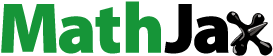
Abstract
In this study, the pollen analysis, physicochemical and volatile profiles of blong song, bidens, coffee, and mint honeys from Vietnam were analyzed. The primary pollen content was 87.0%, 37.5%, 5.7%, and 2.7% in the blong song, coffee, bidens, and mint honey, respectively. The average values of the physicochemical properties ranged from 9.4 to 33.5 mS m−1 for electrical conductivity, from 9.5 to 19.7 meq kg−1 for free acidity, from <2 to 10.7 DN for diastase activity, from 0 to 44.7 mm Pfund for color, and from 2.9 to 39.2 mg kg−1 for 5-hydroxymethyl-2-furfural (5-HMF) showed statistically significant differences (p < 0.05) among the four types of honey. Principal component analysis (PCA) of sugars revealed that trehalose was typical for blong song honey; melibiose, sucrose, and maltose for bidens honey; and turanose for mint honey. Using headspace solid phase microextraction coupled with gas chromatography-mass spectrometry (HS-SPME/GC-MS) several specific volatile compounds for each honey type were identified: linalool oxide isomers for blong song honey, acetic acid phenylethyl ester for bidens honey, citral, nonanoic acid, α-terpineol, 1-nonanol, and benzeneacetaldehyde for coffee honey and menthol isomers, and isomethyl acetate for mint honey. The results provide comprehensive data on chemometrics, melissopalynological profiles, and volatile compounds analysis for the classification of honey with different floral origins from Vietnam.
Introduction
Honey is a natural sweet substance produced by honey bees from the nectar of plants that has many health benefits (Matkovits et al., Citation2023). It contains a high level of carbohydrates (75%–80%), which are predominantly glucose and fructose (Grabato et al., Citation2022). Furthermore, honey also consists of other nutrients and bioactive compounds, including proteins, amino acids, vitamins, minerals, flavonoids, phenolic acids, and enzymes (Pauliuc et al., Citation2022). Depending on the floral source, honey can generally be classified as monofloral and multifloral honey. Specific honey is considered monofloral honey when it is derived primarily from a single source of nectar, while multifloral honey, instead, contains a mixture of nectars from different plant species (Chen et al., Citation2019; Rehman & Majid, Citation2020). Monofloral honey is often chosen by consumers because of its specific aroma, refined and unique taste, and pharmacological properties (Soares et al., Citation2017). Due to higher market values, monofloral honey may be adulterated with multifloral honey, inferior quality honey and other substances or mislabeled (Chiara et al., Citation2021; Pauliuc et al., Citation2022). Therefore, the botanical origin must be identified based on reliable methodologies and chemical markers to protect consumers from fraudulent and low-quality products (Bueno-Costa et al., Citation2016; Ruoff & Bogdanov, Citation2004).
Honey can be authenticated by tracing its botanical and/or geographical origin and by examining its component profile, including carbohydrates, proteins, organic acids, amino acids, minerals, and volatile compounds (Mureșan et al., Citation2022). Melissopalynology is the conventional approach to identifying the botanical origin of honey by observing pollen grains in honey under a microscope (Soares et al., Citation2017). Pollen grains in the sediment are qualitatively and quantitatively examined by trained experts and presented as the percentage and the absolute number of each pollen type (Chiara et al., Citation2021). However, this method is highly influenced by the availability of a pollen collection and the analyst’s subjective ability to determine pollen morphology (Soares et al., Citation2015). Moreover, the melissopalynological results can be affected by many factors such as plant morphology, contamination during uncapping and processing, and over- or under-representation of pollen in different types of nectar (Corvucci et al., Citation2015; Mureșan et al., Citation2022). Therefore, many substitutes or complementary methods have been developed to replace or combine with pollen analysis to improve the quality of honey classification, authentication, and identification (Bodor et al., Citation2021b; Panseri et al., Citation2013; Pauliuc et al., Citation2021).
Physicochemical parameters such as moisture, diastase activity, sugar, and acidity, hydroxymethylfurfural (5-HMF) content, ash and electrical conductivity can vary among honey types providing a tool for the determination of the authenticity and quality of honey (Geană et al., Citation2020). These parameters, together with pollen analysis and organoleptic properties, are the principal standard for honey (Soares et al., Citation2017). Recently, physicochemical parameters and volatile compounds have been used to characterize and discriminate mint honey, longan, sunflower, coffee, lychee, citrus, lavender, thyme, buckwheat, chestnut, rhododendron, astragalus, chaste tree, oak, and pine honey (Bokić et al., Citation2020; Karabagias et al., Citation2020; Pattamayutanon et al., Citation2017; Yildiz et al., Citation2022). Different types of honey (i.e. those derived from the nectar of a different plant species) are characterized by volatile fingerprints or by specific volatile compounds, also known as markers, which are useful for botanical classification (Geană et al., Citation2020). For example, cis-linalool oxide and β-linalool are markers of acacia, citrus and rapeseed honey, while carvacrol and p-cymenene are found only in linden honey compared to acacia honey (Nguyen et al., Citation2023; Špánik et al., Citation2014). To discriminate different honey types with the same volatile markers, many methods such as physicochemical, pollen analysis, and the marker’s concentration in honey are usually combined (Nguyen et al., Citation2023). Electrical conductivity was characterized for chestnut honey with the highest value (154 mS m−1) compared to rhododendron (64 mS m−1), lavandula (25 mS m−1), astragalus (40 mS m−1), chaste tree (50 mS m−1), oak (115 mS m−1), and pine honey (113 mS m−1) (Yildiz et al., Citation2022).
However, it is necessary to take into account the fact that volatile compounds could originate from plants or appear during honey processing and storage (Panseri et al., Citation2013).
Due to the variety of topographic and climatic conditions supporting the multiplicity of fruit trees, Vietnamese honey has a high level of diversity, prosperity, and abundance. In fact, Vietnam is ranked 9th worldwide (2021) and accounts for 4.1% of the global export volume of honey (Workman, Citation2016). However, the beekeeping industry in Vietnam is in a formative stage and inadequate scientific data on the authenticity and quality of honey are available. In addition, to our best knowledge, there has been no in-depth study or comprehensive analysis of the physicochemical, melissopalynological, and volatile profile characterization of domestic honey in Vietnam. Therefore, 4 types of honey from Vietnam including blong song (Schefflera heptaphylla L.), bidens (Bidens pilosa L.), coffee (Coffea robusta L.), and mint (Mentha longifolia L.) honey were chosen to be tested and evaluated for the first time. The aim of this study was to characterize and evaluate the quality of the aforementioned Vietnamese honeys by their physicochemical properties, pollen content, and the profile of volatile compounds. The question was raised whether the collected data would provide a correlation between the quality criteria and the botanical origin of honey.
