Abstract
Six distinct “views” of river hydraulics are presented, beginning with what influences us today, given our shared history, to what we can learn from others as they tackled research topics in their day. Other views relate to how we regard rivers through laboratory studies, the computer screen or in nature at full scale, and to how we might view research problems in the future. Interspersed with these various views are 10 questions that are frequently asked of river engineers, along with some possible answers. In attempting to answer these particular questions, some insights gained from a career in river hydraulics research are offered as a guide to others, both in practice or academe, as they raise some further issues which are worth reflecting on. This paper is particularly aimed at emerging hydraulic researchers and young engineers who are embarking on a career in river hydrodynamics.
1. Introduction
We are all standing midstream in the river of knowledge, as Coleman Citation(2010) helpfully reminds us of in his review of fluvial morphology. Like water in a river, the knowledge we rely on flows from the past to the present, where our task is to add something useful to it today, before it flows on to future generations, who will interpret it and develop it yet further. Just as it is good sometimes to pause by a river, simply to enjoy it and take in the view, so it is appropriate for us to pause occasionally in our intellectual endeavours, to take stock of where our discipline has come from and to reflect on where it might be going to in the future. This paper is particularly aimed at emerging hydraulic researchers and young engineers who are embarking on a career in river hydrodynamics.
Some “vision” papers try to second guess developments, while others attempt to review the key elements of current understanding and only then to elucidate tentatively what might be. Two such comprehensive and insightful reviews worth reading are those titled: “Issues and Directions in Hydraulics” by Nakato and Ettema Citation(1996) and “Perspectives in Fluid Dynamics” by Batchelor et al. Citation(2003).
As part of the Hydraulics Colloquium in honour of Professor John F Kennedy, held at the Iowa Institute of Hydraulic Research in 1996, I had the privilege and task of attempting to sum up the issues raised concerning river mechanics. In doing this, I also presented 25 quotations from various reviews on hydraulic research over several decades. They are worth reading and passing on to students because, like epigrams and epitaphs, they encapsulate wisdom or insight in a few words. Here are just five:
(1) “Why has progress in river engineering been so slow? First recall that most river sedimentation research is carried out by geologists and engineers. By the very nature of its subject, geology is not predisposed to dramatic developments (although its parent subject, geophysics, is); and engineers are made inherently conservative by the consequences of mistakes in their subject. So it is, I believe, that sediment engineering has proceeded step by step, with successive generations happy to set their shoes in the larger footprints made by the handful of intellectual adventurers, enumerated above, who went before them; and content if they merely made slightly deeper imprint in the sand” (Kennedy Citation1989). | |||||
(2) “I strongly suspect, however, that early creative bursts of insight seldom occur when one is staring at equations, or experimental data, or computer printout. Instead, novel ideas seem to me to arrive at unexpected times, when one is working on a problem but not momentarily immersed in it. In my experience, new ideas most often come while I am hiking, gardening, fishing, showering, or engaged in some other seemingly unscientific pursuit” (Kennedy Citation1989). | |||||
(3) “The broadening of knowledge, like the introduction of new tools like numerical methods, results in specialisation of the profession which then implies a loss of general view of hydraulic problems. One consequence of specialisation is the widening of a gap between fluids research and the practice of hydraulic engineering. Closure of this discontinuity will require the efforts of both camps in a variety of ways. Research oriented professionals should devote more time to writing books and monographs that sort, collate, evaluate and translate into usable terms the relevant research results. Practising engineers should share their real-world experiences including technical failures with universities. This exchange has to become part of continuous education” (IAHR Citation1993). | |||||
(4) “Finally, the mathematical model referred to in the paper is based on the solution of Newton's second law of motion (developed in 1687), using the Taylor-Maclaurin infinite series (developed in 1715) and including the Darcy friction factor (developed in 1889) and Prandtl's turbulence theory (developed in 1925). Many of these equations and experimental observations had little relevance at the time. The mathematical model outlined herein has been applied to over 50 environmental impact assessment projects, both in the UK and overseas. It is therefore of fundamental importance that, within the profession, the value of basic original research must never be forgotten” (Falconer Citation1990). | |||||
(5) “Turbulent motions contribute significantly to the transport of momentum, heat and mass in most flows of practical interest and therefore have a determining influence on the distributions of velocity, temperature and species concentration over the flow field. It is the basic task of engineers working in the field of fluid mechanics to determine these distributions for a certain problem, and if the task is to be solved by a calculation method, there is no way around making assumptions about the turbulent transport processes. Basically this is what turbulence modelling is about: because the turbulent processes cannot be calculated with an exact method, they must be approximated by a turbulence model which, with the aid of empirical information, allow the turbulent transport quantities to be related to the mean flow field” (Rodi Citation1980). |
For the remaining quotations, together with details of the numerous issues raised concerning possible future directions for river engineering, see Nakato and Ettema Citation(1996) and Knight Citation(1996). There are, of course, many other books dealing with the fundamentals or specific aspects of flow in open channels, that contain excellent reviews of current knowledge, such as “Floodplain Processes” (Anderson et al. Citation1996), “Coherent Flow Structures in Open Channels” (Ashworth et al. Citation1996), “Mixing and Transport in the Environment” (Beven et al. Citation1994), “Turbulence in Open-Channel Flow” (Nezu and Nakagawa Citation1993), “Turbulence Models and their Application in Hydraulics” (Rodi Citation1980), to name but a few. Several IAHR reports (IAHR Citation1993, Citation1994, Citation1999) also embody research visions as do some specific journal papers (Kennedy Citation1983, Citation1987, Citation1989, Elder Citation1986). Although it is clearly beyond the scope of this paper to give a comprehensive list of references, a selection of papers has been made, some to reflect the variety of subject matter, some for historical perspective, and some for whetting the appetite of the reader or to illustrate a particular point in the text.
2. A view of the upstream boundary conditions
Following the theme of a river of knowledge, it is obvious that the upstream flow and boundary conditions affect us, conditioning our understanding of river mechanics and in some cases polluting it. For example, our own understanding today may become clearer by some ground-breaking piece of mathematical analysis, or alternatively possibly muddied by poor experimental data and incorrectly drawn conclusions. A good understanding of the literature is therefore essential, not only for the academic, but also for the practicing engineer, who may use software based on advanced theoretical concepts. Two of the common weaknesses I have encountered over my career in candidates for PhD examinations are: (1) their lack of appreciation concerning the historical development of certain fundamental concepts in fluid mechanics and (2) their reluctance to read any papers more than 25 years old, that is, before they were born. Undergraduates likewise are often not taught about the philosophical development of ideas in fluid mechanics, which is especially remiss, given a subject abounding in fascinating history, personalities and conflicting opinions. See, for example, the exchange of letters between Osborne Reynolds and Sir Gabriel Stokes, given by Allen Citation(1970) in the book about Osborne Reynolds by McDowell and Jackson Citation(1970), concerning the relative importance/superiority of mathematics over experimental coefficients in engineering (or vice-versa), which is as topical today as it ever was over 100 years ago. Froude's early retirement at the age of 36 and his tenacity in building a 76 m long towing tank near his home to test the use of scale model ships is also instructive. His subsequent arguments with senior naval and government “experts” in the Admiralty show that it is not always easy to explain new developments to those who are unreceptive to new ideas. But who now thinks it unusual to use scale models to determine the optimum hull shapes or wave resistance for boats, as evidenced by tests on military ships or yachts for the America Cup race? Stories about people will always amuse undergraduates, as will anecdotes. Classical fluid mechanics and hydrodynamics is a rich seam with which to entertain and interest students of any age. As well as teaching the history of the development of hydraulic concepts (Rouse and Ince Citation1957, Rouse Citation1976, Citation1978, Citation1980, Hager Citation2003, 2009, Viollet, Citation2007), students should be introduced to the philosophy of science as part of a sound education as well as how scientific ideas interact with the political process.
One example that illustrates the usefulness of background reading for students is that related to the well-known Colebrook–White equation. Although Professor White's output in published work was minimal (only 20 papers in 40 years), his research activities were wide-ranging, influential and long lasting. His research was based on fundamental theoretical ideas, good conceptual thinking, backed up by detailed experimental work, all undertaken with exquisite attention to detail, followed up by the formulation of the results into a dimensionally correct equation after suitable validation studies had been undertaken. In that era, basic knowledge of fluid flow behaviour concerning boundary layers and turbulence may not have been as extensive as it is today, but researchers then were well grounded in the fundamentals of fluid flow behaviour, theoretical approaches and assumptions. They also knew well the history of their subject and previous key works. White well knew that the Nikuradse roughness length is only one parameter to consider when dealing with roughness, as highlighted further by Morvan et al. Citation(2008) in recent times. His earlier experimental work, as indicated by Davies and White Citation(1929), Pendennis-Wallis and White Citation(1939), Colebrook and White Citation(1937), pursued many details of secondary flows, curvature and types of roughness, all adding value to the final paper written by his associate (Colebrook Citation1939) in which the well-known formula appears. If only young undergraduate and postgraduate students of today took as much care about the historical context of their work, especially its philosophy and implicit assumptions, and took as much care over their experimental work, just as Professor White did, then we might have more productive research output today. Other examples that might be cited are the early works of Hele-Shaw (Citation1899, Hele-Shaw and Hay Citation1900), which contain such exquisite detail and photographs that would be exceedingly hard to improve on today. The reasoning behind the development of well-known equations is another incentive to read original works, rather than a later second-hand “explanation” of them. I wonder how many students have ever consulted the original paper by Chezy in 1768 or looked up what Manning actually wrote in 1889, let alone read Yen (Citation1991)?
