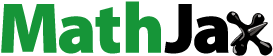
Abstract
In dance, music, or sports, reproducibility and consistency as well as bilateral dexterity/coordination of movement are crucial for motor control. Research into the biomechanics of movement consistency and variability is important for motor learning to achieve proficiency and maximize outcome reproduction and stability as well as to reduce the risk of injury. Thirty-six participants were instructed to perform a repetitive circular, ipsilateral motion of arms and legs at three different tempi, while being recorded with optical motion capture. Two velocity-based consistency measures were developed an overall measure of consistency and a laterality difference measure. Maintaining velocity consistency was more challenging at slower than at faster tempi, suggesting that slow movement could require more attentional focus and thus become more variable. Music experience resulted in higher consistency, especially on the subdominant body side, possibly due to extensive bilateral training. Outcomes could have potential implications for music instrument, dance, and sports practice and training.
Subject classification codes:
Introduction
In dance, music, or sports, the ability to consistently replicate a movement or a movement sequence is an important skill to achieve proficiency (Konczak et al., Citation2009; Preatoni et al., Citation2013; Simmons, Citation2005). Consistent replication enables desirable and reliable outcomes, be it dance choreography, performing a piece of music, or executing efficient swimming or rowing strokes. Training and practice aim to constantly improve individual capabilities to perform better and achieve more consistent results (cf. Bernstein, Citation1967). Yet, regardless of proficiency or familiarity with performing a movement, a certain amount of variability is always present in a repeated movement, as even top professionals cannot execute identical movement sequences (Davids et al., Citation2006; Preatoni et al., Citation2013). Partly this variability has been claimed to be noise of the motor system, partly it has been argued that it is functional, as it offers adaptability of the movement patterns that is needed to ensure a stable performance (e.g., Newell et al., Citation2006). However, despite this inherent variability, overall, reducing and controlling it as much as possible during motor learning seems favorable not only to achieve proficiency and to maximize outcome consistency, reproduction, and stability (Gentile, Citation2000), but also as a way to reduce the risk of injuries (Dierks & Davis, Citation2007; Newell & Ranganathan, Citation2009). Thus, research into the biomechanics of movement proficiency regarding consistency and variability is important for monitoring the professional’s skill level and to furthermore prevent injuries due to the high demand of their tasks and movements on the body (Dierks & Davis, Citation2007; Preatoni et al., Citation2013).
Theories and experimental findings of motor learning point to consistency as a key facet of expert performance. In Gentile’s (Citation2000) two stage model of motor skill acquisition, initial learning is typically based on trial and error, and movements are not very efficient and reproducible. Later stages of learning, in contrast, show higher economy of effort, higher adaptability to the demands of the specific task, and higher consistency. One way to estimate movement consistency is to calculate the statistical variation across repeat trials. Baudry et al. (Citation2008) asked expert and non-expert gymnasts to perform sequences on a pommel horse repeatedly. Spatiotemporal consistency was investigated by calculating the standard deviations of body marker trajectories, resulting in higher consistency for experts in the ankle movements. In the performing arts, dancers or musicians practice many hours in order to achieve a proficient and reliable performance. Being able to reproduce choreography or a piece of music reliably may thus demonstrate the level of the performer’s expertise. In terms of timing and expressiveness, skilled pianists draw on internal representations that enable them to play a piece of music repeatedly with similar timing profiles, even if auditory feedback is not available (Repp, Citation1999). Dancers are not only more balanced and more consistent when drawing ellipses with their foot than non-dancers (Thullier & Moufti, Citation2004), but their muscle activation patterns are also less variable across repeated trials including postural balance perturbations (Simmons, Citation2005). In violin performance, which requires particularly high levels of upper body control, it was found that sagittal shoulder motion, which is closely related to controlling the violin bow, was less variable in experts compared to novice or amateur violinists (Konczak et al., Citation2009). In a similar vein, Schoonderwaldt and Altenmüller (Citation2014) measured performance stability by calculating the standard deviations of the bowing phase, the range as well as the bow inclination offset. Amateur violinists were more variable, hence less consistent, compared to student and professional violinists. Comparable results were found for piano playing, such that experts as compared to novices were more consistent across key strikes in terms of velocity and striking force (Furuya & Kinoshita, Citation2008). Wöllner and Cañal-Bruland (Citation2010) asked groups of violinists, musicians of other instruments and non-musicians to synchronize with the entry gestures of an unfamiliar first violinist by tapping on a keyboard once for each entry. The action expertise of the violinists group led to smaller inter-trial timing variability compared to the other two groups of participants. Taken together, there is strong evidence that expert across different domains show high levels of consistency in their domain-specific actions, which is reflected in their muscle activity, movements, and action-perception systems.
Movement consistency seems to bear an inherent relationship to performance tempo, as skill acquisition in sports and artistic performance requires mastery of the temporal structure to perform expertly. In sports, when being asked to focus on accuracy, people usually reduce their execution speed (Schmidt et al., Citation2019), which was found, for instance, in handballers (van den Tillaar & Ettema, Citation2006). While it is seen as a common way to practice a new piece on an instrument by slowing down the tempo in the beginning (e.g., Barry & McArthur, Citation1994), it was also found in music studies that performance was more accurate when it was practiced at a tempo close to the final performance tempo (Pierce, Citation2006). Moreover, Palmer and van de Sande (Citation1995) observed no speed/accuracy tradeoff in expert adult pianists’ performances, in the sense that errors did not increase at faster performance tempi. Related to sports, Nikodelis et al. (Citation2005) found that anti-phase inter-limb coupling in freestyle swimming was stronger at faster compared to slower tempo, while no effect of expertise (novices versus experts) was revealed.