Materials and methods
Honey samples
In this study, 6 honey samples of each type of honey, including blong song (A1–A6), bidens (B1–B6), coffee (C1–C6), and mint (M1–M6) honey, were analyzed. All coffee honey samples were collected in the early 2022 harvest seasons, while the rest were collected in 2021. The locations of the production of Vietnamese honey samples are presented in Figure S1. Among the 6 samples, 5 of them were collected from hobby beekeepers; this number corresponded to the number of production batches of the beekeeping farm, and 1 sample from each group (A6, B6, C6, and M6) was purchased from the market. Samples were stored at 20 °C in a dark room (in glass bottles) for a maximum of 4 months until analysis. Each sample was analyzed in three replicates and mean values were reported.
Melissopalynological analysis
The qualitative and quantitative melissopalynological analysis was performed using the harmonized methods of melissopalynology with authors’ modification (Ohe et al., Citation2004). For each sample of honey, at least 500 pollen grains were counted and classified to taxon. For each type of pollen, the percentage (%) was determined. The percentage representation of individual types of pollen was divided into the following classes: dominant pollen D (>45%), secondary pollen S (16%–45%), minor pollen M (3%–15%), and trace pollen T (<3%). The absolute number of pollen grains was expressed according to the EquationEquation (1)(1)
(1) (PG/10g × 10³).
Expression of the absolute number of pollen grains
(1)
(1)
Note: PG: pollen grain, g: honey weight in gram, S: surface area of the filter (mm2), s: area of one microscopic field (mm2), nPG: total number of PG, a: counted fields number, p: weight of honey (g)
For the analysis, 5 g of homogenised honey was weighted and diluted in 20 ml of temperate water (40 °C), the samples were examined in duplicate. Vacuum filtration was provided by 3 µm microcelulose filter (Millipore, Germany) on a glass filtration aparature. After filtration, the filter was dried overnight and immersed and mounted with Solakryl BMX acrylic resin (Merci, Czech Republic). View fields representing 500 pollen grains were randomly scanned from the microscope slide by XYZ motorised stage Proscan III (Prior, Great Britain) controlled by NIS Elements ver. 5.2 software (Laboratory imagine, Czech Republic). The 600 times magnification was used in Eclipse Ci-L microscope (Nikon, Japan). The view field was scanned in extended deep focus methods by NIS Elements ver. 5.2 software (Laboratory imagine, Czech Republic) and camera DFK 23U274 (Imaging Source, Germany) with resolution 1600 × 1200 pixels, and 8-bit color depth were used. The scanned view field was evaluated by the trained evaluator and pollen grains were compared against palynological databases.
Physicochemical analysis
Physicochemical parameters including water content, electrical conductivity, 5-HMF content, free acidity, color, and diastase activity were analysed following the Harmonised Methods of the International Honey Commission (Bogdanov et al., Citation2009). Water content was measured using a digital refractometer (RM 40, Mettler-Toledo, Grainfensee, Switzerland). Electrical conductivity was determined by the conductometric method (Multi 9310 IDS Conductivity meter, IDS Tetra Con 925 electrode, WTW GmbH, Weilheim, Germany). Free acidity was determined by alkalimetric titration to pH 8.3 (Automatic titrator T5, electrode DGi115-SC, Mettler Toledo, Greifensee, Switzerland). Determination of 5-HMF was performed by HPLC with UV detection (Agilent Technologies 1290 Series, 1290 Infinity Diode Array Detector, Santa Clara, CA, USA). Diastase activity (diastase number) was determined spectrophotometrically using the Phadebas method (Phadebas Honey Diastase Test, Phadebas AB, Kristianstad, Sweden). Color was measured colorimetrically according to a study by Castro et al., Citation1992 using the Pfund scale (HI 96785, Hanna Instruments, Woonsocket, RI, USA).
Sugar profile analysis
Carbohydrate analysis (glucose, fructose, sucrose, turanose, maltose, trehalose, melibiose, and melezitose) was performed using Dionex UltiMate 3000 [ultra-high performance liquid chromatography (UHPLC)] (Thermo Fisher Scientific, US) equipment with a Nucleosil 100-5 NH2-RP column (Macherey-Nagel, Germany) and a Shodex RI-101 refractive index detector (Showa Denko K.K., Japan). Identification and quantification were based on calibration with standards of individual carbohydrates, their retention times and peak-area measurement.
Volatile compounds analysis
Preparation of standards for chromatographic analysis: The commercially available n-alcane standards, 0.2 µL (C5-C8) and 1 µL (C8-C20) were added separately directly into empty 10 mL vials for subsequent retention indices calculation.
Honey samples preparation for chromatographic analysis: 30 g of honey was mixed with 10 mL of distilled water in a plastic sample container with a lid. Subsequently, 4 mL of this honey solution was pipetted into a 10 mL vial.
HS-SPME-GC/MS: The method for the determination of volatile compounds was modified based on the conditions published in the literature (Alissandrakis et al., Citation2007). The HS-SPME method (DVB/CAR/PDMS, 50/30 μm; Supelco) was used to extract analytes from the samples under the following conditions: pre-incubation 20 min at 60 °C, extraction 40 min at 60 °C and stirring speed 250 rpm. In the injection chamber (inlet), analytes were desorbed at 220 °C for 3 min and injected in a 1:1 split mode, which ensured good sensitivity and acceptable peak shape throughout the chromatogram.
GC/MS analysis was performed using an Agilent Technologies 7890 A gas chromatograph connected to an Agilent Technologies 5975 C mass detector. An HP-5MS capillary column, 30 m x 0.25 mm ID, film thickness 0.25 µm, was used. The temperature gradient started at 40 °C (3 min), followed by a temperature increase at a rate of 3 °C min−1 to 160 °C and a further temperature increase at a rate of 10 °C min−1 to 250 °C. The total run time was 52 min. The injector temperature (Ultra Inert Straight SPME Liner, Agilent) was 220 °C. Helium 4.8 with a flow rate of 0.7 mL min−1 was used as the carrier gas. Electron ionization with ionization energy of 70 eV, with an ion source temperature of 230 °C and a quadrupole analyser of 290 °C, with a measuring mass range m/z 50–550 was used for MS.
The chromatograms were evaluated using HP Chemstation software (Agilent Technologies) and further processed by targeted analysis of the 26 most abundant compounds, which were identified by comparing the spectra with the NIST library of mass spectra (NIST 14) and by retention indices (RI) and quantified by relative peak areas (expressed as % from total peak areas, full scan; RTE integrator).
Statistical analysis
All experimental results were based on three replicates and expressed as mean ± standard deviation. The one-way analysis of variance (ANOVA) test was applied to collect the differences between the means (Statgraphics Technologies, Inc., USA). p-values < 0.05 were considered statistically significant. Principal component analysis (Statistica 12.0, StatSoft, Tulsa, Oklahoma, USA) was used to investigate whether the profile of sugars and volatiles would allow discrimination of the botanical origin of the 4 types of honey.
Results
Pollen profile
The melissopalynological profile significantly differed among the 4 types of honey (). The characteristic pollen grains for the individual types of honey are shown in Figure S2. The content of specific pollen was highest for blong song honey (87 ± 2.8%) followed by coffee honey (37.5 ± 8.1%), bidens honey (5.7 ± 2.5%), and mint honey (at 2.7 ± 2.0%). According to the Maurizio classification, the honey samples had medium pollen content (Ohe et al., Citation2004). Most of the samples (A1-A4, A6, C1-C6, B1-B6, and M1-M5) (91.7%) belonged to class III of Maurizio (101 × 103 ≤ PG 10 g−1 ≤ 500 × 103) and only 8.3% (A5 and M6) fell into class II (20 × 103 ≤ PG 10 g−1 ≤ 100 × 103).