Different types of publication should also be appreciated. Short papers, often proposing a new idea, are particularly useful as they indicate the first fresh description of a phenomenon that may be of lasting value. The paper by Einstein and Li Citation(1958), describing secondary currents in straight channels is one such example of a brief informative nugget, whereas the later much longer paper by Perkins Citation(1970) is invaluable reading as a follow up. Academic papers such as Grass et al. Citation(1991), which at first sight might not seem to have much relevance or impact on practical issues, should likewise not be ignored, as also ground-breaking work by many PhD students, for example, Tehrani Citation(1992), to name one among many that could be mentioned. Picture books of fluid flow are also extremely useful, as illustrated by the one on “Visualized Flow” by the Japan Society of Mechanical Engineers (JSME Citation1988). As always, knowledge acquisition is a time consuming task, but thankfully now much simpler with tools like Google Scholar than it used to be. A regular habit of reading the contents pages of journals, or occasionally browsing through the stack rooms of libraries still yields surprising gems, often in a serendipitous moment, and helps to protect against the dangers of the specialization/broad understanding problem raised in the third quotation of the introduction.
3. A view from the river banks
It is often said that river engineering is an “art” as well as a “science”, and that modelling should therefore take into account two distinct elements: “theoretical fluid mechanics” and a multitude of “practical issues”. This is illustrated by , taken from Nakato and Ettema Citation(1996), in which river engineering is envisaged as the joining together of two river banks, one named “theoretical fluid mechanics” and the other “practical problems”. On the left hand side (looking downstream), the shapes of the zones represent various practical river problems and their inter-related nature. The idea for this came to me after seeing the sketch by Lighthill Citation(1966) in which the various types of waves in liquids and gases were displayed in a similar manner, with the shapes and positions, reflecting particular wave characteristics and possible interactions with their neighbouring phenomenon.
Figure 1. The art and science of river engineering (after Knight) (reproduced from Nakato and Ettema Citation(1996), page 448)
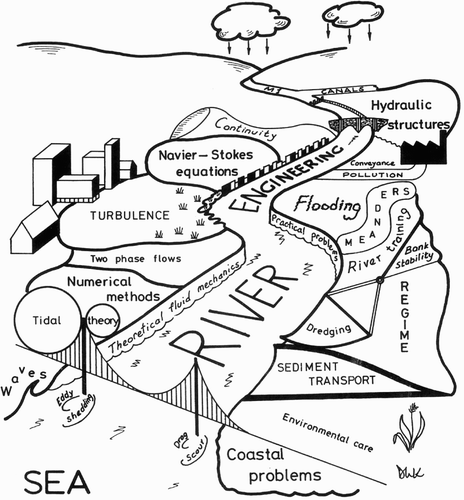
To deal with a specific practical issue in river engineering, one has to understand its relationship with other problems, gain that knowledge, cross the river by one of the bridges, pick up the appropriate theoretical knowledge from the right hand bank (again looking downstream), return by another bridge and deal with the particular practical problem or issue in question. In doing so, one may have to repeat the journey several times, or recollect previous journeys, thus slowly building up a more complete picture of the river system itself. The two river banks should not therefore be regarded as opposites, but as complementary. Sometimes a false dichotomy develops between people who tend to inhabit one side of the river or the other, perhaps stereotyped by the outdated “division” between practitioners and theoreticians, each regarding the other side as being either “too academic”, “too simple” or maybe a worse epithet. However, rivers always have two banks, and the most fruitful advances in river engineering have often occurred when the two sides meet, exchange views and deal with common issues.
It should be noted that the various areas of theoretical knowledge also have regions too. The river bank alongside the continuity and Navier–Stokes equations has sheet piling, indicating that the bank is firm and well established. It has been there since 1845. However, the sheet piling barely extends into the turbulence region, where the ground appears to be marshy and not so well developed. The high rise buildings nearby indicate enormous growth, activity and development in this region, as typified by the growth in the subject of computational fluid dynamics and advanced experimental studies in turbulence. A deeper understanding of turbulence is, however, for future generations to achieve.
Although it is said that a sketch is worth a thousand words, visual images can create lasting impacts, so it is important to recognize that a poor drawing of a fluid flow phenomenon can solidify ones thinking into believing that it is a true representation of reality. An example of this, drawn from the author's experience, is described later. Likewise, a common danger in interpreting model output is to believe that the visualizations (e.g. vector plots, velocity fields, etc.) are a true representation of the physical process. What is seen can be an artefact of the visualization algorithms and not a true reflection of the solution of the numerical procedures in the hydrodynamic simulation. For example, post-processing of raw outputs may have smoothed results or introduced false flow structures. There is sometimes a tendency for users to give greater weight to more detailed pictorial output from a two-dimensional (2D) or three-dimensional (3D) model, than from a one-dimensional (1D) model or measurements. Having now mentioned models, it is appropriate to consider briefly the impact of computer modelling.
4. A view from the computer screen
Mathematical models that deal with whole catchments, combining hydrological models with hydraulic models of flows in individual rivers, have changed fundamentally the way river engineering is now practiced compared to say 50 years ago. Flood risk management, eco-hydrodynamic and geomorphic studies have all been transformed by the development of powerful numerical tools and techniques that solve the governing equations. The process of obtaining the most significant concepts, through model building to achieve particular objectives, calibration and commercial exploitation is complex, as anyone knows who has attempted it. It is possibly worthy of a review in its own right. Some experiences of this process are described in Abbot and Basco Citation(1989), Abbot et al. Citation(1991), ASCE (Citation1988), Cunge et al. Citation(1980), Knight et al. Citation(2010a), Knight (Citation2008, Citation2013), Mc Gahey et al. Citation(2008) and Nakato and Ettema Citation(1996).
In dealing with complex technical issues, and particularly when trying to explain an issue to government officials or politicians, it is a good practice to pose the issue in the form of a simple question with the key technical issues highlighted immediately afterwards. This undoubtedly helps them to understand unfamiliar material and, hopefully, to go on to appreciate the relevance of the scientific issues raised. This approach is now used here and in subsequent sections to illustrate several important hydraulic issues that arise in river engineering. In all, some 10 questions are posed.
Question 1 – What level of modelling is generally required in river engineering?
Key issue: appropriate model selection and correct application
• | There is generally a range of models available. It is essential that the user is able to select the best model for each application and is aware of the shortcomings and uncertainties of the chosen method. | ||||
• | Correct model selection and application will pay for itself many times over in terms of improved accuracy and certainty in the results. |
The river engineer has to select the right tool, for example, the appropriate 1D, 2D and 3D model, for any particular job, and this demands considerably more knowledge and understanding than was required in the past. Today, the questions that are likely to be asked are: When should one adopt a 2D depth-averaged numerical model to represent the flow physics, rather than a 1D model? When one uses a 3D model, what type should it be and what level of turbulence closure is appropriate? The experience of the modeller in using 2D and 3D models is now also important, as different answers will be obtained by different users, not only due to subtle differences in model type and calibration requirements but also according to what type and version of commercial software is used. Some background is provided in Abbott et al. (1991), in previous reviews (ASCE Citation1988) and in IAHR guidelines (IAHR Citation1994).
Depth-averaged models are commonly used in practice. However, depth-averaging of velocities in rivers, especially in sinuous and meandering channels, may lead to false representation of actual flow patterns. For example, where the velocity vectors vary over the depth, depth-averaging in a preferred direction will give misleading results, especially so when used to calculate the discharge. This is commonly seen in flood conditions at the cross-over region of a meandering channel, where the upper flow from the floodplain interacts with the flow below the bankfull level in the main channel. The former is predominately in the valley slope direction whereas the latter is predominately in the channel streamwise direction. Thus, in some circumstances, a 3D model might be preferable, and so one has to ask at what stage should a 3D model be considered? The use of hybrid models, such as a 1D river model with a 2D floodplain model, also raises a number of technical issues.
It is worth noting at this point that higher dimensionality of a model does not necessarily lead to better accuracy in the results. In certain cases, the opposite may be true. The principle of Occam's razor should always be applied – that of starting with the simplest by assuming the least (Pluralitas non est ponenda sine necessitate; “Plurality should not be posited without necessity”, William Occam, 1285–1347). The principle gives precedence to simplicity; of two competing theories, the simplest explanation of an entity is to be preferred. In the context of modelling flows in rivers, this suggests caution before embarking on 3D modelling for solving every type of river engineering problem. It should be remembered that very often simple tools are still used to craft works of art and beauty. Just consider what can be done with a paintbrush, chisel and tape measure. It is the skill to which they are put that portrays the “usefulness” of the tools.