Adams suggested closed and open loop systems for human movement control (Adams, Citation1971; Schmidt et al., Citation2019). Closed loop control refers to a feedback-based mechanism, where movement control (e.g., error correction, change, or adaptation) happens through sensory-motor feedback. Since sensory processing is used for movement control, movement execution cannot be faster than sensory feedback, resulting in faster movement becoming potentially less accurate. Open loop control, on the other hand, is a feed forward control mechanism that does not include feedback for error correction, and is for instance used when there is the need to quickly integrate sensory information. This could be also seen in light of Schmidt’s schema theory of motor control (Schmidt, Citation1975; Ehrlenspiel, Citation2001) that divides cognitive and motor processes into ‘automatic’, meaning fast, effortless processes not under subjective control, but used for executing well-developed skills, and ‘controlled’, meaning slow, serial-type processes being subject-regulated and used for new tasks or information.
Temporal aspects in music have been studied extensively in the field of sensorimotor synchronization using finger tapping paradigms (see Repp, Citation2005; Repp & Su, Citation2013). Humans can synchronize to a metronome pulse in a wide range of tempi from about 100-200 to 1800 msec (Fraisse, Citation1982; Repp, Citation2003). Zendel et al. (Citation2011) used a tempo/inter onset interval (IOI) range from 260 to 1560 msec and found decreasing tapping asynchronies and variability the shorter the IOI (i.e., the faster the tempo), suggesting more accurate and constant synchronization with faster compared to slower tempi. Most accurate synchronization has been found for tempi between 300 and 900 msec (Fraisse, Citation1982; van Noorden & Moelants, Citation1999), with a preference for beats in the 500–600 msec range (Fraisse, Citation1982; Moelants, Citation2002). Moreover, musically highly trained participants showed generally higher accuracy in tapping consistency (i.e., less asynchronies) than musically non-trained (Krause et al., Citation2010; Repp & Doggett, Citation2007; Repp & Penel, Citation2004), whereas Hove et al. (Citation2010) found no difference between amateur musicians and non-musicians. Not only finger tapping has been used in synchronization tasks: Studenka and Zelaznik (Citation2011) used circle drawing instead, finding that phase drift occurred (i.e., participants were too fast), while Repp and Steinmann (Citation2010) suggested phase stability, but asynchronies being twice as large than in finger tapping. Furthermore, Repp (Citation2011) found that circle drawing asynchronies and variability increased with slower tempo, in line with the results achieved in finger tapping studies.
Related to movement consistency, lateral dexterity and the ability to move both sides of the body in equivalent ways – to be able to for instance move both arms efficiently in rowing or swimming, perform the same motion with both arms in a contemporary or ballet dance sequence, or play a musical instrument evenly – is crucially important. In case of musical instruments though it has to be noted that different instruments afford different skills for each body side (e.g., string instruments), with both sides being highly trained and versatile for their specific set of playing requirements. However, despite the symmetry of the human body, the two body sides (i.e., arms, hands, legs, and feet) are very different in their sensorimotor abilities. Most people have a preference for side, called handedness or footedness – this is for 90% of the world population for instance the right hand, leading to asymmetries in dexterity (Goble & Brown, Citation2008). Building up bilateral symmetry and dexterity, however, is not only beneficial for successful performance and a sign of proficiency, but also for reducing injuries and pains resulting from both repetitive and one-sided use of the body (Parrington & Ball, Citation2016).
A general finding in the field of music research has been that musicians show less asymmetry compared to non-musicians, probably due to the bimanual use and requirements when playing musical instruments (Kopiez et al., Citation2010). In a study by Jäncke et al. (Citation1997), right-handed musicians showed higher hand skill symmetry in different dexterity tasks than non-musicians. Fuji and Oda (Citation2006) found that drummers, compared to non-drummers, tapped significantly more stable for with both dominant and non-dominant hands. In addition, they were faster with their non-dominant hand, while both groups tapped similarly fast with their dominant hand. However, results of a study by Kopiez et al. (Citation2012) suggested that for both right- and left-handed pianists, precision of the right hand was better than for the left hand, reflecting the fact that the main melodic and most virtuosic lines in western music are typically played by the right hand. Moreover, several brain studies have suggested stronger equilaterality in musicians than non-musicians. Schlaug et al. (Citation1995) discovered that musicians have larger corpus callosum sizes than non-musicians suggesting more interhemispheric communication and hemispheric symmetry. Burunat et al. (Citation2015) results furthermore indicated increased interhemispheric functional symmetry particularly in visual and motor networks in musicians compared to non-musicians, as well as in keyboarders compared to string players. This increased bilateral neural connectivity could be helpful when performing bimanual movement tasks.
Movement laterality in dance has been researched far less. Kimmele (Citation2010) points out that even a trained dancer can have an asymmetrical body structure and a functionally asymmetrical dance technique as well as a lateral preference, which is, however, not favorable, both in terms of performance quality and prevention of injury. Ramsay and Riddoch (Citation2001) and Jola et al. (Citation2011) demonstrated that, in an upper limp proprioceptive positioning task and respectively in a proprioceptive endpoint position matching task, professional ballet dancers achieved greater accuracy than non-ballet dancers for both the dominant and the sub-dominant arm. While these results suggest that artistic expertise could result in more consistent bilateral coordination, not much is known yet about how laterality and expertise relate to movement consistency.
Therefore, the present study aimed to investigate movement consistency in the context of tempo, laterality, and music and dance expertise from an artistic perspective. To this end, a motion capture experiment was designed using a circular, repetitive dance-like movement routine involving coordination of arms and legs at different tempi. The movement was performed in three different tempi (a comfortable tempo based on pilot testing, and a slower and faster tempo than that) to account for possible biomechanical constrains and potential learning benefits at different tempi. From the motion capture data, a consistency measure was computationally derived based on the velocity of the performed motion, providing insights about individual motor and body control during a circular repeating motion. The standard deviation of the velocity of a repeated, circular motion (see Movement Data Processing/Consistency Measures below) was used as the consistency measure, being aware of an ongoing debate about which measure to choose (e.g., Parrington & Ball, Citation2016). However, we chose the standard deviation, being a relatively simple and straightforward measure, because of its previous use in related studies (e.g., Baudry et al., Citation2008; Schoonderwaldt & Altenmüller, Citation2014), and since normally (i.e., regularly, circle-shaped within a restricted diameter) distributed data could be assumed due to the circular nature of the movement and the relative simplicity of the data.