Table 1. Quantitative pollen content and percentage representation of individual types (D: >45%, S: 16%–45%, M: 3%–15%, and T: <3%).
Physicochemical parameters
The physicochemical parameters of four honey types including electrical conductivity, free acidity, diastase activity, 5-HMF and colour showed a statistically significant difference (p < 0.05) (). Most of the samples in our study are considered fresh and of good quality according to the European Honey Directive (2001) (Council, Citation2002) except for sample M6 and low diastase values were observed for blong song honey (< 2 DN). Due to the suspicion of unwanted modification of sample M6 (from the market), its values of parameters associated with heating (5-HMF, diastase, color) were not included in .
Table 2. The physicochemical parameters and sugars of blong song, bidens, coffee, and mint honey.
The moisture content in all samples remained stable (18.6%–19.8%) and less than the maximum acceptable limit (20%) according to the International Regulations of Quality (Codex Alimentarius Commission, Citation2001). The 5-HMF content of blong song, bidens, coffee, and mint honey was in the ranges of 30.5–49.0, 2.6–3.4, 16.1–39.3, and 2.7–129.5 mg kg−1, respectively. DN values and free acid content were significantly different (p < 0.05) among the four analysed groups. The lowest acid content was recorded in blong song honey (9.5 ± 0.8 meq kg−1) while the highest was in coffee honey (19.7 ± 1.9 meq kg−1). The electrical conductivities of blong song, coffee, bidens, and mint honey ranged from 8.2 to 11.1, 28.1 to 37.7, 19.3 to 19.6, and 20.1 to 24.2 mS m−1, respectively. Blong song honey had the lowest Pfund value (< 1.0 mm) followed by bidens (21.3 ± 0.5 mm), mint (31.3 ± 15.7 mm), and coffee honey (44.7 ± 2.7 mm).
Sugar profile
The results, shown in , presented seven mono and disaccharides in blong song, coffee, bidens, and mint honey. The lowest value of F + G was observed for bidens honey (about 65 g 100 g−1) whereas about 71 g 100 g−1 honey was recorded for the rest. The highest sucrose content was detected in bidens honey (with an average of 4.9 g 100 g−1) followed by mint honey (with an average of 1.2 g 100 g−1). No sucrose is present in coffee and blong song honey. Maltose content in bidens honey was significantly higher than the other honey types (p < 0.05). In addition, trehalose was only found in blong song honey (0.36 g 100 g−1) while melibiose was detected in both mint (4.6 g 100 g−1) and bidens (8.1 g 100 g−1) honey. The presence of turanose was on average less than 1 g 100 g−1. Additionally, the results of PCA obtained by performing the presence of 7 sugars in 4 different types of honey emphasized the difference among them ().
Volatile compounds analysis
The 26 major volatile compounds presented in blong song, bidens, coffee, and mint honey are listed in ; the condition for inclusion was the relative representation higher than 1% for at least one type of honey. Mint honey showed a uniqueness in terms of compounds including menthone (RI: 1158), menthol isomers (RI: 1172), and isomethyl acetate (RI: 1299). Citral co-eluting with nonanoic acid (RI: 1269), α-terpineol (RI: 1198), and 1-nonanol (RI: 1175) was found predominantly in coffee honey. Acetic acid phenylethyl ester (RI: 1256) was dominantly present in bidens honey, whose volatile compounds profile was considerably poor compared to the other samples. Furfural (RI: 829) and linalool oxide isomers (RI: 1102) were identified in a higher average amount in blong song honey. Furfural, similar to 5-HMF, is a sugar degradation product, and its increased content can be correlated with low diastase activity (Ahmed et al., Citation2014; Perez-Arquillue et al., Citation1994). Benzeneacetaldehyde (RI: 1040), undecane, and linalool were found in blong song, bidens, and coffee honey but they were remarkably high in coffee honey. The relationship between honey samples is shown in using PCA analysis of the volatile profiles of 4 types of Vietnamese honey. PC1 and PC2 contributed 39.41% and 24.83% of the variance, respectively. The proximity among the samples of each honey type reflected the similarities of the variables.
Figure 2. Principal component analysis (PCA) diagrams: (a) PCA score plot of blong song honey (A), bidens honey (B), coffee honey (C), and mint (M) honey and (b) Loading plot of PCA—26 volatile compounds.
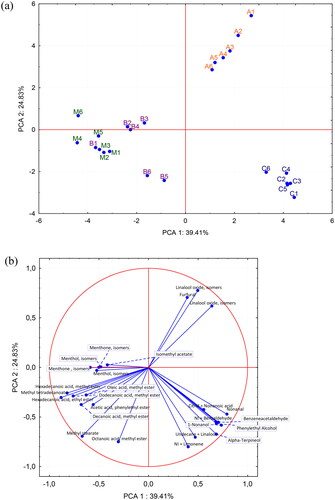
Table 3. The main volatile compounds (expressed as relative representation in %) that were present in blong song (A1-A6), bidens (B1-B6), coffee (C1-C6), and mint (M1-M6) honey.
Discussion
The monofloral origin of blong song honey was characterised by the high percentage of Schefflera heptaphylla pollen (87%). In general, monofloral honey requires at least 45% of representative pollen grains from the taxon claimed (Ohe et al., Citation2004). In all blong song honey samples, pollen grains of Schefflera heptaphylla are considered to be over-represented due to the extraordinarily high percentage in pollen spectra. Over-represented pollen grains were established in other monofloral honey like Castanea (86%) (Ohe et al., Citation2004), Eucalyptus spp. (83%) (Ohe et al., Citation2004), Castanea sativa Mill (> 90%) (Persano Oddo & Piro, Citation2004), Mimosa scabrella Benth. (92%) (Azevedo et al., Citation2017) and Myracrodruon (84%) (Calaça et al., Citation2018). The reason for the uniform pollen spectra of blong song honey can be explained by the dissimilarity of synchronized blooming seasonality of this species compared to other melliferous plants in the bee farm area.
Previous studies reported the percentage of Coffea arabica pollen in the range of 73%–78% for coffee honey samples from Brazil (Kadri et al., Citation2016), 54.4%–94.2% from Ethiopia (Debela & Belay, Citation2021), 64%–95% from Colombia and 36% from Honduras (Bodor et al., Citation2021b). The variation of predominant pollen in different samples was generally influenced by the pollen-producing capacity and the geographic origin of the honey (Bodor et al., Citation2021b; Oddo et al., Citation1995). For instance, the percentage of Robinia pseudocacacia pollen for acacia honey was 28% in a European study, 13% in Czech honey, 22%–68% in samples from northwest Croatia, 11%–70% in eastern Croatia, and 33%–71% in Istria (Pospiech et al., Citation2021; Uršulin-Trstenjak et al., Citation2017). Therefore, the set of coffee honey in our study with Coffea robusta pollen representation ranging from 25% to 49% can be considered monofloral.