As regarding accuracy and certainty, at least three points should be kept in mind, discretization, averaging and equifinality. Morvan et al. Citation(2008) write that
Roughness appears in fluid mechanics as a consideration at wall boundaries, to account for momentum and energy dissipation that are not explicitly accounted for in the simplified or discrete formulae used in numerical engineering and science. In this way roughness is a model of the physical processes that are omitted. There is indeed no need for such an artefact in the continuum mechanics Navier–Stokes (NS) equations for laminar flow, or in the Reynolds Averaged Navier–Stokes (RANS) equations for turbulent flow, since all momentum and other energy losses – such as turbulence, shear, drag force, etc. – are implicitly contained in the equations. The issue only arises when the exact form of the equations needs to be simplified, as for example in discretisation purposes in three-dimensional (3-D) models, and for discretisation and conceptual reasons in 2-D and 1-D models. In the latter, the definition of the so-called ‘roughness’ or ‘friction factor’ becomes more uncertain, and therefore less rigorous in terms of definition and sizing, than it does for 3-D models, although it must be said that it does not account for the same thing as in 3-D models.
Then, Nikora Citation(2008) raises the question whether the Reynolds-averaged Navier–Stokes (RANS) equations, based on temporal averaging, should be the default starting place for all problems, or whether those based on spatial averaging might not be more appropriate. Indeed, one might question why one starts with a numerical approach at all, involving a discretization process and advanced numerical techniques, when it might be worth the effort to obtain an analytical solution to the governing equation, or a simplified form of them.
Third, a particular difficulty arises in models discretized into cells or panels (for depth-averaged models), where several parameters are used to simulate certain physical flow mechanisms in each cell/panel. This raises the issue of equifinality (Aronica et al. Citation1998). There is usually a lack of sufficiently comprehensive data from which to select such parameters and hence to calibrate the model without ambiguity for practical use. For example, in the Shiono and Knight Model (Shiono and Knight Citation1991, Knight et al. Citation2010a,Citationb), the choice of the three calibration parameters (f, λ and Γ) for each panel is fraught with difficulties due to lack of measured turbulence data for flows in even generic-shaped prismatic channels. Until such work is undertaken, this will inevitably limit the application of this type of model. Recent work by Sharifi et al. (Citation2009, Citation2010) on the application of evolutionary computation to open-channel modelling, and by Chlebek and Knight Citation(2006), indicate that it is possible to investigate numerically the physical parameters more thoroughly than hitherto.
Question 2 – What are the appropriate calibration parameters for use in 2D and 3D models?
Key issue: model calibration
• | 2D and 3D models can provide much improved flow and level predictions in many cases, for example on floodplain flows. However, there are issues in model calibration that need resolving. |
The use of 2D and 3D models poses particular difficulties with respect to calibration. Not only does the level of turbulence closure and the values for many turbulence coefficients have to be specified, but also the time and effort spent on data handling increases proportionately. Furthermore, there will often be a lack of any appropriate field data for suitable verification, for example, Reynolds stresses, turbulent intensities, secondary flows, vortex behaviour, time averaged values of fluxes for use in the RANS equations, etc. Although it is commonly thought that by increasing the dimensionality of the model the answers might be more “accurate”, this is not necessarily so, since in 2D and 3D models, there are many more factors that need to be considered. Many fundamental hydraulic parameters are at present unknown, or in the natural river not easily measurable in sufficient temporal and spatial detail, to make the application of a 3D model sensible.
This means that approximations are often made in order to produce simpler models with specific objectives, for example, flood routing, and for predicting stage–discharge relationships, sediment or pollutant transport rates. Any such approximations highlight the need to understand the assumptions implicit in a given model and to know the limits of its application. This is illustrated later in Section 5 by reference to a simple lateral distribution model, for steady flows in prismatic channels, aimed at predicting the lateral distributions of depth-averaged velocity and boundary shear stress. However, it is worth re-iterating that when dealing with many practical river issues, steady flows very often do still need to be analysed (e.g. when estimating conveyance capacity or in extending stage–discharge relationships), that river reaches or channels are often treated as being prismatic (e.g. reaches near weirs or gauging stations and canals), and that knowing the distributions of depth-averaged or velocity boundary shear stresses across a channel are useful in vegetation and sediment studies.
Question 3 – What is the role of computer software in learning about river engineering?
Key issue: acquiring knowledge of fluid flow phenomena
• | Useful in understanding certain issues, either through numerical experiments or through applying a dedicated model to a case study. Both require a model for repetitive calculations in order to investigate physical effects, boundary changes or calibration techniques. | ||||
• | Helpful in understanding fluid flow concepts through flow visualization of velocity or turbulence data, showing videos of laboratory or natural phenomena, and transmitting teaching notes with embedded pictures, graphs and comments, maybe on some rare and unusual events. |
Many textbooks on fluid mechanics, computing and thermodynamics now come with a CD at the back containing numerical programmes for analysing certain problems, together with teaching materials for learning about advanced techniques in numerical analysis, data processing and modelling. These ready-to-use materials, as well as what can be downloaded directly from the web, are possibly akin to what one buys from the fast-food counter of a supermarket – handy, processed and convenient, but not to be regarded as the only source of nutrition. One needs a balanced diet, with opportunities to work through simple problems by oneself step-by-step, for example, stability of numerical procedures as in Abbot and Basco Citation(1989), statistical quirks, simulation of stage–discharge curves, plotting of isovels, etc.
One pioneering teaching material, developed by Ligget and Caughey Citation(1998), is called “Fluid Mechanics: an interactive text”, which enables the student to learn not only about the basics of turbulence, stream functions and open-channel flow, but also to undertake examples with its embedded Matlab routines. The early years of the Flood Risk Management Research Consortium (FRMRC) also developed teaching materials for undergraduate use, based on commercial software and practical examples. These may be found at www.floodrisk.org.uk and http://frmc.hw.ac.uk. Likewise, the recent conveyance estimation system (CES) may be used to investigate roughness issues and uncertainty in predicting stage–discharge relationships. The CES features in the Conveyance and Afflux Estimation System software, which is freely available at www.river-conveyance.net. The trend in the use of computer-based models will increase and already most commercial codes produce cut-down versions that are suitable for student tuition. The cost implications are no doubt a factor, but introducing students to a well-respected brand name makes long-term commercial sense. Is there a role here for the IAHR to develop instructional tools, in a similar way to it making a library of pictures of hydraulic phenomena available to students?
However, learning from a computer screen is only one way of acquiring knowledge, as theoretical knowledge learnt in the lecture room or from a book needs the learning experience of the laboratory as well. I always try and ensure that PhD students who mainly pursue numerical studies spend some time with another student whose work is mainly laboratory-based (and vice-versa), so that they all avoid the dichotomy illustrated in . Although this is more difficult to maintain today, as specialization increases, it is worth attempting. It is therefore expedient to move on from what some call “virtual hydraulics” to take a view of “actual hydraulics”, as maybe acquired in the laboratory or from fieldwork.
5. A view from the laboratory
Undergraduate students at Birmingham undertake several hydraulic experiments in their first semester, one of which involves using Ahlborn visualization tanks with rigid and mobile beds. They are provided with various objects and thin flexible plates, together with powder and paint, and asked to see what they can learn about fluid flow behaviour involving flow separation, vortex shedding, horseshoe vortices, boundary layers, contraction effects, breaching dynamics, dam failures, etc. Since by this stage they have had virtually no formal tuition in fluid mechanics, this can be an advantage, as they can make up their own minds and try and understand what they see with their own eyes. Someone is always on hand with short “guidance notes” on what they might do, as well as to stimulate questions, offer help when required, and interact as a tutor. The aim is to get them to observe closely, attempt to draw what they see, and subsequently produce a report with sketches and seminal concepts, based on their own ideas. This particular experiment is always popular, but incidentally teaches them how difficult it is to capture a particular fluid flow phenomenon in a drawing or sketch, especially in the manner of Leonardo de Vinci. You can often tell from illustrations of fluid phenomena in textbooks, whether the author has actually drawn it from experience or simply used a copy artist.
At the postgraduate level, many papers in research journals highlight the importance of well-focused experimental studies to elucidate key hydraulic phenomena that are not readily amenable to investigation by purely analytical or numerical models on their own. The availability of experimental facilities, as well as the staff who work in the laboratories should never be taken for granted. Indeed many laboratories in our universities, as well as at national level, are under threat today due to the high costs involved in running them. This is a particularly worrying trend, as now is just the time when, with advanced turbulence modelling being used to an ever increasing extent, there is an even greater need than ever before to undertake well focused and imaginative experiments to provide data with which to improve or validate such models. This regrettable trend in shunning basic experimental work is highlighted by Nakato and Ettema Citation(1996) on pages 450–459. Having studied for my PhD at Aberdeen University, under Professor Jack Allen, who pioneered the use of scale models in the UK (Allen Citation1947), I have always enjoyed experimental work, for which I am grateful, as it has stayed with me throughout all subsequent numerical and analytical research work.