Regarding the relationships between movement consistency and tempo, laterality and experience, the following hypotheses were proposed based on the literature summarized above:
H1) We expected that dance sequences at fast and medium tempi would be more consistent compared to slow tempo. More specifically, the medium tempo would lead to most consistent movement (in line with sensorimotor synchronization results), while slow tempo would lead to most variable movement.
H2) Velocity consistency was predicted to increase with participants’ music and dance experience, as prior experience helps maintaining stable motor control.
H3) Taken the dominant side bias, velocity consistency was assumed to be better for the dominant hand in general. However, it was expected that increasing music and dance experience shifts this to a more balanced consistency between both body sides, due to being trained in bimanual coordination.
Materials and Methods
Participants
Thirty-six participants (female = 23, mean age = 27.39 years, SD = 7.40) were recruited via word of mouth and email lists to take part in the experiment. One further participant was excluded due to being unable to perform the movements at the required level of competence. All participants had the physical ability required to move as instructed and had normal or corrected-to-normal vision. Participants were of normal body shape (mean BMI: 22.1, SD BMI: 2.6). All but four participants were right-handed according to the Edinburgh Handedness Inventory (short form, see Oldfield, Citation1971, and Veale, Citation2014).
Participants’ years of music and dance training was assessed and divided into three equal-sized groups for each domain (music and dance). For musical experience, the three groups were as follows: Training level 1: 0 to 7 years; Training level 2: 8 to 14 years; Training level 3: more than 14 years; while for dance experience, they were: Training level 1: 0 years; Training level 2: 1 to 4 years; Training level 3: more than 4 years. Due to an open recruitment strategy, we had flowing boundaries for the experience categories instead of forming three distinct groups with more clearly bordered ranges. The two experience variables did not correlate with each other (r(36) = −.04, p = .81), thus we assume an independent distribution within the sample. For music experience, the groups differed slightly in age, as the participants in Training level 2 were significantly younger than in Training level 1 (F(2, 33) = 3.49, p = .042; M(TL1) = 30.50 years, SD(TL1) = 10.35; M(TL2) = 23.25, SD(TL2) = 3.31; M(TL3) = 28.42, SD(TL3) = 5.05; difference between TL1 and TL 2, p = .045). There were no significant differences for the dance experience groups (F(2, 33) = 0.66, p = .52). Furthermore, groups did not differ significantly in terms of their BMI (music: F(2, 33) = 1.48, p = .24; dance: F(2, 33) = 0.59, p = .56). Participants were either awarded course credits or received 10€for taking part. Participants took part in accordance with the guidelines of the Ethics committee of the Facility of Humanities, University of Hamburg.
Design and Procedure
Participants were taught to perform a repetitive dance-like movement while being recorded with optical motion capture. Each participant was trained separately by an instructor with a background in professional dance and performed the task individually. The movement was both verbally described and physically demonstrated by the instructor, before being performed by the participants. To avoid confounding effects of familiarity with movement (Calvo-Merino et al., Citation2006), we chose relatively novel movements based on ipsilateral coordination between arms and legs. The movements were adapted from Tanaka Min’s “Body Weather” dance training methodology (Marshall, Citation2006). The type of movement chosen was relatively slow and continuous to be suitable for consistency: participants adopted a martial arts type stance with feet wide and turned out, and knees slightly bent. They then stepped from one leg to the other moving their knees in circles while simultaneously circling their ipsilateral arm (see ). Participants were instructed to keep their torso as still as possible, to perform the movement in the most consistent and stable way and in the tempo as indicated by the auditory stimulus.
Figure 1. (A) Movement task as trace illustration (one cycle with the right side followed by one cycle with the left side); (B) Example of position data in three dimensions of the right hand (five cycles). (C) Example of velocity data in three dimensions of the right hand (five cycles).
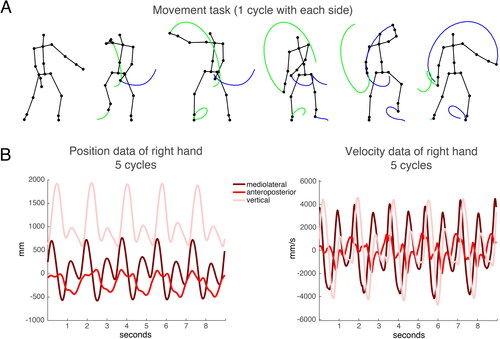
Participants were recorded performing this movement at three different speeds (slow, medium, and fast) for 30 seconds each. The medium speed was intended to provide a ‘comfortable’ speed, while the slow and fast speeds were intended to challenge participants. The speeds corresponded for the slow tempo to 30 BPM (beats per minute, i.e., one cycle equals 2 seconds, 15 cycles in total), for the medium tempo to 45 BPM (22 cycles in total), and for the fast tempo to 67 BPM (33 cycles in total). The speeds had been tested and adjusted in a pilot phase with five untrained and inexperienced participants. During the actual recording session, each condition was recorded a minimum of two and maximum of five times per participant and speed level, until adequate performance was achieved in terms of postural control and matching the tempo. The instructor afterwards chose the best performances based on these criteria.