Mint and bidens honey were characterized by a lower specific pollen representation compared to the others (2.7%–6.2% and 5.7%–8.8% for mint and biden honey, respectively). These honey types cannot meet the general requirement of percent frequency types of the main pollen grains (≥45%) but there are exceptions for some honey types with under-represented pollen grains due to nectar and pollen production situation. A low pollen content of Mentha spp. (21%–28%) was also observed for mint honey samples in Croatia (Pospiech et al., Citation2021). Indeed, according to the International Honey Commission, the presence of the main pollen types in monofloral honey can be as low as 5%–25% for Carduus, 2%–42% for Citrus, and 1%–56% for Tilia (Persano Oddo & Piro, Citation2004). Unfortunately, there is no national or international requirement regarding these types of honey. With regard to the low presentation of specific pollens and limited information in the specific literature, it is difficult to prove the monofloral nature of mint and bidens honeys based only on pollen analysis. For authentification of honey, the percentage of the main pollen type is important but the percentages of secondary pollen types are also essential in the case of the authentification regarding the geographical origin of the imprescindible samples. Samples of blong song and coffee honey met the requirements of monofloral honey. Mint and bidens honey had a low specific pollen representation so we cannot conclude whether they are monofloral or not. We need to use other methods such as organoleptic or physiochemical analysis to withdraw the conclusion. Typical physicochemical properties and organoleptic properties such as smell, taste and appearance that were associated with specific chemical compositions are important (Persano Oddo & Piro, Citation2004). For example, linalool is responsible for citrus flavour (Viuda‐Martos et al., Citation2010), hexanal characterizes flavour of lavender honey (Manyi-Loh et al., Citation2011) and methyl alcohols relate to the typically fresh aroma (Da Silva et al., Citation2016).
The moisture content of honey depends on various factors such as the source of the honey, the harvest time, environmental conditions, and the manipulation of the beekeeper (Pham et al., Citation2022). It is a limiting indicator for the stability of honey (Zarei et al., Citation2019). The water content in honey above 20% can promote the fermentative process and the growth of osmophilic yeasts creating undesirable flavours (Saxena et al., Citation2010). In a previous study, different results were obtained in the moisture content of 22.5% and 14.2% for Vietnamese coffee and mint honey, respectively (Pham et al., Citation2022). However, in general, compared to honey from other countries with the same climate and soil conditions as Vietnam, similar results were also obtained for honey from Thailand (17.0%–20.0%) (Wetwitayaklung et al., Citation2018), and Malaysia (17.0%–19.1%) (Moniruzzaman et al., Citation2013b).
5-HMF and diastase are two important parameters to assess the freshness and/or overheating of honey (Nguyen et al., Citation2023; Soares et al., Citation2017). High 5-HMF and low diastase activity indicate overheating during pasteurization process, extended storage period and poor storage conditions (Ruoff & Bogdanov, Citation2004). The maximum 5-HMF content of 40 mg kg−1 and the minimum diastase number (DN) of 8 have been set by the Codex Alimentarius Standard (2001) (Codex Alimentarius Commission, Citation2001) and EU Council directive (2002) (Council, Citation2002). However, some botanical types of honey originated from tropical countries (e.g. citrus honey) had a higher maximum 5-HMF content of 80 mg kg−1 and a lower minimum DN of 3 (Codex Alimentarius Commission, Citation2001).
Most of the samples passed the 5-HMF criteria with one exception of M6 which comes from a set of mint honey samples (129.5 mg kg−1). This sample may be old or overheated during processing. The initial 5-HMF content of bidens honey was 1.21 mg kg−1 and reached 80 mg kg−1 after 6 months of storage at 35 °C (Chou et al., Citation2020). The 5-HMF content of Vietnamese honey including longan, coffee, mint, lychee, coconut, rubber, melaleuca, rambutan, mangrove, acacia and manuka was reported to range from 12.4 to 58.6 mg kg−1 (Pham et al., Citation2022). The blooming season of each flower type is as follows Mint: October–December; Coffee: November–February; Blong song: August–September; and Biden: year-round. The different blooming seasons of these flower types may contribute to the significant differences in four sets of honey samples. Similar results were obtained in the study of Chuttong et al. (Citation2016) with 5-HMF contents ranged from 2.4 to 46.0 mg kg−1 (Chuttong et al., Citation2016).
According to the regulation for honey in tropical countries, samples of bidens, coffee, and mint honey conformed to the DN standard, except blong song honey. The values of DN in blong song honey samples were lower than minimum requirement (< 2.0 DN) and the 5-HMF contents were slightly elevated (but not more than the limit of 80 mg kg−1). Therefore, it is difficult to conclude whether these parameters could be characteristic for this type of honey or whether honey management could affect them. It would be necessary to measure a larger set of samples in the future to confirm this. Similarly, a low DN value of 1.5 ± 1.6 was also detected in honey samples from Thailand (Chuttong et al., Citation2016).
Free acids, particularly gluconic, pyruvic, and citric, in honey contribute to the flavor and are partially responsible for antimicrobial activity (Isla et al., Citation2011). All the studied honey samples from different floral sources showed a statistically significant difference (p < 0.05) in free acid content. Our results confirm the acidic nature of honey which is limited to < 50 meq kg−1 according to EU Council directive (2002) and International Regulations (Codex Alimentarius Commission, Citation2001). The initial free acid value of bidens honey from Taiwan was 43 meq kg−1 and was higher (50 meq kg−1) after 9 months of storage at 35 °C (Chou et al., Citation2020). The free acidity of Vietnamese honey samples was close to those previously reported in Vietnamese longan and coffee honey (15–30 meq kg−1) (Trinh et al., Citation2022) or in general blossom honey (12.5–24.5 meq kg−1) (Nguyen et al., Citation2023).
All honey samples met the regulatory requirement that the limit of electrical conductivity of blossom honey does not exceed 80 mS m−1 (Codex Alimentarius Commission, Citation2001). Four types of studied honey samples showed a statistically significant difference (p < 0.05) in electrical conductivity. In this study, the correlation between electrical conductivity and acid content of honey was observed. Pires et al. (Citation2009) suggested that the higher the content of ions and organic acids, the higher the electric conductivity values (Pires et al., Citation2009). Moreover, the variations in electric conductivity in different honey were influenced by floral origin (Kropf et al., Citation2008).
According to the Pfund scale in , blong song honey had a white color of water (Bodor et al., Citation2021a). Bidens and mint honey were white and extra light amber while coffee honey was light amber. Honey pigments depend on floral origin, minerals, and organic compounds such as phenolics and flavonoids (Moniruzzaman et al., Citation2013a). In our study, the color of 4 types of honey depended on their botanical origin. Therefore, the color of honey can be used as an appropriate parameter for classifying monofloral honey (Oddo & Bogdanov, Citation2004).