In my research career involving studies in estuary dynamics, sediment mechanics, boundary shear stresses, overbank flow and floods, I have selected just one topic to illustrate what might be gained by a view from the laboratory. This topic all began with a diagram in Chow Citation(1959), in which the distribution of boundary shear stress in a prismatic channel was given. At the time I was involved in resistance and sediment studies, involving the well-known shear velocity u *=(τ o /ρ)1/2 that featured in many equations. When I asked others about the whereabouts of the data for τ o in such a diagram, I received mainly blank looks followed by a long silence. So I began searching for the evidence, and finding only a limited amount, decided to start investigating it myself. It is always wise to inspect data oneself, and not to rely on second-hand knowledge or what people say. I will now consider a small part of this topic, how the lateral distribution of boundary shear stress varies in open-channel flow.
A typical distribution is shown in , for inbank flow in a trapezoidal channel, one of the simplest shapes used in river engineering. It is but one set, taken from hundreds of experiments in prismatic channels and closed ducts of various shapes, aimed at trying to understand this phenomenon. This topic proved to be very useful in acquiring appropriate skills in new experimental techniques and error control as well as improving my education and understanding of secondary flows, planform vorticity, and the fundamentals of boundary layers, all of which were useful in other research topics.
Figure 2. Flow parameters measured in a trapezoidal channel (after Knight et al. Citation2010a)
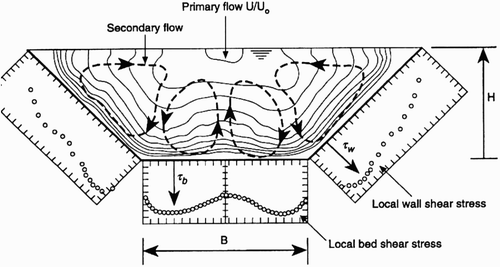
shows some isovels and four dominant secondary flow cells for a particular aspect ratio, B/H, together with measured boundary shear stresses on the channel walls and bed. shows part of one set of experiments in which the Froude number, F , was held between 0.48 and 0.59 and the aspect ratio varied from 1.0 to 10.0. The distributions differ with B/H, and depend on the number and positions of the secondary flow cells. The wider the channel, the more uniform is the distribution of boundary shear stress in the central region, as would be expected. shows a corresponding set, this time for supercritical flows with F =3.2 and aspect ratios between 1.5 and 5.3. The significant influence of secondary flows and aspect ratio on the distributions may again be seen.
Figure 3. Boundary shear stresses measured for inbank subcritical flows in a trapezoidal channel for aspect ratios between 1 and 10 and F ≈ 0.48–0.59 (after Knight et al. Citation1994)
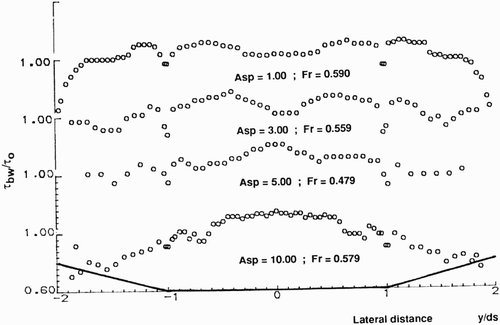
Figure 4. Measured boundary shear stresses for inbank supercritical flows in a trapezoidal channel for aspect ratios between 1.5 and 5.3 and F ≈ 3.2 (after Knight et al. Citation1994)
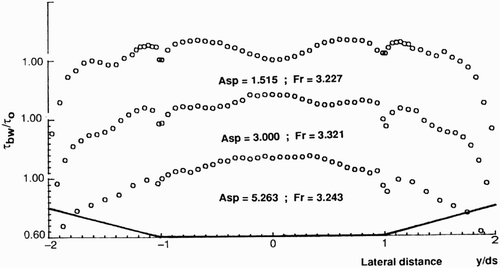
illustrates the integrated values of wall shear stresses, expressed in terms of a percentage of the total shear stress carried by the channel, plotted against the ratio of wetted perimeters for the bed and walls, P b /P w , rather than in terms of aspect ratio, B/H. This then combines data from rectangular and trapezoidal channels with differing side slopes. The plot contains a mixture of open channel and duct data, as well as data from smooth and uniformly roughened channels. There are differences between open channel and closed ducts, too small to see in this figure, but noticeable in a more detailed analysis of free surface effects. For further details, see Knight et al. (Citation1992, Citation1994) and Rodi Citation(1980).
Figure 5. Measured percentage boundary wall shear force for inbank flows in a trapezoidal channel for wetted perimeter ratios between 0 and 50 (after Knight et al. Citation1994)
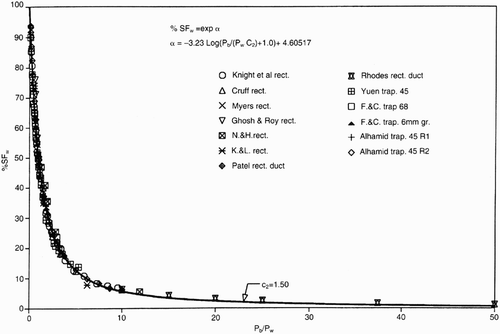
shows how an empirically fitted equation to can be used to predict the mean bed shear stress in trapezoidal channels, with varying side slopes, for both subcritical and supercritical flows. Experiments show that % SF w reduces slightly with F , and this may need to be taken into account when dealing with very high speed flows, such as occur in some flood-water release tunnels at high dams where velocities may exceed 60 ms−1. Cavitation effects then need to be considered. Data from channels in which either the walls or bed are roughened differentially from each other are given by Knight et al. Citation(1992). Such an empirically derived % SF w v P b /P w equation can also be used to examine standard sidewall correction procedures, as shown by Chlebek and Knight Citation(2006). These figures serve to illustrate how a piece of basic research in one topic aids another topic, such as sediment transport, in which flume studies involving composite roughness feature strongly. in Section 2 has already drawn attention to the inter-related nature of topics in river engineering.
Figure 6. Measured mean bed boundary shear stresses for flows in trapezoidal channels with different side slopes (after Knight et al. Citation1994)
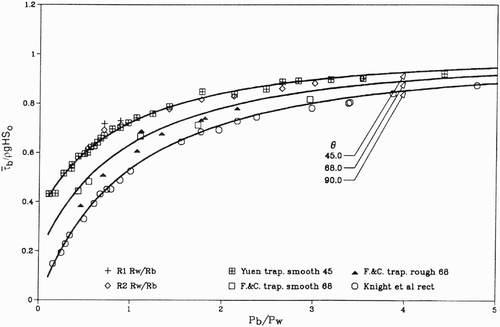
might appear to reinforce the widely held opinion that the “view from the laboratory” is at “too small a scale” and is based on flows in “too idealized conditions”, that is, being conducted in straight flumes with only simple shapes for cross-sections. is, therefore, inserted as a reminder that natural river channels are usually neither prismatic, nor composed of bed with a uniform roughness distribution over the wetted perimeter, even for inbank flows. The interpretation of laboratory data, and the application to natural rivers of any subsequently derived equations, is however problematic.
Figure 7. Concepts to be considered for inbank flows in natural channels (after Knight and Shiono Citation1996)
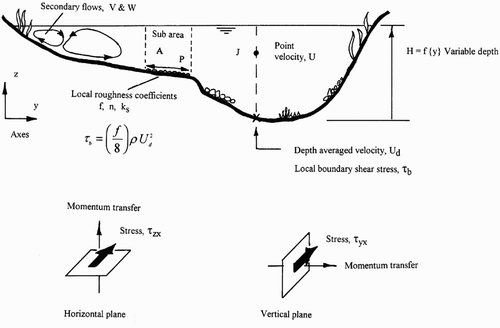
The same is true for overbank flows, where the flow concepts are more complex than for inbank flows, as indicated in . Typically, there is a strong lateral shear between the faster moving flow in the deeper main channel and the slower flow in the shallower floodplain region. The shear layer produces large-scale planform vortices at the floodplain edge, shown by a series of vortices at the interface between the floodplain and main channel, rotating around vertical axes. These should be distinguished from the streamwise vortices, drawn within the main channel, which are driven by the differential gradients in turbulence intensities and rotate around horizontal axes. Both types of vortex are generally present in many flows, but in overbank flow, one form may dominate another depending on the value of the ratio of floodplain depth to main channel depth, Dr. For Dr<0.3, the planform vortices are large flow structures with low frequency, extending over the entire width of the floodplain, even in natural rivers. For higher relative depths, that is, Dr>0.4, the streamwise vorticity at any re-entrant corner tends to dominate the flow at the floodplain/main channel interface, making the planform vortices much smaller, as drawn in . One should not, therefore, take this sketch at face value as being representative of all types of floodplain flow, as some authors do when copying this diagram. The planform vortices are drawn relatively small here to indicate higher depth flows. This highlights the point made earlier about “solidifying” false concepts through sketches. Alternative sketches of this 3D phenomenon are given by a number of other authors, such as Fukuoka and Fujita Citation(1989) and van Prooijen et al. Citation(2005). A fuller explanation of the interaction between the vortices, with photographs is given by Knight and Shiono Citation(1996), Knight Citation(2008), Chapter 17 of Knight and Shamseldin Citation(2006) and in Chapter 2 of Ikeda and McEwan Citation(2009).