The speeds of the movements were controlled through sound stimuli created using loops in Ableton Live 10. It consisted of a looped sinusoidal sweep sound with oscillating pitch from low (60 Hz) to high (260 Hz) back to low (illustrating the circular characteristic of the movement) of corresponding length for each speed level with a tom-tom sound to mark when the foot should approximately hit the floor (see for a spectrogram of the auditory stimulus). The sound was used as a tempo-indicator, while the participants were not explicitly told to synchronize to the signal. Additionally, the start and end of the motion capture recordings were indicated with audible beeps.
Figure 2. Spectrogram of the auditory stimulus (45 BPM, medium tempo). The black wave indicates the sinusoidal sweep sound, the gray vertical lines the tom-tom sound.
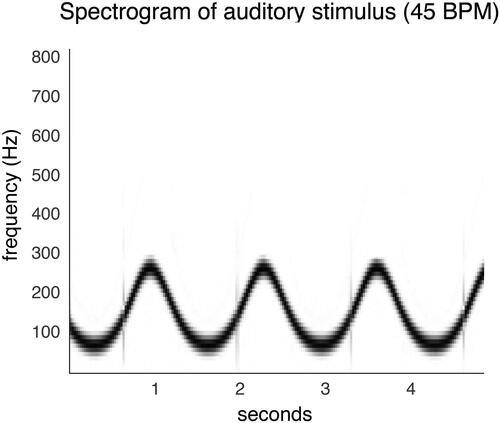
The performed order of the speeds was the same for all participants (medium, slow, fast). This approach was chosen to achieve the same priming across participants and thus yield the most consistent and similar recordings. For example, participants may have achieved less stability in slow conditions if recorded after fast conditions due to having muscles primed for faster changes. Participants also filled out background questionnaires, including basic demographic information, Edinburgh Handedness Inventory (short), as well as number of years of dance and music training. Thus, the experimental design yielded two within-participants variables: speed (3 levels) and laterality (2 levels); and one in-between variable: music resp. dance experience (3 levels).
Apparatus
Recordings took place in an approximately five by five meter laboratory fitted with a semi-sprung dance floor. Participants’ movements were recorded using an 11-camera optical motion capture system (Qualisys Oqus), tracking the three-dimensional positions of 31 reflective markers attached to each participant at a frame rate of 200 Hz. The positions of the markers can be seen in .
Figure 3. Marker and joint locations. (A) Anterior view of the marker locations as stick figure illustration; (B) Anterior view of the locations of joints used in the analysis.
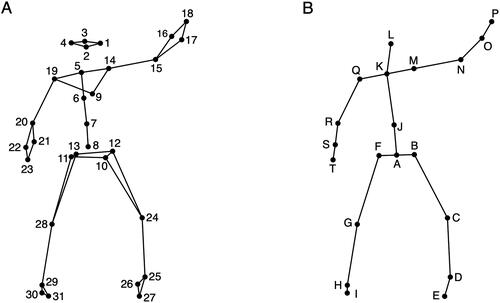
The location of the markers was as follows (L = left, R = right, F = front, B = back): 1: LF head; 2: RF head; 3: LB head; 4: RB head; 5: spine C7; 6: spine T7; 7: spine L3; 8: spine S2; 9: sternum; 10: LF hip; 11: RF hip; 12: LB hip; 13: RB hip; 14: L shoulder; 15: L elbow; 16: L wrist/radius; 17: L wrist/ulna; 18: L middle finger; 19: R shoulder; 20: R elbow; 21: R wrist/radius; 22: R wrist/ulna; 23: R middle finger; 24: L knee; 25: L ankle; 26: L heel; 27: L big toe; 28: R knee; 29: R ankle; 30: R heel; 31: R big toe. Sound cues for controlling the speed of the movement conditions were played using Audiodesk software through a mounted four-channel Neumann speaker system. Motion capture recordings were synchronized with the audio cues using SMPTE time code controlled by a MOTU audio interface and Audiodesk software. Both example point light displays and sound stimuli are available on the project’s Zenodo repository (LINK TO FOLLOW).
Movement Data Processing/Consistency Measures
After labeling the motion capture data, the MATLAB Motion Capture (MoCap) Toolbox (Burger & Toiviainen, Citation2013) was used to first derive a set of 20 secondary markers – subsequently referred to as joints – from the original 31 markers. The locations of these 20 joints are depicted in . The locations of joints C, D, E, G, H, I, M, N, P, Q, R, and T are identical to the locations of one of the original markers, while the locations of the remaining joints were obtained by averaging the locations of two or more markers; joint A: midpoint of the four hip markers 10-12; B: midpoint of markers 10 & 12 (left hip); F: midpoint of markers 11 & 13 (right hip); J: midpoint of spine, sternum, and hip markers 5-13 (mid-torso); K: midpoint of shoulder markers 14 & 19 (manubrium), L: midpoint of the four head markers 1-4 (head); O: midpoint of the two left wrist markers 16 & 17 (left wrist); S: mid-point of the two right wrist markers 21 & 22 (right wrist).
To broadly align the data (i.e., participants facing the same frontal direction), they were rotated so that the hip joints (A-C) were parallel to the mediolateral axis on average per person and trial. Subsequently, the data was trimmed to contain ten dance-like movement cycles in total (five circles per side) taken from the middle of each recording (slow tempo: cycles 3-12; medium tempo: cycles 5-14; fast tempo: cycles 11-20). The middle section was used to account for the time participants might have needed to get into the movement and produce a stable performance in the beginning and for a certain fatigue effect in the end of each trial. We thus expected the middle section to be the most stable and consistent. We also aimed to analyze the same number of circles, not the same time of performance across the three tempo conditions, to avoid incorrect/inflated values due to more repetitions at the fast tempo. Next, the data were rotated separately for each recording so that the circles of each arm formed the largest diameter on average over the five repetitions, ensuring that the calculation of the consistency was based on the best orientation of the participants’ movements in a 2-dimensional space relative to the frontal plane. Following this, joints of the left (B, C, D, E, M, N, O, & P) and right (F, G, H, I, Q, R, S, & T) side were separated to assess body laterality. For each joint and tempo, the instantaneous velocity was estimated using numerical differentiation and applying a second-order low pass Butterworth smoothing filter with cutoff frequency of 12 Hz, and data were trimmed to only contain the circle phase (discarding the resting phase, when the other arm was performing the circle). The data were standardized (z-scores) to account for a possible influence of heights and arm lengths of different participants. The data processing workflow is graphically displayed in .