Sugars make up the majority of honey components and can be useful for authenticating the origin of honey (Isla et al., Citation2011; Pospiech et al., Citation2021). Glucose and fructose are predominant carbohydrates accounting for more than 65% of total fresh weight while the other sugars include sucrose, turanose, trehalose, and melibiose. Fructose is normally the predominant carbohydrate in most honey with the exception of some honey such as rape (Brassica napus) and dandelion (Taraxacum officinale), where the glucose content is slightly higher than fructose content (Da Silva et al., Citation2016; Escuredo et al., Citation2014). In our study, the fructose content is also higher than the glucose content for all 4 types of Vietnamese honey.
According to European legislation and codex standards (Codex Alimentarius Commission, Citation2001), blossom honey should contain a minimum content of sum fructose and glucose (F + G) of 60 g 100 g−1. In this study, all samples met the requirement for blossom honey according to European legislation and codex standards. Similar results were also obtained for other Vietnamese honey (62.6–76.1 g 100 g−1) (Trinh et al., Citation2022) and the lower F + G values (61.2–63.9 g 100 g−1) were presented in honey samples from Malaysia (Moniruzzaman et al., Citation2013b).
The amount of sucrose in 4 types of honey met the limit according to the Codex Alimentarius and the EU standard (<5 g 100 g−1) (Codex Alimentarius Commission, Citation2001). Sucrose is an important constituent for evaluating and screening honey quality (Belay et al., Citation2017). High sucrose values indicate incomplete maturation and the possibility of adulteration with commercial sugar or bee feeding.
Other sugars such as maltose, turanose, trehalose, melibiose, and melezitose were also investigated in honey samples. Melezitose was not detected (<0.1 g 100 g−1) in any of the tested samples; its content is a monitored parameter of honey quality because this sugar triggers rapid crystallization of honey in high concentrations (Čížková et al., Citation2022). The content of melezitose in blossom honey is significantly lower than in honeydew, for example, 0.1 g 100 g−1 was found for chestnut and 0.3 g 100 g−1 for heather honey while 3.2 g 100 g−1 for honey from Abies alba and 4.9 g 100 g−1 for mixed honeydew honey (Čížková et al., Citation2022; Seeburger et al., Citation2022). Although these sugars are considered minor carbohydrates, their presence is attributed to the different botanical origins (Bergamo et al., Citation2019).
Principal component analysis (PCA) is a useful tool for assessing the association among data, samples, and their distribution (Kamal et al., Citation2019). The first principal component (PC1) accounted for 54.47% of the total data variability and the second principal component (PC2) occupied 22.96% (). The blong song, bidens, coffee, and mint honey were grouped separately, demonstrating the success in discrimination of these honeys excluding sample M6 from the set of mint honey. Among 7 sugars, trehalose is present in blong song honey only; bidens honey is characterized by a high proportion of monitored disaccharides (melibiose, sucrose, and maltose); mint honey is distinguished from others by its turanose content; and glucose and fructose are not specific for any honey types.
A considerable number of 82; 73; 61; and 49 of peaks representing individual volatile compounds were recorded with the total peak area of 5.4 ± 0.1; 20 ± 0.2; 5.5 ± 0.4; and 2.3 ± 0.2 (×107 abundance × second) for blong song, mint, coffee, and bidens honey, respectively. The total peak area showed the richness of volatile compounds concentration of mint honey and the poor of bidens honey. These compounds were classified into aldehydes, alcohols, alkanes, esters, acids, and hydrocarbons which significantly contribute to the general honey aroma or the unique scent of certain plants (Seisonen et al., Citation2015). Some compounds were previously identified in the honey and also in the nectar whereas the others were produced by bees. For example, linalool, methyl anthranilate and α-terpineol were identified in both nectar and honey samples while linalool derivatives were found only in honey due to the hive conditions and bee enzyme activity (Jerković et al., Citation2016). To the best of our knowledge, the volatile compounds analysis of bidens, blong song, and mint honey have never been reported. Several compounds were found in the ethanol and water (7:3) extract and methanol extract from leaves of B. pilosa by GC-MS including 1,3,6,10-dodecatetraene, 3,7,11-trimethyl-(Z,E) (α-farnesene); 1H-3A, 7-methanozulene, octahydro-1,4,9,9-tetramethyl (patchoulane); 9H–fluorene, 9-diazo (diazofluorene); 1-octadecyne; n-hexadecanoic acid (palmitic acid); 3,7,11,15-tetramethyl-2-hexadecen-1-ol; tetradecanoic acid (myristic acid), hexadecanoic acid ethyl ester (ethyl palmitate); phytol (florasol, phytosol); DL-α-tocopherol (vitamin E); and phenol, 2,2′-methylenebis [6-(1,1-dimethylethyl)]-4-methyl (Falowo et al., Citation2017; Singh et al., Citation2017). The volatile compounds profiles of mint extracts from leaves, stems and branches were characterized with the main constituents as menthol, carvone, piperitenone, linalyl acetate, p-menthone, linalool, limonene, 1,8-cineol, α-terpinyl acetate, pulegone, and β-pinene (Bokić et al., Citation2020; de Oliveira et al., Citation2021; Kowalczyk et al., Citation2021).
Regarding botanical markers for coffee honey, 2-furanmethanol, butyryl lactone, phenylmethanol, anisaldehyde, anise alcohol, and 3,4,5-trimethylphenol were suggested (Pattamayutanon et al., Citation2017). However, a totally different result was obtained in our study. The difference could be due to the coffee varieties and different honey bee species. In our study, Coffea robusta was used, but it was not available in the study of Pattamayutanon et al. (Citation2017). Citral, nonanoic acid, α-terpineol, 1-nonanol, and benzeneacetaldehyde could be considered as markers for coffee honey. Although undecane and linalool were found in notably high quantities, they could not be considered specific to this honey because they were found in both blong song and bidens honey. Linalool was also found in lychee, logan, and citrus honey (Karabagias et al., Citation2020; Pattamayutanon et al., Citation2017).
Generally, the PCA results () showed that some botanical origins could be well distinguished with a relatively independent space. The honey samples of blong song and coffee were clearly separated, whereas mint honey was partially overlapped with bidens honey (mainly B1). Mint honey was found in the lower left quadrant except for M6 which is of unsatisfactory physicochemical parameters probably due to heated and therefore obviously the composition of volatile substances was also affected. Blong song and coffee honey were found in the upper and lower right quadrant, respectively. The results suggested the pure blossom honey of these samples. In contrast, the set of bidens honey may contain highly variable volatile compounds.
Conclusions
The physicochemical properties of blong song, bidens, coffee, and mint honey from Vietnam mostly complied with the general criteria according to EU Council directive and International Regulations except for DN value of blong song honey. The set of blong song and coffee honey can be considered as monofloral honey while there is no national and international standard for mint and bidens honey. Based on the results of volatile compounds menthone, menthol isomers, and isomethyl acetate were found to be typical for mint honey. Moreover, linalool oxide isomers had the highest representation in blong song honey whereas coffee honey was characterized by citral, nonanoic acid, α-terpineol, 1-nonanol and benzeneacetaldehyde. Our results also showed that some minor carbohydrates such as maltose, sucrose, trehalose, melibiose, and turanose can be used to discriminate between tested Vietnamese monofloral honeys.