Figure 8. Concepts to be considered for overbank flows in prismatic compound channels (after Shiono and Knight Citation1991)
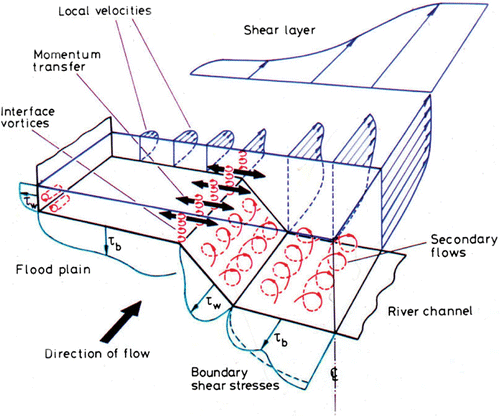
Mention should also be made at this point about full-scale observations and measurement of flow structures in the field. My own personal view of hydraulics and fluid mechanics has benefitted from observing and measuring various mixing, resistance, sediment and turbulence phenomena in various estuaries and rivers. Finally, one question that is still asked with surprising regularity, usually by senior finance officers worried about the cost of maintaining experimental facilities, is the following:
Question 4 – Why are laboratory experiments still needed when computers can do it all?
Key issue: without laboratory-based research, we cannot validate models effectively
• | Laboratory studies sometimes provide the only way of gaining insights into the behaviour of complex flow patterns under controlled conditions, thereby enabling theoretical concepts to be validated and numerical models to be developed securely. | ||||
• | Laboratory research often provides the primary data on many empirical coefficients used in turbulence models, without which no closure is possible. |
, and 8 have shown that flow in relatively simple shapes of prismatic channels is more complex than many people imagine. Modelling such flows is not easy and far less straightforward than modelling stresses in solid mechanics. Indeed, turbulence has often been described as “the outstanding difficulty of our time”. We simply do not know enough about it, or really what turbulence is, given the many types of flow structure that are implicit in many flow problems (Lighthill Citation1970). For example, the relationships between turbulent intensities, Reynolds stresses, secondary currents, flow structures and boundary shear stress have not been comprehensively measured yet, even for flows in simple channels, such as the trapezoidal one shown in .
If such data were available for uniformly roughened channels, over a wide range of aspect ratios, these would provide a sound basis for many design scenarios and research studies. Further measurements in non-uniformly roughened prismatic channels, and after that in channels of other shapes, would enable models to be benchmarked properly against agreed data sets for certain commonly used generic shapes. Ultimately, even more data should be obtained for non-prismatic channels, involving various planform geometries, with the effects of sediments and vegetation added in as well. Clearly, such a data acquisition programme would be an enormous undertaking, but until it is done, no turbulence model of river flow can be said to be properly validated. This is but one example of where laboratory-based research is needed, where controlled conditions are possible and measuring facilities available.
These data would then become the basic building blocks for future engineers to benefit from, without having to rely on partial data sets from individual experiments, written up in numerous papers, often conducted with differing objectives, and not necessarily aimed at this fundamental task. It is what might be regarded as an essential preliminary 3D programme of work, similar to that undertaken so painstakingly to understand 2D wall-governed turbulence and boundary layers. Consider what effort has gone into investigating hairpin vortices, through to the viscous sub-layer, buffer and logarithmic layers to the wake zone, all carried out under varying pressure gradients, to be followed up by the theoretical development of displacement thicknesses, thin shear layer equations, and so on. We now benefit from previous decades of such work. Similar work is now needed for 3D flows in open channels.
6. A view from under the surface
The “views” given in the preceding sections, taken from different vantage points, now need to be consolidated by asking some further questions concerning practical river engineering issues. To illustrate this, I select another topic that interests me, namely floods. Flood disasters account for about a third of all natural disasters (i.e. floods, earthquakes or storms) world-wide, but are responsible for over half the deaths. For an introduction and insight into recent flood-related research and some case studies, see Berz Citation(2000), Knight et al. Citation(2006), Knight and Samuels Citation(2007), Miller Citation(1997) and the FRMRC website (www.floodrisk.org.uk and http://frmrc.hw.ac.uk).
Question 5 – What is wrong with our estimates of flow and level, and what will make them better?
Key issues: extreme flows, composite roughness and hydraulic resistance
• | There is high uncertainty in flood levels for extreme flows predicted by models. One reason for this is that models use simple roughness parameters, often only calibrated for lower flows. | ||||
• | There is uncertainty in deriving “design” flood flows in hydrology for a given return period or frequency, because of poor understanding and extrapolation of stage–discharge relationships. | ||||
• | Care should be taken to distinguish between roughness and hydraulic resistance. |
Discharges and water levels are the primary parameters of interest in flood forecasting, flood risk management, floodplain modelling, river rehabilitation and geomorphological studies. At present, there is considerable uncertainty about our estimates of high flows, whether it is based on our present gauging methods and technology, or based on our predictions from mathematical models of the hydrodynamic processes. The fundamental link between discharge and water level is important, since flood risk maps should give an accurate estimate of water levels and inundation areas as possible, as these are used in econometric models to quantify flood damage costs, in planning emergency response strategies and in flood alleviation design studies. Determining the stage–discharge relationship for channels with complex cross-sections, non-uniform roughness distribution and in unsteady flow conditions is technically challenging and not a simple matter, as indicated by Ramsbottom and Whitlow Citation(2004), Knight and Shamseldin Citation(2006) and Knight et al. Citation(2010b). The CES website www.river-conveyance.net also contains many helpful details.
Hydraulic resistance coefficients need to take into account the interaction between turbulence generated by surface roughness, local topographical features and large-scale flow structures. Values of the coefficients for the local surface roughness should not be arbitrarily increased to account for these other effects. In practice, this may mean distinguishing between friction, energy and water surface slopes in 1D river models and taking care in 2D and 3D models over the use and spatial distribution of “sink” terms and interfacial stresses between cells or panels. Equations dealing with “patchy” roughness, composite and non-uniformly distributed roughness should also be examined carefully before incorporation into models, as well as those dealing with vegetation or sediment bed forms. Boundary zones between areas of different roughness may create particular boundary-related secondary flows, as shown by Ikeda Citation(1981) and Tominaga and Nezu Citation(1991).
For reliable predictions of stage–discharge relationships, the modeller needs adequate turbulence data such as Reynolds stresses (in order to obtain boundary shear stresses in particular zones) and knowledge of large-scale flow structures (eddies, shear layers and mixing zones). The common practice of reliance on a piece-wise rating relationship, as recommended in the International Standard ISO 1100-2 Citation(2010), is not suitable for modern hydraulic analyses, where the whole HvQ relationship should be understood in terms of the flow behaviour, and related to topography, fluid mechanisms, resistance and driving forces. It is only then possible to extend the HvQ relationship rationally, and in a manner that is easily transferable to other modellers, or hydrometric staff, building up progressively a more comprehensive understanding as more data become available. In river work, we should be aiming at measuring what is needed, particularly that which is required for numerical models, and not what is easy or traditionally undertaken, often just velocities and water level. I suggest that there should be a flood plain handbook, along similar lines to the flood estimation handbook in the UK, in which all relevant hydraulic information is kept on HvQ data, roughness and turbulence data, as well as modelling equations for future practitioners to use.
Question 6 – What is the role of broad-scale modelling in catchment flood risk mapping?
Key issue: hydrodynamic modelling is only one aspect of catchment modelling
• | There is an important role for broad-scale models, making the best use of available technology, but recognizing the uncertainties associated with their application. | ||||
• | There is a need to integrate broad-scale models of different processes, hydraulic, hydrological, flood risk, socio-economic and biological, into a systems approach. |
River basin management considers flooding from the governing meteorological conditions and the point of impact of rainfall on the land to the discharge of the flood to the sea. It recognizes, evaluates and takes into account the human dimension as well as the technical and economic cases for intervention, and the environmental impact of these. River basin modelling is, therefore, not just about hydraulics or hydrology, but also involves social, economic and environmental considerations as well, as shown by the examples in the FRMRC programme (http://frmrc.hw.ac.uk and www.floodrisk.org.uk) and in several books on catchment modelling (Knight and Shamseldin Citation2006). When viewed from a catchment perspective, or indeed from a national flood risk mapping perspective, there is a clear need for models that are capable of producing results over a wide area, so-called “Broad-Scale” models. This need is further driven by directives from the European Community, such as the water framework directive (WFD) in 2000 and the flood hazard directive in 2006.