Figure 4. Data processing workflow. (A) Flowchart of the data processing steps; (B) – (D) graphical depiction of the data processing steps, the upper row showing data from a highly consistent participant, the lower row data from a highly inconsistent participant: (B) velocity time series of the right hand in mediolateral (x) and vertical (z) dimensions; (C) velocity with the mediolateral dimension plotted against the vertical dimension displaying the circles phases (solid lines) and when the other hand is performing the circle (dashed lines); (D) data after removing the dashed parts to have the data only contain the cycle phases (data that was finally analyzed).
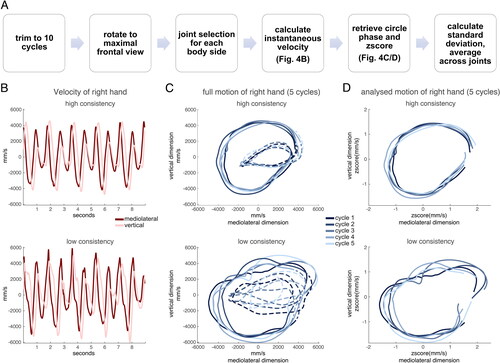
Subsequently, a consistency measure was derived by calculating the distance of each point in the frontal plane over the five cycles for each joint and tempo, taking the standard deviation as a measure of (velocity) consistency/variability, and averaging the standard deviations across the joints of left and right side respectively, receiving six values per participant (left and right side at three tempi). A higher value corresponds to a higher averaged standard deviation, thus less consistent velocity over the repetitions, while a lower value corresponds to more consistent velocity over the repetitions. In order to account for right- or left-hand dominance, the data of the four left-handed participants were flipped to receive values for the dominant and subdominant side respectively.
A second measure, assessing the relative laterality difference in velocity consistency between both body sides when executing the task, was taken by calculating the signed difference between the laterality values per tempo level and participant. This measure gives an indication of whether a participant was able to move both sides of the body similarly consistent. As the values for the dominant side were subtracted from the subdominant side, difference scores close to zero would indicate that participants moved in similar ways with both dominant and subdominant sides, while positive values would mean that the dominant side was more consistent than the subdominant side, and negative values would indicate that the subdominant side was more consistent compared to the dominant side. However, this constitutes a relative measure between both body sides independent from the actual level of the individual’s overall consistency.
Results
In order to assess effects of tempo, laterality, and music respectively dance experience on velocity consistency in a repetitive dance-like movement task, two mixed ANOVAs with tempo and laterality as within-subjects variables and each experience as between-subjects variable were conducted. Two additional mixed ANOVAs were conducted with the difference scores assessing effects of laterality differences at the tempo levels and music respectively dance experience.
The mixed ANOVA for music experience on velocity consistency resulted in a significant main effect for tempo, as well as in a significant main effect for experience, and a significant interaction effect between laterality and experience (see for statistical results). The main effect for laterality as well as the remaining interactions was non-significant (see ).
Table 1. Overview of the mixed ANOVA results. Significant effects are indicated in bold.
Following up the main effect for tempo, post-hoc pair-wise comparisons using Bonferroni correction revealed significant differences between fast (M = 0.23, SD = 0.07) and slow (M = 0.29, SD = 0.08) tempo performances (t(35) = −3.57, p = .002), with higher consistency in the fast tempo; as well as between slow and mid tempi (M = 0.24, SD = 0.08), with higher consistency for mid tempo (t(35) = −2.57, p = .038). The results are graphically displayed in . Following up the significant interaction between laterality and experience, pair-wise comparisons using Bonferroni correction indicated significant differences between Training level 1 (M = 0.30, SD = 0.06) and Training level 2 subdominant side (M = 0.24, SD = 0.03), t(22) = 3.17, p = .04, as well as Training level 1 and Training level 3 subdominant side (M = 0.22, SD = 0.04), t(22) = 4.02, p = .004, with no significant differences between Training level 2 and Training level 3 subdominant side as well as none on the dominant side, suggesting that music novices showed more variability in their subdominant side compared to participants with more musical training. As visible in the graphical depiction in , participants in Training levels 2 and 3 tended to be both more consistent compared to Training level 1 for the dominant side as well (though non-significantly so, p = .072, for the difference between Training level 1 and 2).
Figure 5. Velocity consistency mixed ANOVA for music experience. (A) Main effect of tempo. (B) Interaction effect between laterality and music experience. Note: The higher the value is, the more inconsistent the movement was. Error bars indicate the standard error. (** p < .01, * p < .05).
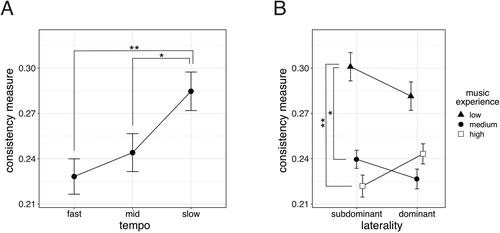
The mixed ANOVA for dance experience on velocity consistency resulted, as above, in a significant main effect for tempo (see for statistical results), with significant differences between fast and slow tempo, t(35) = −3.73, p = .001, mid and slow tempo, t(35) = −2.67, p = .027 (so following the same pattern as in the ANOVA on music experience displayed in ). Dance experience, however, failed to have significant main or interaction effects with the other variables (see ; the interaction between laterality and dance experience was close to reaching significance, however, post-hoc comparisons revealed no statistical differences between the variables), suggesting that dance experience did not influence velocity consistency in the repetitive dance-like movement task used in the current study.