Supplementary.docx
Download MS Word (1 MB)Disclosure statement
The authors declare no conflict of interest.
Additional information
Funding
References
- Ahmed, M., Khiati, B., Meslem, A., Aissat, S., & Djebli, N. (2014). Evaluation of physicochemical and antioxidant properties of raw honey from Algeria. Journal of Microbial & Biochemical Technology, s1(01), 1–6. https://doi.org/10.4172/1948-5948.S4-006
- Alissandrakis, E., Tarantilis, P. A., Harizanis, P. C., & Polissiou, M. (2007). Comparison of the volatile composition in thyme honeys from several origins in Greece. Journal of Agricultural and Food Chemistry, 55(20), 8152–8157. https://doi.org/10.1021/jf071442y
- Azevedo, M. S., Valentim-Neto, P. A., Seraglio, S. K. T., da Luz, C., F. P., Arisi, A. C. M., & Costa, A. C. O. (2017). Proteome comparison for discrimination between honeydew and floral honeys from botanical species Mimosa scabrella Bentham by principal component analysis. Journal of the Science of Food and Agriculture, 97(13), 4515–4519. https://doi.org/10.1002/jsfa.8317
- Belay, A., Haki, G. D., Birringer, M., Borck, H., Lee, Y. C., Cho, C. W., Kim, K. T., Bayissa, B., Baye, K., & Melaku, S. (2017). Sugar profile and physicochemical properties of Ethiopian monofloral honey. International Journal of Food Properties, 20(11), 2855–2866. https://doi.org/10.1080/10942912.2016.1255898
- Bergamo, G., Seraglio, S. K. T., Gonzaga, L. V., Fett, R., & Costa, A. C. O. (2019). Physicochemical characteristics of bracatinga honeydew honey and blossom honey produced in the state of Santa Catarina: An approach to honey differentiation. Food Research International (Ottawa, Ont.), 116, 745–754. https://doi.org/10.1016/j.foodres.2018.09.007
- Bodor, Z., Benedek, C., Urbin, Á., Szabó, D., & Sipos, L. (2021a). Colour of honey: Can we trust the Pfund scale?: An alternative graphical tool covering the whole visible spectra. LWT, 149, 111859. https://doi.org/10.1016/j.lwt.2021.111859
- Bodor, Z., Kovacs, Z., Benedek, C., Hitka, G., & Behling, H. (2021b). Origin identification of Hungarian honey using melissopalynology, physicochemical analysis, and near infrared spectroscopy. Molecules (Basel, Switzerland), 26(23), 7274. https://doi.org/10.3390/molecules26237274
- Bogdanov, S., Martin, P., & Lullmann, C. (2009). Harmonised methods of the international honey commission. Int. Honey Comm, 62.
- Bokić, B. S., Rat, M. M., Kladar, N. V., Anačkov, G. T., & Božin, B. N. (2020). Chemical diversity of volatile compounds of mints from southern part of Pannonian Plain and Balkan Peninsula—New Data. Chemistry & Biodiversity, 17(8), e2000211. https://doi.org/10.1002/cbdv.202000211
- Bueno-Costa, F. M., Zambiazi, R. C., Bohmer, B. W., Chaves, F. C., Silva, W. P., da, Zanusso, J. T., & Dutra, I. (2016). Antibacterial and antioxidant activity of honeys from the state of Rio Grande do Sul, Brazil. LWT—Food Science and Technology, 65, 333–340. https://doi.org/10.1016/j.lwt.2015.08.018
- Calaça, P., Schlindwein, C., & Bastos, E. M. A. F. (2018). Discriminating unifloral honey from a dioecious mass flowering tree of Brazilian seasonally dry tropical forest through pollen spectra: Consequences of honeybee preference for staminate flowers. Apidologie, 49(6), 705–720. https://doi.org/10.1007/s13592-018-0597-8
- Castro, R. M., Escamilla, M. J., & Reig, F. B. (1992). Evaluation of the color of some Spanish unifloral honey types as a characterization parameter. Journal of AOAC International, 75(3), 537–542. https://doi.org/10.1093/jaoac/75.3.537
- Chen, C. T., Chen, B. Y., Nai, Y. S., Chang, Y. M., Chen, K. H., & Chen, Y. W. (2019). Novel inspection of sugar residue and origin in honey based on the 13C/12C isotopic ratio and protein content. Journal of Food and Drug Analysis, 27(1), 175–183. https://doi.org/10.1016/j.jfda.2018.08.004
- Chiara, B., Francesco, C., Fulvio, B., Paola, M., Annalisa, G., Stefania, S., Luigi, A. P., & Simone, P. (2021). Exploring the botanical composition of polyfloral and monofloral honeys through DNA metabarcoding. Food Control, 128, 108175. https://doi.org/10.1016/j.foodcont.2021.108175
- Chou, W., Liao, H., Yang, Y., & Peng, C. (2020). Evaluation of honey quality with stored time and temperatures. Journal of Food and Nutrition Research, 8(10), 591–599. https://doi.org/10.12691/jfnr-8-10-8
- Chuttong, B., Chanbang, Y., Sringarm, K., & Burgett, M. (2016). Physicochemical profiles of stingless bee (Apidae: Meliponini) honey from South East Asia (Thailand). Food Chemistry, 192, 149–155. https://doi.org/10.1016/j.foodchem.2015.06.089
- Čížková, H., Titěra, D., Hrabec, P., & Pospiech, M. (2022). Nine-year statistics of Czech honey carbohydrate profiles in the Czech Republic. Czech Journal of Food Sciences, 40(2), 85–92. https://doi.org/10.17221/213/2021-CJFS
- Codex Alimentarius Commission. (2001). Report of the seventh session of the codex committee on sugars. FAO, ALINORM, 2–7.
- Corvucci, F., Nobili, L., Melucci, D., & Grillenzoni, F. V. (2015). The discrimination of honey origin using melissopalynology and Raman spectroscopy techniques coupled with multivariate analysis. Food Chemistry, 169, 297–304. https://doi.org/10.1016/j.foodchem.2014.07.122
- Council, E. U. (2002). Council directive 2001/110/EC. Off. J. Eur. Communities, L10/(47), 47–52.