Risk is generally accepted to be the product of probability by consequence, and therefore both should be considered in models. In hydraulic terms, modelling discharge, local velocity and depth is routine, but with the concept of “flood risk for people”, what is required are combinations of depth and velocity to be evaluated concurrently. Recent work by Shu et al. Citation(2011) and Xia et al. Citation(2011), on determining the degree of hazard associated with particular depths and velocities in the devastating floods at Boscastle in the UK in 2004, has shown that the application of depth–critical velocity relationships for the stability of adults, children and partially submerged cars is useful in highlighting danger zones. Comparison of the predicted danger zones from the results of numerical models with video recordings of the actual movement of cars during the flood were favourable, indicating the effectiveness of such tools in planning and siting of car parks, as well as in undertaking risk assessments for people.
The Source–Pathway–Receptor (SPR) modelling of flood impacts and outcomes is frequently used to assess how the impact of local-scale changes in the runoff generation anywhere in the catchment (the Source) propagate through the river network (the Pathway) to affect flooding downstream (the Receptor). SPR impact decomposition mapping is the inverse problem in which one asks the question “How can downstream impacts of a flood (Receptor) be tracked back through the channel network (Pathway) to the local Source areas that created the impact?” This is but one example of where inverse questions could usefully be asked of most hydraulic models in order to apply them more effectively.
Question 7 – It looks good, but is it correct?
Key issue: accuracy and reliability
• | Outputs from numerical models often look impressive but the user must be able to assess whether they are correct, preferably by some independent means. | ||||
• | The performance of hydraulic models is very dependent on the quality and quantity of calibration data, and so there is a need to maximize the use of existing data and to understand what additional data should be acquired. |
How does one check the answers given by a numerical procedure or software package provided by another person or institution? As commercial software becomes easier to use with better user interfaces and “hidden” default strategies, questions arise about the technical value of such results and output. There is clearly an increasing need for independent checks to be made of model results. The more complex the model, the more difficult it becomes to comprehend the internal software algorithms used, the default values for coefficients and in some circumstances even the flow conditions to be modelled. Despite 3D colour graphics and virtual reality video images, there is a need to substantiate such output, both by the non-specialist user and by the technical expert. This is achieved traditionally by benchmarking the software results against field or laboratory data, against specific test cases for which there are analytical solutions, and in some circumstances by the use of alternative methods. Forensic analysis of model results may become a growth industry by “experts” in a court of law, but it is not for the faint-hearted.
Question 8 – Would an intelligent client save money by improving the job specification?
Key issue: understanding uncertainty and risk
• | Clients should be aware of the risks and uncertainties associated with different methods of hydraulic analysis in order to better assess and guide decisions. | ||||
• | Many client representatives have limited knowledge of hydraulics and rely on the judgement of experts or their consultants. |
Following on from Questions 4–7, there is a need for any client to appreciate what is involved in flood risk management modelling. For example, the specification for projects used by the Environment Agency (EA) in the UK are continually updated to reflect changes in project objectives, experience in the technical aspects (including feedback from consultants) and the improvements in techniques and in the data these techniques use. For these improvements to be maximized, the EA, or for that matter any client agency, need to ensure that its own internal hydraulic knowledge and its skill base are up to date. Large organizations, such as the EA, should not “outsource” all its modelling work, as this usually leads to a loss of scientific and engineering expertise and hence an ability to effectively engage with external consultants. Over a number of projects, investment in better specification, more intelligent modelling, and transparent technical logic, should save money and improve credibility.
Furthermore, the public is now much better informed about flood-related topics, and have access to data hitherto not previously available. Flood damage and insurance costs are now more of a “political” issue than they used to be, and river restoration projects have likewise heightened the public's awareness of the role of the river engineer. The public now demand of governing bodies a better quality of “service”, and so the modeller now has to act together with the public and governing bodies, in partnership, to solve local problems. In a litigious society, where conflict arises, the use of litigation may force all technical experts to review their capability in this area.
The role of the hydraulician in society also needs to change. Elder Citation(1986) stated that “Our most urgent professional need is to become involved with the issues of the day, not as another pressure group but as a recognised source of factual information, upon which the public can rely.” Whatever we may think of this statement, it should perhaps be debated by IAHR as part of its societal responsibility.
Question 9 – What is eco-hydraulics, and why is it important?
Key issue: the natural environment
• | There is a growing need to link hydraulics with ecosystems to ensure that hydraulically acceptable solutions are acceptable from an environmental viewpoint. | ||||
• | The requirements of the WFD and similar legislation demand our attention. |
There is a need to appreciate that the hydraulic behaviour of rivers is the key to ecological modelling. The depth of flow, velocity field, turbulence levels, substrate and vegetation within the river reach are all governed by the hydraulics. Indeed one might say that the biology and chemistry are driven primarily by the physics of the flow. The sciences of hydrology (catchment-scale), geomorphology, hydrodynamics and eco-hydraulics (catchment and reach-scale) describe the complex interaction between the flow characteristics within a channel, the sediment fluxes and the habitats. The flow characteristics determine the nutrients, oxygen levels, movement of sediment, the physical form of the channel bed and vegetation, all of which determine the nature of habitats.
Under the new EC WFD, there is a requirement to achieve good ecological status for all water bodies by 2016, with the exception of heavily modified water bodies. Hydraulicians must therefore broaden their outlook and to appreciate not only eco-hydrology (Zalewski Citation2011) and mixing zone regulations for effluent discharge (Bleninger and Jirka Citation2011), but also how modelling and monitoring towards “ecological good” status should be undertaken (Hartnett et al. Citation2011). These skills should be added to those traditionally attributed to them, such as understanding the basis of fluvial processes (Chang Citation1988), sediment transport and the principles behind regime theories (White et al. Citation1982, Bettess and White Citation1987), vegetation biotypes and resistance (Cheng and Nguyen Citation2011) and finally to the possible impact of climate change on all of these in the future (Bronstert Citation2006).
7. A view looking downstream
There is one final question that people regularly expect any author of a “vision” paper to tackle, and that is:
Question 10 – What will be the problems and novel applications in 10 or 100 years’ time?
Key issue: knowing the boundary between the unknown and the unknowable
• | Present research must fulfil the requirements of future practitioners. Likely, future needs must be kept under constant review and R and D programmes developed to match those needs. | ||||
• | It is not always sensible to try and second guess the future. |
The scientific basis of hydraulics and fluid mechanics is currently largely based on the RANS equations, and that is not likely to change for some time, despite the RANS approach averaging out large-scale motions that can be important (e.g. planform vortices in overbank flow flood studies). The use of large eddy simulation models in river engineering is likely to grow in the future, albeit slowly. Direct numerical simulation models are probably never likely to be used in practice for decades, if ever. Non-deterministic models and new probabilistic models, based on entropy or other principles, are likely to be developed for specific processes.
There is no doubt that new problems and issues will be tackled in the future. For example, inverse problems are now capable of being solved (e.g. given a velocity field, deduce the turbulence coefficients that produce such a flow field). The use of models in this way, both in calibration and application, will lead to new developments in fuzzy logic, uncertainty analysis and in understanding of hydraulic processes. As in hydrology, such questions will be pursued in hydraulics through a range of conceptual, deterministic and probabilistic models, all demanding greater knowledge of basic hydraulics and the underlying physics. Benchmarking exercises should increase, as should collaborative work on fundamental processes in jointly owned large-scale experimental facilities, professional networking and sharing of data. This must be good news for our profession as well as conference organizers.
Having eschewed making any firm predictions about future developments let me conclude by instead making just three suggestions:
(1) Share results – the acquisition of both knowledge and data are costly enterprises. Since rivers are universally present in all countries, and no two river basins are identical, we can learn so much more by sharing data and information, as well as playing a full part in IAHR conferences and technical meetings. The book on New Zealand rivers by Hicks and Mason Citation(1998) is a good example of how to share data easily, as are the media used by the FRMRC (www.floodrisk.org.uk and http://frmrc.hw.ac.uk), the UK Flood Channel Facility experimental results (Shiono and Knight Citation1991; www.flowdata.bham.ac.uk), the CES (www.river-conveyance.net and many more, as evidenced in a selection of websites given before the references. | |||||||||||||||||
(2) Shun popularity – at the risk of offending all my academic colleagues, avoid the pernicious influence of “impact factors” and try instead to:
| |||||||||||||||||
(3) Relax more – and you let your brain do it for you. Following the discovery of a new fundamental particle, consistent with the Higgs boson, Professor Peter Higgs from the University of Edinburgh is reported that he “hit on the concept of the Higgs mechanism in 1964 while walking in the Cairngorms” (The Times Newspaper, 7 July 2012). This just reinforces the second quotation by Kennedy given in Section 1. You do not necessarily need money to set the ball rolling in hydraulic engineering. Building large-scale experimental facilities can wait until later, like the Large Hadron Collider. |
I hope you have been stimulated by reading a different style of paper on river engineering, written from various viewpoints and with perhaps an unusual selection of questions and answers. Enjoy discovering and learning from your own “meandering pathway” through the science of one of the most delightful phenomena in the world – our rivers.
