destructor: A review of acaricide resistance. International Journal of Pest Management, 68, 1–18. https://doi.org/10.1080/09670874.2022.2094489
- Mondet, F., Beaurepaire, A., McAfee, A., Locke, B., Alaux, C., Blanchard, S., Danka, B., & Le Conte, Y. (2020). Honey bee survival mechanisms against the parasite Varroa destructor: A systematic review of phenotypic and genomic research efforts. International Journal for Parasitology, 50(6-7), 433–447. https://doi.org/10.1016/j.ijpara.2020.03.005
- Morawetz, L., Köglberger, H., Griesbacher, A., Derakhshifar, I., Crailsheim, K., Brodschneider, R., & Moosbeckhofer, R. (2019). Health status of honey bee colonies (Apis mellifera) and disease-related risk factors for colony losses in Austria. PloS One, 14(7), e0219293. https://doi.org/10.1371/journal.pone.0219293
- Murphy, J. T., Breeze, T. D., Willcox, B., Kavanagh, S., & Stout, J. C. (2022). Globalisation and pollinators: Pollinator declines are an economic threat to global food systems. People and Nature, 4(3), 773–785. https://doi.org/10.1002/pan3.10314
- Narahashi, T. (2000). Neuroreceptors and ion channels as the basis for drug action: Past, present, and future. Journal of Pharmacology and Experimental Therapeutics, 294(1), 1–26.
- Pirk, C. W., Human, H., Crewe, R. M., & VanEngelsdorp, D. (2014). A survey of managed honey bee colony losses in the Republic of South Africa–2009 to 2011. Journal of Apicultural Research, 53(1), 35–42. https://doi.org/10.3896/IBRA.1.53.1.03
- R Development Core Team, (2022). R: A language and environment for statistical computing computer program, version By R Development Core Team, Vienna, Austria.
- Rademacher, E., Harz, M., & Schneider, S. (2017). Effects of oxalic acid on Apis mellifera (Hymenoptera: Apidae). Insects, 8(3), 84. https://doi.org/10.3390/insects8030084
- Ramsey, S. D., Ochoa, R., Bauchan, G., Gulbronson, C., Mowery, J. D., Cohen, A., Lim, D., Joklik, J., Cicero, J. M., & Ellis, J. D. (2019). Varroa destructor feeds primarily on honey bee fat body tissue and not hemolymph. Proceedings of the National Academy of Sciences of the United States of America, 116(5), 1792–1801.
- Reilly, J. R., Artz, D. R., Biddinger, D., Bobiwash, K., Boyle, N. K., Brittain, C., Brokaw, J., Campbell, J. W., Daniels, J., Elle, E., Ellis, J. D., Fleischer, S. J., Gibbs, J., Gillespie, R. L., Gundersen, K. B., Gut, L., Hoffman, G., Joshi, N., Lundin, O., … Winfree, R. (2020). Crop production in the USA is frequently limited by a lack of pollinators. Proceedings of the Royal Society B: Biological Sciences, 287(1931), 20200922. https://doi.org/10.1098/rspb.2020.0922
- Rinkevich, F. D. (2020). Detection of amitraz resistance and reduced treatment efficacy in the Varroa mite, Varroa destructor, within commercial beekeeping operations. PloS One, 15(1), e0227264. https://doi.org/10.1371/journal.pone.0227264
- Rinkevich, F. D., Du, Y., & Dong, K. (2013). Diversity and convergence of sodium channel mutations involved in resistance to pyrethroids. Pesticide Biochemistry and Physiology, 106(3), 93–100. https://doi.org/10.1016/j.pestbp.2013.02.007
- Rosenkranz, P., Aumeier, P., & Ziegelmann, B. (2010). Biology and control of Varroa destructor. Journal of Invertebrate Pathology, 103 (Suppl 1), S96–S119. https://doi.org/10.1016/j.jip.2009.07.016
- Sammataro, D., Untalan, P., Guerrero, F., & Finley, J. (2005). The resistance of Varroa mites (Acari: Varroidae) to acaricides and the presence of esterase. International Journal of Acarology, 31(1), 67–74. https://doi.org/10.1080/01647950508684419
- Spivak, M., & Gilliam, M. (1998). Hygienic behaviour of honey bees and its application for control of brood diseases and Varroa: Part II. Studies on hygienic behaviour since the Rothenbuhler era. Bee World, 79(4), 169–186. https://doi.org/10.1080/0005772X.1998.11099408
- Stahlmann-Brown, P., Hall, R. J., Butt, R., McCall, B., Torres, G., & Wright, T. (2022b). Valuing over-winter colony losses for New Zealand’s commercial beekeepers. New Zealand Economic Papers, 57(2), 184–190. https://doi.org/10.1080/00779954.2022.2146527
- Stahlmann-Brown, P., Hall, R. J., Pragert, H., & Robertson, T. (2022a). Varroa appears to drive persistent increases in New Zealand colony losses. Insects, 13(7), 589. https://doi.org/10.3390/insects13070589
- Stahlmann-Brown, P., Robertson, T. (2022). New Zealand Colony Loss Survey Report – 2021. https://www.mpi.govt.nz/dmsdocument/50221-Report-on-the-2021-New-Zealand-Colony-Loss-Survey.