- Da Silva, P. M., Gauche, C., Gonzaga, L. V., Costa, A. C. O., & Fett, R. (2016). Honey: Chemical composition, stability and authenticity. Food Chemistry, 196, 309–323. https://doi.org/10.1016/j.foodchem.2015.09.051
- de Oliveira, T., Balduino, M. C. M., de Carvalho, A. A., Bertolucci, S. K. V., Cossa, M. C., Coelho, A. D., Leite, J. J. F., & Pinto, J. E. B. P. (2021). The effect of alternative membrane system, sucrose, and culture methods under photosynthetic photon flux on growth and volatile compounds of mint in vitro. In Vitro Cellular & Developmental Biology—Plant, 57(3), 529–540. https://doi.org/10.1007/s11627-020-10147-z
- Debela, H., & Belay, A. (2021). Caffeine, invertase enzyme and triangle test sensory panel used to differentiate Coffea arabica and Vernonia amygdalina honey. Food Control, 123, 107857. https://doi.org/10.1016/j.foodcont.2020.107857
- Escuredo, O., Dobre, I., Fernández-González, M., & Seijo, M. C. (2014). Contribution of botanical origin and sugar composition of honeys on the crystallization phenomenon. Food Chemistry, 149, 84–90. https://doi.org/10.1016/j.foodchem.2013.10.097
- Falowo, A. B., Muchenje, V., Hugo, A., Aiyegoro, O. A., & Fayemi, P. O. (2017). Antioxidant activities of Moringa oleifera L. and Bidens pilosa L. leaf extracts and their effects on oxidative stability of ground raw beef during refrigeration storage. CyTA—Journal of Food, 15(2), 249–256. https://doi.org/10.1080/19476337.2016.1243587
- Geană, E.-I., Ciucure, C. T., Costinel, D., & Ionete, R. E. (2020). Evaluation of honey in terms of quality and authenticity based on the general physicochemical pattern, major sugar composition and δ13C signature. Food Control, 109, 106919. https://doi.org/10.1016/j.foodcont.2019.106919
- Grabato, J. R., Pilario, K. E., Micor, J. R. L., & Mojica, E.-R. E. (2022). Geographical and entomological differentiation of Philippine honey by multivariate analysis of FTIR spectra. Journal of Food Composition and Analysis, 114, 104853. https://doi.org/10.1016/j.jfca.2022.104853
- Isla, M. I., Craig, A., Ordoñez, R., Zampini, C., Sayago, J., Bedascarrasbure, E., Alvarez, A., Salomón, V., & Maldonado, L. (2011). Physico chemical and bioactive properties of honeys from Northwestern Argentina. LWT—Food Science and Technology, 44(9), 1922–1930. https://doi.org/10.1016/j.lwt.2011.04.003
- Jerković, I., Prđun, S., Marijanović, Z., Zekić, M., Bubalo, D., Svečnjak, L., & Tuberoso, C. (2016). Traceability of Satsuma Mandarin (Citrus unshiu Marc.) honey through nectar/honey-sac/honey pathways of the headspace, volatiles, and semi-volatiles: chemical markers. Molecules (Basel, Switzerland), 21(10), 1302. https://doi.org/10.3390/molecules21101302
- Kadri, S. M., Zaluski, R., Pereira Lima, G. P., Mazzafera, P., & De Oliveira Orsi, R. (2016). Characterization of Coffea arabica monofloral honey from Espírito Santo, Brazil. Food Chemistry, 203, 252–257. https://doi.org/10.1016/j.foodchem.2016.02.074
- Kamal, M. M., Rashid, M. H. U., Mondal, S. C., El Taj, H. F., & Jung, C. (2019). Physicochemical and microbiological characteristics of honey obtained through sugar feeding of bees. Journal of Food Science and Technology, 56(4), 2267–2277. https://doi.org/10.1007/s13197-019-03714-9
- Karabagias, I. K., Badeka, A., & Kontominas, M. G. (2020). A decisive strategy for monofloral honey authentication using analysis of volatile compounds and pattern recognition techniques. Microchemical Journal, 152, 104263. https://doi.org/10.1016/j.microc.2019.104263
- Kowalczyk, A., Piątkowska, E., Kuś, P., Marijanović, Z., Jerković, I., Tuberoso, C. I. G., & Fecka, I. (2021). Volatile compounds and antibacterial effect of commercial mint cultivars—chemotypes and safety. Industrial Crops and Products, 166, 113430. https://doi.org/10.1016/j.indcrop.2021.113430
- Kropf, U., Jamnik, M., Bertoncelj, J., & Golob, T. (2008). Linear regression model of the ash mass fraction and electrical conductivity for slovenian honey. Food Technol. Biotechnol, 46, 335–340.
- Manyi-Loh, C. E., Ndip, R. N., & Clarke, A. M. (2011). Volatile compounds in honey : A review on their involvement in aroma, botanical origin determination and potential biomedical activities. International Journal of Molecular Sciences, 12(12), 9514–9532. https://doi.org/10.3390/ijms12129514
- Matkovits, A., Nagy, K., Fodor, M., & Jókai, Z. (2023). Analysis of polyphenolic components of Hungarian acacia (Robinia pseudoacacia) honey; method development, statistical evaluation. Journal of Food Composition and Analysis, 120, 105336. https://doi.org/10.1016/j.jfca.2023.105336
- Moniruzzaman, M., Sulaiman, S. A., Azlan, S. A. M., & Gan, S. H. (2013a). Two-year variations of phenolics, flavonoids and antioxidant contents in acacia honey. Molecules (Basel, Switzerland), 18(12), 14694–14710. https://doi.org/10.3390/molecules181214694
- Moniruzzaman, M., Sulaiman, S. A., Khalil, M. I., & Gan, S. H. (2013b). Evaluation of physicochemical and antioxidant properties of sourwood and other Malaysian honeys: A comparison with manuka honey. Chemistry Central Journal, 7(1), 138. https://doi.org/10.1186/1752-153X-7-138
- Mureșan, C. I., Cornea-Cipcigan, M., Suharoschi, R., Erler, S., & Mărgăoan, R. (2022). Honey botanical origin and honey-specific protein pattern: Characterization of some European honeys. LWT, 154, 112883. https://doi.org/10.1016/j.lwt.2021.112883
- Nguyen, T. Q. N., Hanková, M., Kružík, V., Grégrová, A., Škorpilová, T., Štarha, P., Tran, V. N., & Čížková, H. (2023). Determination of volatile compound profiles and physico-chemical analysis of linden and acacia Czech honey. Journal of Apicultural Research, 62(2), 374–382. https://doi.org/10.1080/00218839.2022.2146346
- Oddo, L. P., & Bogdanov, S. (2004). Determination of honey botanical origin: Problems and issues. Apidologie, 35(Suppl. 1), S2–S3. https://doi.org/10.1051/apido:2004044
- Oddo, L. P., Piazza, M. G., Sabatini, A. G., & Accorti, M. (1995). Characterization of unifloral honeys. Apidologie, 26(6), 453–465. https://doi.org/10.1051/apido:19950602
- Ohe, W. V. d., Oddo, L. P., Piana, M. L., Morlot, M., & Martin, P. (2004). Harmonized methods of melissopalynology. Apidologie, 35(Suppl. 1), S18–S25. https://doi.org/10.1051/apido:2004050
- Panseri, S., Manzo, A., Chiesa, L. M., & Giorgi, A. (2013). Melissopalynological and volatile compounds analysis of buckwheat honey from different geographical origins and their role in botanical determination. Journal of Chemistry, 2013, 1–11. https://doi.org/10.1155/2013/904202