The mixed ANOVA for music experience on the laterality difference scores yielded a main effect for music experience (see for full statistical results). Following up this effect, pair-wise comparisons using Bonferroni correction revealed a significant difference between Training level 1 (M = 0.01, SD = 0.03) and Training level 3 (M = −0.02, SD = 0.03), t(22) = 2.76, p = .028, and a marginally significant difference between Training level 2 (M = 0.01, SD = 0.02) and Training level 3, t(22) = 2.49, p = .054, suggesting that participants with less musical training were significantly more consistent in their dominant-side velocity (positive values), while highly musically trained participants were relatively more consistent in their subdominant-side velocity (negative values). The result is graphically displayed in .
Figure 6. Laterality difference score mixed ANOVA for (A) music and (B) dance experience main effects. Positive values indicate more consistent values on the dominant side, whereas negative values indicate more consistent values on the subdominant side. Error bars indicate the standard error. (* p < .01, * p ∼ .05).
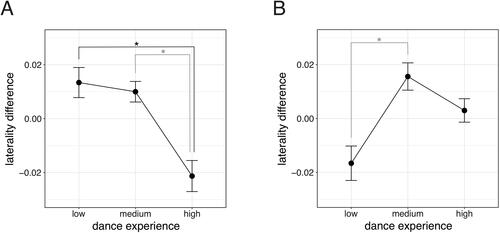
The mixed ANOVA for dance experience on the laterality difference scores resulted in a marginally significant main effect of dance experience (see for full statistical results). Pairwise comparisons using Bonferroni correction indicated a marginally significant difference between Training level 1 (M = −0.02, SD = 0.04) and Training level 2 (M = 0.02, SD = 0.03), t(22) = −2.46, p = .058, suggesting that participants with no previous dance experience were more consistent on their subdominant side, while participants with some dance experience were more consistent on their dominant side (see ). Noteworthy, as indicated in the graphical depiction in , Training level 3 participants (M = 0.00, SD = 0.03) were similarly consistent on both sides.
Discussion
In this study, we investigated the interplay between tempo, body laterality, and movement experience on velocity consistency in a controlled dance-like movement routine. We developed two consistency measures – a velocity consistency measure and a laterality difference measure – based on the velocity profiles of a repetitive circular, ipsilateral motion of arms and legs performed at three different tempi. We found that velocity consistency was mainly affected by the performance tempo as well as by music experience, which had a particular impact on the consistency of the subdominant side. Dance experience failed to show a relation to the velocity consistency, but did show a relation to the laterality difference measure. Overall, these results provide new insights into movement consistency, suggesting a strong influence of music experience on the ability to maintain a consistent velocity during a repetitive circular task.
Velocity consistency resulted in a significant main effect for tempo with significant differences between the slow and fast as well as slow and mid tempi. Performance at the slow tempo was less consistent than for mid and fast tempo performances, suggesting it being more difficult and potentially motorically more challenging to maintain a consistent and constant movement speed in slow tempo range. This outcome confirms findings from sports (e.g., Nikodelis et al., Citation2005) and especially from sensorimotor synchronization, in that finger tapping and drawing circles become more variable the slower the tempo that could be related to more discrete movements to ensure smooth and regular coordination (e.g., Huys et al., Citation2008; Repp, Citation2011; Varlet et al., Citation2012; Zendel et al., Citation2011). Furthermore, this result is in line with our first hypothesis, although a possibly boosting effect of the medium tempo on consistency was weaker than expected, since there was no difference between mid and high tempi. Being able to keep a more consistent speed at higher tempi could be related to biomechanical constrains of the human body as well as to motion perpetuation and momentum of the circular movement. This could comply with Schmidt’s schema theory of motor control (Schmidt, Citation1975), suggesting that such fast movement could constitute an ‘automatic’ process and thus be more consistently executed, while slower movements could require more attentional focus and motor control, and thus become more variable. It could further be that slow movement rely more on sensory-motor feedback being used to control the movement (i.e., greater ‘closed loop control’, Adams, Citation1971; Schmidt et al., Citation2019), while movements at faster rates would more likely be open loop with less sensory information being used. Moreover, moving at a slower tempo than the comfortable, medium tempo used in our study (which would be expected to be close to the preferred tempo if participants were asked to perform the movement at a self-chosen tempo) could yield more inconsistent movement, due to performing at a slower tempo than the own ‘eigenfrequency’ (in line with previous findings in the domain of visual coordination, see e.g., Lopresti-Goodman et al., Citation2008, audio sensorimotor coordination, e.g., Huys et al., Citation2008, as well as audio and visual sensorimotor coordination, e.g., Varlet et al., Citation2012). Future studies could include movement routines of various levels of complexity. From a (music/dance/sports) educational standpoint, practicing movement sequences in a slow tempo could enhance movement consistency and motor control overall.
Furthermore, a significant interaction was found between body laterality and music experience for the velocity consistency measure, showing significant differences between the low-level trained and both the medium- and high-level trained participants for the subdominant side. Participants with considerable musical training performed the movement with more consistent velocity of their subdominant side than participants with little musical training, which supports our third hypothesis (musicians being more bilateral), as well as partly our second hypothesis (overall results indicate higher consistency for musically trained compared to non-trained participants). This result, corroborating previous results on music experience and laterality (Fuji & Oda, Citation2006; Jäncke et al., Citation1997), could suggest that musical training has a beneficial effect for body and movement control of the subdominant side, as learning and playing musical instruments involves movement coordination of both body sides (Kopiez et al., Citation2010). It could further be that such greater experience could result in an increased ability to apply open loop control movement also at slower tempi. Thus, this kind of repetitive movement task could be seen as a transfer effect from the ability to play musical instruments and relate to stronger equilaterality/lateral dexterity and more interhemispheric communication as well as increased symmetry in motor networks in musicians (e.g., Burunat et al., Citation2015; Schlaug et al., Citation1995).