Websites
www.actif-ec.net/library/review_EU_flood_projects.pdf
References
- Abbot , M. B. and Basco , D. R. 1989 . Computational fluid dynamics: An introduction for engineers , New York : Longman Scientific and Technical, John Wiley .
- Abbot , M. B. , Havno , K. and Lindberg , S. 1991 . The fourth generation of numerical modeling in hydraulics . J. Hydraulic Res. , 29 ( 5 ) : 581 – 600 . (doi:10.1080/00221689109498978)
- Allen , J. 1947 . Scale models in hydraulic engineering , London : Longmans .
- Allen , J. 1970 . “ The life and work of Osborne Reynolds ” . In Osborne Reynolds and engineering science today , Edited by: McDowell , D. M. and Jackson , J. D. 1 – 82 . England : Manchester University Press .
- Anderson , M. G. , Walling , D. E.S. and Bates , P. D. 1996 . Floodplain processes , Chichester : John Wiley .
- Aronica , G. , Hankin , B. and Beven , K. 1998 . Uncertainty and equifinality in calibrating distributed roughness coefficients in a flood propagation model with limited data . Adv. Water Res. , 22 ( 4 ) : 349 – 365 . (doi:10.1016/S0309-1708(98)00017-7)
- ASCE Task Committee on Turbulence Models in Hydraulic Computation . 1988 . Turbulence modelling of surface water flow and transport, Parts 1-V . J. Hydraulic Eng. , 114 ( 9 ) : 970 – 1073 . (doi:10.1061/(ASCE)0733-9429(1988)114:9(970))
- Ashworth , P. J. , Bennett , S. J. , Best , J. L. and McLelland , S. J. 1996 . Coherent flow structures in open channels , Chichester : John Wiley .
- Batchelor , G. K. , Moffatt , H. K. and Worster , M. G. 2003 . Perspectives in fluid dynamics: A collective introduction to current research , Cambridge : Cambridge University Press .
- Berz , G. 2000 . Flood disasters: Lessons from the past – worries for the future. Proc. Inst. Civil Eng . Water and Maritime Engineering, London , 142 ( 1 ) : 3 – 8 . (doi:10.1680/wame.2000.142.1.3)
- Bettess , R. and White , W. R. 1987 . “ Extremal hypotheses applied to river regime ” . In Sediment transport in gravel bed rivers , Edited by: Thorne , C. R. , Bathurst , J. C. and Hey , R. D. 767 – 789 . Chichester : John Wiley and Sons .
- Beven , K. , Chatwin , P. and Millbank , J. 1994 . Mixing and transport in the environment , Chichester : Wiley .
- Bleninger , T. and Jirka , G. H. 2011 . Mixing zone regulation for effluent discharges into EU waters. Proc. Inst. Civil Eng . Water Management, London , 164 ( WM8 ) : 387 – 396 .
- Bronstert , A. 2006 . “ Overview of current perspectives on climate change, Chapter 3 ” . In River basin modelling for flood risk mitigation , Edited by: Knight , D. W. and Shamseldin , A. Y. 59 – 75 . Leiden : Taylor & Francis .
- Chang , H. H. 1988 . Fluvial processes in river engineering , New York : John Wiley .
- Cheng , N. S. and Nguyen , H. T. 2011 . Hydraulic radius for evaluating resistance induced by simulated emergent vegetation in open channel flows . J. Hydraulic Eng. , 137 ( 9 ) : 995 – 1004 . (doi:10.1061/(ASCE)HY.1943-7900.0000377)
- Chlebek , J. and Knight , D. W. 2006 . “ A new perspective on sidewall correction procedures, based on SKM modeling ” . In RiverFlow 2006 Lisbon, Portugal , Edited by: Ferreira , R. M.L. , Alves , E. C.T.L. , Leal , J. G.O.B. and Cardoso , A. H. Vol. 1 , 135 – 144 . Leiden : Taylor & Francis .
- Chow , V. T. 1959 . Open channel hydraulics , New York : McGraw-Hill .
- Colebrook , C. F. 1939 . Turbulent flow in pipes, with particular reference to the transition region between the smooth and rough pipe laws . J. Inst. Civil Eng. , 11 ( 4 ) : 133 – 156 .
- Colebrook , C. F. and White , C. M. 1937 . Experiments with fluid friction in roughened pipes . Proc. R. Soc. , A 161 : 367 – 381 .
- Coleman , S. E. Fluvial sediment transport and morphology: Views from upstream and midstream . RiverFlow 2010, Proc. Int. Conference on Fluvial Hydraulics , Edited by: Dittrich , A. , Koll , K. , Aberle , J. and Geisenhainer , P. pp. 11 – 21 . Karlsruhe : Braunschweig, Bundesanstalt fur Wasserbau (BAW) .
- Cunge , J. A. , Holly , F. M. and Verwey , A. 1980 . Practical aspects of computational river hydraulics , London : Pitman Publishing Ltd . (reprints available from Institute of Hydraulic Research, The University of Iowa).
- Davies , S. J. and White , C. M. 1929 . “ A review of flow in pipes and channels ” . In Engineering London reprints of July 19, 26 and August 2, 1929
- Einstein , H. A. and Li , H. 1958 . Secondary currents in straight channels . Trans. Amer. Geophys. Union. , 39 : 1085 – 1088 . (doi:10.1029/TR039i006p01085)
- Elder , R. A. 1986 . Advances in hydraulic engineering practice: The last four decades and beyond . J. Hydraulic Eng. , 112 ( 2 ) : 73 – 89 . (doi:10.1061/(ASCE)0733-9429(1986)112:2(73))
- Falconer , R. A. 1990 . Engineering problems and the application of mathematical models relating to combating water pollution . Municipal Eng. , 7 : 281 – 291 .
- Fukuoka , S. and Fujita , K. 1989 . Prediction of flow resistance in compound channels and its application to design of river courses . J. Hydr. Coast. Envir. Eng. , 411 ( 12 ) : 63 – 72 . (in Japanese).
- Grass , A. J. , Stuart , R. J. and Mansour-Tehrani , M. 1991 . Vortical structures and coherent motion in turbulent flow over smooth and rough boundaries . Trans. Phys. Sci. Eng. , 336 ( 1640 ) : 35 – 65 . (doi:10.1098/rsta.1991.0065)
- Hager , W. H. 2003, 2009 . Hydraulicians in Europe: 1800–2000 , Vol. 1 and 2 , Madrid : International Association of Hydraulic Engineering Research .
- Hartnett , T. , Dabrowski , T. and McGovern , J. 2011 . Modelling and monitoring towards “ecologically good” status. Proc. Inst. Civil Eng . Water Management , 164 ( WM8 ) : 421 – 431 .
- Hele-Shaw , H. S. 1899 . “ The motion of a perfect fluid, London Proc. R. Inst ” . In Great Britain Friday evening discourse , London : William Clowes and Sons, Charing Cross . 10 February
- Hele-Shaw , H. S. and Hay , H. 1900 . Lines of induction in a magnetic field . Phil. Trans. R. Soc. , 195 : 303 – 327 . (doi:10.1098/rsta.1900.0030)
- Hicks , D. M. and Mason , P. D. 1998 . Roughness characteristics of New Zealand rivers National Institute of Water and Atmospheric Research Ltd, 1–329, Water Resources Publications, Colorado.
- IAHR . 1993 . IAHR looking into sustainable development: Research agenda and future topics of concern , Edited by: Muller , A. Delft : IAHR .
- IAHR . 1994 . Guidelines for documenting the validity of computational modelling software , Delft : IAHR .
- IAHR . 1999 . Bridging the gap between research and application, IAHR Research Agenda 1999 , Delft : IAHR .
- Ikeda , S. 1981 . Self-formed straight channels in sandy beds . J. Hydraulic Div. , 107 ( 4 ) : 389 – 406 .
- Ikeda , S. and McEwan , I. K. 2009 . Flow and sediment transport in compound channels: The experiences of Japanese and UK Research , 1 – 333 . Madrid : Monograph Series, IAHR .
- ISO 1100-2 . 2010 . Measurement of liquid flow in open channels. Part 2: Determination of the stage-discharge relation , Geneva, Switzerland : International Standards Organization .
- JSME . 1988 . Visualized flow , Oxford : Japan Society of Mechanical Engineers, Pergamon Press .
- Kennedy , J. F. 1983 . Reflections on rivers, research and rouse . J. Hydraulic Eng. , 109 ( 10 ) : 1253 – 1271 . (doi:10.1061/(ASCE)0733-9429(1983)109:10(1253))
- Kennedy , J. F. 1987 . “ Hydraulic trends towards the year 2000 ” . In Hydraulics and hydraulic research: A historical review, IAHR 1935–1985 , Edited by: Garbrecht , G. 357 – 362 . Balkema, Rotterdam : Jubilee Volume, International Association for Hydraulic Research .
- Kennedy , J. F. 1989 . “ Whence and whither river sediment research? Fourth Int. Symp ” . In River Sedimentation 18 – 36 . Beijing
- Knight , D. W. 1996 . “ Issues and directions in river mechanics – Closure of sessions 2, 3 and 5 ” . In Issues and directions in hydraulics , Edited by: Nakato , T. and Ettema , R. 435 – 462 . Iowa, Balkema : An Iowa Hydraulics Colloquium in honour of Professor John F Kennedy, Iowa Institute of Hydraulic Research .