- Pattamayutanon, P., Angeli, S., Thakeow, P., Abraham, J., Disayathanoowat, T., & Chantawannakul, P. (2017). Volatile organic compounds of Thai honeys produced from several floral sources by different honey bee species. PloS One, 12(2), e0172099. https://doi.org/10.1371/journal.pone.0172099
- Pauliuc, D., Ciursă, P., Ropciuc, S., Dranca, F., & Oroian, M. (2021). Physicochemical parameters prediction and authentication of different monofloral honeys based on FTIR spectra. Journal of Food Composition and Analysis, 102, 104021. https://doi.org/10.1016/j.jfca.2021.104021
- Pauliuc, D., Dranca, F., Ropciuc, S., & Oroian, M. (2022). Advanced characterization of monofloral honeys from Romania. Agriculture, 12(4), 526. https://doi.org/10.3390/agriculture12040526
- Perez-Arquillue, C., Conchello, P., Arino, A., Juan, T., & Herrera, A. (1994). Quality evaluation of Spanish rosemary (Rosmarinus officinalis) honey. Food Chemistry, 51(2), 207–210. https://doi.org/10.1016/0308-8146(94)90258-5
- Persano Oddo, L., & Piro, R. (2004). Main European unifloral honeys: Descriptive sheets. Apidologie, 35(Suppl. 1), S38–S81. https://doi.org/10.1051/apido:2004049
- Pham, T. N., Nguyen, T. V., Le, D. T., Diep, L. M. N., Nguyen, K. N., To, T. H. N., Le, T. H., & Nguyen, Q. V. (2022). Phenolic profiles, antioxidant, antibacterial activities and nutritional value of Vietnamese honey from different botanical and geographical sources. AgriEngineering, 4(4), 1116–1138. https://doi.org/10.3390/agriengineering4040069
- Pires, J., Estevinho, M. L., Feás, X., Cantalapiedra, J., & Iglesias, A. (2009). Pollen spectrum and physico-chemical attributes of heather (Erica sp.) honeys of north Portugal. Journal of the Science of Food and Agriculture, 89(11), 1862–1870. https://doi.org/10.1002/jsfa.3663
- Pospiech, M., Javůrková, Z., Hrabec, P., Čížková, H., Titěra, D., Štarha, P., Ljasovská, S., Kružík, V., Podskalská, T., Bednář, J., Burešová, P. K., & Tremlová, B. (2021). Physico-chemical and melissopalynological characterization of Czech honey. Applied Sciences, 11(11), 4989. https://doi.org/10.3390/app11114989
- Rehman, M. U., & Majid, S. (2020). Therapeutic applications of honey and its phytochemicals. Springer.
- Ruoff, K., & Bogdanov, S. (2004). Authenticity of honey and other bee products. Apiacta, 38, 317–327.
- Saxena, S., Gautam, S., & Sharma, A. (2010). Physical, biochemical and antioxidant properties of some Indian honeys. Food Chemistry, 118(2), 391–397. https://doi.org/10.1016/j.foodchem.2009.05.001
- Seeburger, V. C., Shaaban, B., Schweikert, K., Lohaus, G., Schroeder, A., & Hasselmann, M. (2022). Environmental factors affect melezitose production in honeydew from aphids and scale insects of the order Hemiptera. Journal of Apicultural Research, 61(1), 127–137. https://doi.org/10.1080/00218839.2021.1957350
- Seisonen, S., Kivima, E., & Vene, K. (2015). Characterisation of the aroma profiles of different honeys and corresponding flowers using solid-phase microextraction and gas chromatography-mass spectrometry/olfactometry. Food Chemistry, 169, 34–40. https://doi.org/10.1016/j.foodchem.2014.07.125
- Singh, G., Passsari, A. K., Singh, P., Leo, V. V., Subbarayan, S., Kumar, B., Singh, B. P., Lalhlenmawia, H., & Kumar, N. S. (2017). Pharmacological potential of Bidens pilosa L. and determination of bioactive compounds using UHPLC-QqQLIT-MS/MS and GC/MS. BMC Complementary Medicine and Therapies, 17, 1–16.
- Soares, S., Amaral, J. S., Oliveira, M. B. P. P., & Mafra, I. (2015). Improving DNA isolation from honey for the botanical origin identification. Food Control, 48, 130–136. https://doi.org/10.1016/j.foodcont.2014.02.035
- Soares, S., Amaral, J. S., Oliveira, M. B. P. P., & Mafra, I. (2017). A comprehensive review on the main honey authentication issues: Production and origin. Comprehensive Reviews in Food Science and Food Safety, 16(5), 1072–1100. https://doi.org/10.1111/1541-4337.12278
- Špánik, I., Pažitná, A., Šiška, P., & Szolcsányi, P. (2014). The determination of botanical origin of honeys based on enantiomer distribution of chiral volatile organic compounds. Food Chemistry, 158, 497–503. https://doi.org/10.1016/j.foodchem.2014.02.129
- Trinh, N. T. N., Tuan, N. N., Thang, T. D., Kuo, P.-C. C., Thanh, N. B., Tam, L. N., Tuoi, L. H., Nguyen, T. H. D. D., Vu, D. C., Ho, T. L., Anh, L. N., & Thuy, N. T. T. (2022). Chemical composition analysis and antioxidant activity of Coffea robusta monofloral honeys from Vietnam. Foods (Basel, Switzerland), 11(3), 388. https://doi.org/10.3390/foods11030388
- Uršulin-Trstenjak, N., Puntarić, D., Levanić, D., Gvozdić, V., Pavlek, Ž., Puntarić, A., Puntarić, E., Puntarić, I., Vidosavljević, D., Lasić, D., & Vidosavljević, M. (2017). Pollen, physicochemical, and mineral analysis of Croatian acacia honey samples: Applicability for identification of botanical and geographical origin. Journal of Food Quality, 2017, 1–11. https://doi.org/10.1155/2017/8538693
- Viuda‐Martos, M., Ruiz‐Navajas, Y., Zaldivar‐Cruz, J. M., Kuri, V., Fernández‐López, J., Carbonell‐Barrachina, Á. A., & Pérez‐Álvarez, J. (2010). Aroma profile and physico‐chemical properties of artisanal honey from Tabasco, Mexico. International Journal of Food Science & Technology, 45(6), 1111–1118. https://doi.org/10.1111/j.1365-2621.2010.02243.x
- Wetwitayaklung, P., Narakornwit, W., Sratthaphut, L., & Wangwattana, B. (2018). Physicochemical analysis and determination of diand tri-saccharide content in Longan, Litchi and Siam weed honeys of Thailand. Journal of Apicultural Research, 57(1), 175–185. https://doi.org/10.1080/00218839.2017.1411183
- Workman, D. (2016). Natural honey exports by country [WWW document]. World’s top export. URL https://www.worldstopexports.com/natural-honey-exporters/
- Yildiz, O., Gurkan, H., Sahingil, D., Degirmenci, A., Er Kemal, M., Kolayli, S., & Hayaloglu, A. A. (2022). Floral authentication of some monofloral honeys based on volatile composition and physicochemical parameters. European Food Research and Technology, 248(8), 2145–2155. https://doi.org/10.1007/s00217-022-04037-4
- Zarei, M., Fazlara, A., & Tulabifard, N. (2019). Effect of thermal treatment on physicochemical and antioxidant properties of honey. Heliyon, 5(6), e01894. https://doi.org/10.1016/j.heliyon.2019.e01894