With respect to the difference measure, a significant main effect for music experience was found. Less musically trained participants showed higher consistency in their dominant side’s velocity compared to their subdominant side, emphasizing the dominance of the dominant side in the normal population (Goble & Brown, Citation2008), while the highly musically trained group showed higher consistency in their subdominant side compared to their dominant side. In line with the results regarding the overall consistency measure described in the previous paragraph, proficiency in knowing how to play a musical instrument could be beneficial for movement control of the subdominant hand and potentially for better bimanual execution of motion (e.g., Kopiez et al., Citation2010). The result, however, that the sub-dominant side is (even) more consistent than the dominant hand, is somewhat unexpected, as one would rather assume both body sides to be similarly consistent. It could be speculated that professional musicians, due to their extensive bimanual training, are able to control their subdominant side even more accurately than their dominant side, at least related to maintaining a consistent speed in a circular movement sequence. Thus, future research could investigate whether this effect only occurs in our task or could be generalizable, or whether this result is instrument-specific (i.e., that players of certain groups of instruments, such as strings or keyboards, would perform differently).
While the overall velocity consistency of medium-level and high-level experience groups was high for both body sides, they showed an interesting pattern concerning the laterality: while the high-level group moved with more consistent velocity on their subdominant side, the medium-level participants showed more consistent velocity on their dominant side. The same pattern was shown in the difference measure. This could suggest that only with high music proficiency, this laterality effect toward the subdominant side occurs, while moderate music training increases velocity consistency on both body sides already. This result might bear potential implications for music practice and teaching, but would need further investigation and refinement to know more about possible transfer effects of such movement tasks on performance practice, as well as about possible influences of knowing how to play different kinds of instruments.
Dance experience only showed a marginal effect on the difference measure and no relationships with the overall consistency measure, hence not supporting the dance experience part of Hypothesis 2. It could be that the chosen movement was too abstract to have felt like a dance movement, thus participants with dance experience could have tried to perform the movement as dance by adding some expressivity and meaning to them. This could have resulted in more variability, and a larger number of trials/repetitions would have been required to decrease that variability. Results indicate that participants with no dance training tended to be more consistent on their subdominant side, while medium-level trained participants tended to be more consistent on their dominant side. Participants with high-level dance training tended to be similarly consistent on both body sides. Especially the latter result suggests that dance training could be beneficial for bilaterally balanced movement execution, which is in line with results by Ramsay and Riddoch (Citation2001) and Jola et al. (Citation2011). Furthermore, dance training exercises are usually performed with one side of the body or in one direction, followed by the opposite side/direction to achieve equilaterality. Thus, the result is consistent with training methodology, providing trained dancers with a potential advantage in the current task. It is therefore likely that a study including dancers with a wider range of experience (comparable to musicians in this study) might further support the laterality benefits of the dance system. However, the results regarding participants with no dance training versus participants with medium-level training remain unclear and unintuitive, as one would expect untrained participants to be more consistent on their dominant side. One possible explanation could be that only the existence of substantial dance training would impact the given task, but no to little training would result in more variable results. For future studies, other background variables, such as experience in certain sports involving lateral coordination, should be systematically gathered to pinpoint this result in more detail and be able to consider implications for dance practice.
Interestingly, the results did not indicate an effect of experience on tempo, so regardless of music and dance background, performances in the slow tempo range resulted in more inconsistent behavior compared to mid and fast tempo. This result is supportive of Hove et al. (Citation2010) results that found no differences between musicians and non-musicians, while they are not in line with studies that found that musicians outperform non-musicians (e.g., Krause et al., Citation2010; Repp & Doggett, Citation2007). Additionally, no effect of laterality on tempo was found either, so overall both left and right body sides performed similarly at each tempo level, while becoming more variable in speed at slower tempi.
An interesting question regarding these results is why music seems to have a stronger impact on this repetitive circular movement task than dance background. There is no clear indication for that, although it could be that a repetitive circular movement is relatively close to different instrumental movements, such as bowing motions in case of string instruments or body/instrument sway in case of woodwind instruments. Following this notion, it could be that dance background would have shown differences with a different type of movement, maybe a more complex or expressive one, as dancers might be used to more complex movement sequences and also focusing more on expressive, variable execution of movements than on a technical, repetitive execution. Maybe a less smooth and circular, but more accentuated movement or a more complex sequence displaying expressive content would have resulted in different outcomes in relation to dance experience. Another explanation for the differing results could be the varying ranges of experience in participants’ music and dance background, as there were only very few highly trained dancers in our sample and a much larger spread with respect to music training. A sample containing more highly trained dancers could possibly reflect a higher degree of motor learning and control and therefore result in outcomes closer to that of highly trained musicians. Furthermore, a future participant sample could control for the possible combination of music and dance experience to tease out effects of music or dance experience (i.e., participants with: large experience in music and no experience in dance; large experience in dance and no experience in music; large experience in both; no experience in either). Thus, the results warrant further studies, in particular using a similar paradigm with different kinds of movements and a larger range of dance experience and years of training.
In order to dive further into possible influences of music experience and instrument knowledge, participants’ instruments should be gathered in future studies. This would be particularly interesting regarding the laterality aspect, since instruments not only afford different movements – like more circular ones when playing, for instance, a string instrument, while more discrete, straight movements, such as playing piano or a brass instrument – some instruments also afford different movements with left and right side, in particular string instrument. As brain studies suggest differences in brain laterality between violinists and pianists (e.g., Burunat et al., Citation2015), it would be interesting to investigate in the future whether different instrumentalists are able to execute such transfer movements differently. This could not only encompass consistency movement, but also movement that relate to a wider scope of movement control and that could potentially offer benefits for musicians’ performance.