- Knight , D. W. 2008 . “ Modelling overbank flows in rivers – Data, concepts, models and calibration ” . In Numerical modelling of hydrodynamics for water resources , Edited by: Garcia-Navarro , P. and Playan , E. 3 – 23 . Leiden : Taylor & Francis .
- Knight , D. W. 2013 . “ Hydraulic problems in flooding: From data to theory and from theory to practice ” . In Experimental and computational solutions of hydraulic problems , Edited by: Rowinski , P. 1 – 34 . Lochow, Poland, Springer : International School of Hydraulics .
- Knight , D. W. and Samuels , P. G. 2007 . Examples of recent floods in Europe . J. Disast. Res. , 2 ( 3 ) : 190 – 199 .
- Knight , D. W. and Shamseldin , A. 2006 . River basin modelling for flood risk mitigation , Leiden : Taylor & Francis .
- Knight , D. W. and Shiono , K. 1996 . “ River channel and floodplain hydraulics ” . In Floodplain processes , Edited by: Anderson , M. G. , Walling , D. E.S. and Bates , P. D. 139 – 181 . Chichester : John Wiley .
- Knight , D. W. , Al-Hamid , A. A.I. and Yuen , K. W.H. 1992 . “ Boundary shear in differentially roughened trapezoidal channels ” . In Hydraulic and environmental modelling: Estuarine and river waters , Edited by: Falconer , R. A. , Shiono , K. and Matthew , R. G.S. 3 – 14 . Aldershot : Ashgate Press .
- Knight , D. W. , Yuen , K. W.H. and Alhamid , A. A.I. 1994 . “ Boundary shear stress distributions in open channel flow ” . In Physical mechanisms of mixing and transport in the environment , Edited by: Beven , K. , Chatwin , P. and Millbank , J. 51 – 87 . Chichester : John Wiley .
- Knight , D. W. , Cao , S. , Liao , H. , Samuels , P. G. , Wright , N. G. , Liu , X. and Tominaga , A. 2006 . Floods – Are we prepared? . J. Disast. Res. , 1 ( 2 ) : 325 – 333 .
- Knight , D. W. , Mc Gahey , C. , Lamb , R. and Samuels , P. G. 2010a . Practical channel hydraulics – Roughness, conveyance and afflux , Leiden : CRC Press/Taylor & Francis .
- Knight , D. W. , Tang , X. , Sterling , M. , Shiono , K. and Mc Gahey , C. 2010b . “ Solving open channel flow problems with a simple lateral distribution model ” . In Riverflow 2010, Proc. Int. Conf. on Fluvial Hydraulics Edited by: Dittrich , A. , Koll , K. , Aberle , J. and Geisenhainer , P. 41 – 48 . Braunschweig
- Ligget , J. A. and Caughey , D. A. 1998 . Fluid mechanics: An interactive text , Reston : ASCE Press, CD .
- Lighthill , M. J. 1966 . Waves in liquids and gases’, The seventh Selig Brodetsky memorial lecture , England : Leeds University Press .
- Lighthill , M. J. 1970 . “ Turbulence ” . In Osborne Reynolds and engineering science today , Edited by: McDowell , D. M. and Jackson , J. 83 – 146 . England : Manchester University Press .
- McDowell , D. M. and Jackson , J. D. Osborne Reynolds and engineering science today , pp. 1 – 263 . Papers presented at the Osborne Reynolds Centenary Symposium, The University of Manchester, September.
- Mc Gahey , C. , Samuels , P. G. , Knight , D. W. and O'Hare , M. T. 2008 . Estimating river flow capacity in practice . J. Flood Risk Manag. , 1 ( 1 ) : 23 – 33 . (doi:10.1111/j.1753-318X.2008.00004.x)
- Miller , J. B. 1997 . Floods: People at risk, strategies for prevention , New York : United Nations .
- Morvan , H. , Knight , D. W. , Wright , N. G. , Tang , X. and Crossley , M. 2008 . The concept of roughness in fluvial hydraulics and its formulation in 1-D, 2-D and 3-D numerical simulation models . J. Hydraul. Res. , 46 ( 2 ) : 191 – 208 . (doi:10.1080/00221686.2008.9521855)
- Nakato , T. and Ettema , R. 1996 . Issues and directions in hydraulics , Iowa, Balkema : An Iowa hydraulics Colloquium in honour of Professor John F Kennedy, Iowa Institute of Hydraulic Research .
- Nezu , I. and Nakagawa , H. 1993 . Turbulence in open-channel flows , Balkema, Rotterdam : IAHR monograph .
- Nikora , V. 2008 . “ Hydrodynamics of rough-bed turbulent flows. Proc. Int. Conf ” . In Fluvial River Hydraulic Flow 2008 , Edited by: Altinakar , M. S. , Kokpinar , M. A. , Aydin , I. , Cokgor , S. and Kirkgoz , S. 11 – 19 . London : Cesme, Izmir, Taylor & Francis .
- Pendennis-Wallis , R. and White , C. M. 1939 . “ Resistance to flow through nests of tubes ” . In Engineering reprints of November 26, Dec. 9 and 23, 1938, London.
- Perkins , H. J. 1970 . The formation of streamwise vorticity in turbulent flow . J. Fluid Mech. , 44 ( Part 4 ) : 721 – 740 . (doi:10.1017/S0022112070002112)
- van Prooijen , B. C. , Battjes , J. A. and Uijttewaal , W. S.J. 2005 . Momentum exchange in straight uniform compound channel flow . J. Hydraul. Eng. , 131 ( 3 ) : 175 – 183 . (doi:10.1061/(ASCE)0733-9429(2005)131:3(175))
- Ramsbottom , D. M. and Whitlow , C. D. 2004 . Extension of rating curves at gauging stations: Best practice guidance manual, R and D Manual W6-061/M , Bristol : The Environment Agency .
- Rodi , W. 1980 . Turbulence models and their application in hydraulics: State of the art paper , Delft : IAHR .
- Rouse , H. 1976 . Hydraulics in the United States: , 1776 – 1976 . Iowa : Institute of Hydraulic Research, The University of Iowa .
- Rouse , H. 1978 . First four decades of the hydraulics division . J. Hydraulic Div. , 104 ( HY3 ) : 327 – 336 .
- Rouse , H. 1980 . Some paradoxes in the history of hydraulics . J. Hydraulic Div. , 106 ( HY6 ) : 1077 – 1084 .
- Rouse , H. and Ince , S. 1957 . History of hydraulics , New York : Dover Publications Inc .
- Sharifi , S. , Knight , D. W. and Sterling , M. 2009 . A novel application of a multi-objective evolutionary algorithm in open channel flow modelling . J. Hydroinformatics. , 11 ( 1 ) : 31 – 50 . (doi:10.2166/hydro.2009.033)
- Sharifi , S. , Sterling , M. and Knight , D. W. 2010 . Can the application of a multi-objective evolutionary algorithm improve conveyance estimation? . Water Environ. J. , 25 : 230 – 240 . (doi:10.1111/j.1747-6593.2010.00223.x)
- Shiono , K. and Knight , D. W. 1991 . Turbulent open channel flows with variable depth across the channel . J. Fluid Mech. , 222 : 617 – 646 . (and Vol. 231, p. 693). (doi:10.1017/S0022112091001246)
- Shu , C. , Xia , J. , Falconer , R. A. and Lin , B. 2011 . Incipient velocity for partially submerged vehicles in floodwaters . J. Hydraulic Res. , 49 ( 6 ) : 709 – 717 . (doi:10.1080/00221686.2011.616318)
- Tehrani , M. M. 1992 . Spatial distribution and scaling of bursting events in boundary layer turbulence over smooth and rough surfaces , London : Department of Civil and Environmental Engineering, University College . PhD Thesis.
- Tominaga , A. and Nezu , I. Turbulent structure of shear flow with spanwise roughness heterogeneity. Proc. Int. Symp. Environmental Hydraulics . December 16–18 , Hong Kong . pp. 415 – 420 .
- Viollet , P. L. 2007 . Water engineering in ancient civilizations: 5000 years of history , Madrid : IAHR .
- White , W. R. , Bettess , R. and Paris , E. 1982 . Analytical approach to river regime . J. Hydraulics Div. , 108 ( HY10 ) : 1179 – 1193 .
- Xia , J , Falconer , R. A. , Lin , B. and Tan , G . 2011 . Modelling flash flood risk in urban areas. Proc. Inst. Civil Eng . Water Management, London , 164 ( WM6 ) : 267 – 282 .
- Yen , B. C. 1991 . Channel flow resistance: Centennial of Manning's formula , Colorado : Water Resources Publications .
- Zalewski , M. 2011 . Ecohydrology for implementation of the EU framework directive. Proc. Inst. Civil Eng . Water Management, London , 164 ( WM8 ) : 375 – 385 .