- Stahlmann-Brown, P., Robertson, T., Borowik, O. (2021). Report on the 2020 New Zealand colony loss survey. https://www.mpi.govt.nz/dmsdocument/44590-Report-on-the-2020-New-Zealand-Colony-Loss-Survey.
- Steinhauer, N., Aurell, D., Bruckner, S., Wilson, M., Rennich, K., vanEngelsdorp, D., Williams, G. (2021). United States honey bee colony losses 2020–2021. https://beeinformed.org/2021/06/21/united-states-honey-bee-colony-losses-2020-2021-preliminary-results.
- Tan, J., Levine, S. L., Bachman, P. M., Jensen, P. D., Mueller, G. M., Uffman, J. P., Meng, C., Song, Z., Richards, K. B., & Beevers, M. H. (2016). No impact of DvSnf7 RNA on honey bee (Apis mellifera L.) adults and larvae in dietary feeding tests. Environmental Toxicology and Chemistry, 35(2), 287–294. https://doi.org/10.1002/etc.3075
- Thompson, H. M., Brown, M. A., Ball, R. F., & Bew, M. H. (2002). First report of Varroa destructor resistance to pyrethroids in the UK. Apidologie, 33(4), 357–366. https://doi.org/10.1051/apido:2002027
- Tihelka, E. (2018). Effects of synthetic and organic acaricides on honey bee health: A review. Slovenian Veterinary Research, 55(3), 119–140. https://doi.org/10.26873/SVR-422-2017
- Traynor, K. S., Mondet, F., de Miranda, J. R., Techer, M., Kowallik, V., Oddie, M. A. Y., Chantawannakul, P., & McAfee, A. (2020). Varroa destructor: A complex parasite, crippling honey bees worldwide. Trends in Parasitology, 36(7), 592–606. https://doi.org/10.1016/j.pt.2020.04.004
- van Alphen, J. J., & Fernhout, B. J. (2020). Natural selection, selective breeding, and the evolution of resistance of honey bees (Apis mellifera) against Varroa. Zoological Letters, 6(6), 6. https://doi.org/10.1186/s40851-020-00158-4
- van der Zee, R., Gray, A., Holzmann, C., Pisa, L., Brodschneider, R., Chlebo, R., Coffey, M. F., Kence, A., Kristiansen, P., Mutinelli, F., Nguyen, B. K., Noureddine, A., Peterson, M., Soroker, V., Topolska, G., Vejsnæs, F., & Wilkins, S. (2013). Standard survey methods for estimating colony losses and explanatory risk factors in Apis mellifera. Journal of Apicultural Research, 52(4), 1–36. https://doi.org/10.3896/IBRA.1.52.4.18
- van der Zee, R., Pisa, L., Andonov, S., Brodschneider, R., Charrière, J.-D., Chlebo, R., Coffey, M. F., Crailsheim, K., Dahle, B., Gajda, A., Gray, A., Drazic, M. M., Higes, M., Kauko, L., Kence, A., Kence, M., Kezic, N., Kiprijanovska, H., Kralj, J., … Wilkins, S. (2012). Managed honey bee colony losses in Canada, China, Europe, Israel and Turkey, for the winters of 2008–9 and 2009–10. Journal of Apicultural Research, 51(1), 100–114. https://doi.org/10.3896/IBRA.1.51.1.12
- van Engelsdorp, D., Hayes, J., Underwood, R. M., & Pettis, J. (2008). A survey of honey bee colony losses in the US, fall 2007 to spring 2008. PloS One, 3(12), e4071. https://doi.org/10.1371/journal.pone.0004071
- Vanbergen, A. J., & Pollinators Initiative, I. (2013). Threats to an ecosystem service: Pressures on pollinators. Frontiers in Ecology and the Environment, 11(5), 251–259. https://doi.org/10.1890/120126
- Wang, R., Huang, Z. Y., & Dong, K. (2003). Molecular characterization of an arachnid sodium channel gene from the Varroa mite (Varroa destructor). Insect Biochemistry and Molecular Biology, 33(7), 733–739. https://doi.org/10.1016/s0965-1748(03)00068-7
- Wilfert, L., Long, G., Leggett, H. C., Schmid-Hempel, P., Butlin, R., Martin, S. J. M., & Boots, M. (2016). Deformed wing virus is a recent global epidemic in honey bees driven by Varroa mites. Science (New York, N.Y.), 351(6273), 594–597. https://doi.org/10.1126/science.aac9976