In the current study, we used velocity instead of, for instance, position data as a basis for calculating a consistency measure. Velocity gives an indication of smoothness of the execution, that is, moving at a constant speed. Position consistency, on the other hand, would provide an indicator of whether the participant was able to follow the exact same circle trajectory during each repetition. This could be seen as a less valuable and necessary skill when dancing, playing an instrument, or executing an athletic movement, compared to performing a repetitive movement at a constant speed. Such movement execution would rather relate to the ability to control the body and limbs and could help in executing expressive, accurate, and effective movements. While other movement types might require different descriptors, we have developed a seemingly meaningful feature for describing a repetitive circular movement representing an abstract dance-like routine. Future approaches, especially ones that use more elaborated movement sequences could employ more complex measures, for instance, based on time-series analysis or entropy (see Parrington & Ball, Citation2016).
Another approach to investigate this phenomenon would include analysis of the synchronization between the phase of auditory stimulus and the movement. While this would exceed the scope of this paper, foremost since we asked the participants to perform consistent cycles, while not explicitly asking them to synchronize to a specific part of the auditory stimulus, phase analysis would offer further insights into temporal regularity as well as into differences between experts and novices, as experts could be more consistent due to them being able to decouple their limps to a higher extent from each other (i.e., being able to move their limbs more independently). In order to fully investigate this question, an additional, (more) discrete auditory signal would be required to compare synchronization facilitation with the continuous signal we used in the current data collection as well as specifically instructing the participants to synchronize to the given auditory stimulus (see Varlet et al., Citation2012).
To conclude, this study investigated the interplay between tempo, body laterality, and experience in music and dance in a controlled dance-like movement routine was investigated. We developed consistency measures based on the velocity data that showed to be affected mostly by execution tempo and music experience. In particular, maintaining velocity consistency was more challenging at slow tempi than at faster tempi. Music proficiency had a positive effect on movement consistency, especially related to the subdominant body side, and on smaller laterality differences. Dance proficiency, on the other hand, resulted in no or only marginal effects, yet more research with a wider range of dance expertise levels is needed. Overall, the results show differences regarding movement consistency and body control abilities that could have potential implications on music instrument, dance, and maybe even sports practice and training. It could be thus beneficial for music and dance beginners to train specifically the subdominant hand and body side to increase bilateral dexterity, symmetry, and consistency by using movement tasks that require different levels of lateral coordination. Additionally, focusing on executing slow movement in a consistent manner could further increase motor control abilities. Moreover, such practice might reduce the risk for pain and injury.
Disclosure Statement
No potential conflict of interest was reported by the author(s).
Additional information
Funding
References
- Adams, J. A. (1971). A closed-loop theory of motor learning. Journal of Motor Behavior, 3(2), 111–149. https://doi.org/10.1080/00222895.1971.10734898
- Barry, N. H., & McArthur, V. (1994). Teaching practice strategies in the music studio: A survey of applied music teachers. Psychology of Music, 22, 44–55. https://doi.org/10.1177/0305735694221004
- Baudry, L., Seifert, L., & Leroy, D. (2008). Spatial consistency of circle on the pedagogic pommel horse: Influence of expertise. Journal of Strength and Conditioning Research, 22(2), 608–613. https://doi.org/10.1519/JSC.0b013e31816346f3
- Bernstein, N.A. (1967). The co-ordination and regulation of movements. Pergamon Press.
- Burger, B., & Toiviainen, P. (2013). MoCap Toolbox: A Matlab toolbox for computational analysis of movement data. In R. Bresin (Ed.), Proceedings of the 10th Sound and Music Computing Conference, Stockholm, Sweden (pp. 172–178). KTH Royal Institute of Technology.
- Burunat, I., Brattico, E., Puoliväli, T., Ristaniemi, T., Sams, M., Toiviainen, P. (2015). Action in perception: Prominent visuo-motor functional symmetry in musicians during music listening. PLoS ONE 10(9): e0138238. https://doi.org/10.1371/journal.pone.0138238
- Calvo-Merino, B., Grèzes, J., Glaser, D. E., Passingham, R. E., & Haggard, P. (2006). Seeing or doing? Influence of visual and motor familiarity in action observation. Current Biology, 16(19), 1905–1910. https://doi.org/10.1016/j.cub.2006.07.065
- Davids, K., Bennett, S., & Newell, K. (Eds.). (2006). Movement system variability. Human Kinetics.
- Dierks, T. A., & Davis, I. (2007). Discrete and continuous joint coupling relationships in uninjured recreational runners. Clinical Biomechanics, 22(5), 581–591.
- Ehrlenspiel, F. (2001). Paralysis by analysis? A functional framework for the effects of attentional focus on the control of motor skills. European Journal of Sports Science, 1(5), 1–11. https://doi.org/10.1080/17461390100071505
- Fraisse, P. (1982). Rhythm and tempo. In D. Deutsch (Ed.), The psychology of music (pp. 149–180). Academic Press.
- Fuji, S., & Oda, S. (2006). Tapping speed asymmetry in drummers for single-hand tapping with a stick. Perceptual and Motor Skills, 103(1), 265–272. https://doi.org/10.2466/pms.103.1.265-272
- Furuya, S., & Kinoshita, H. (2008). Expertise-dependent modulation of muscular and non-muscular torques in multi-joint arm movements during piano keystroke. Neuroscience, 156(2), 390–402. https://doi.org/10.1016/j.neuroscience.2008.07.028
- Gentile, A. M. (2000). Skill acquisition: Action, movement, and neuromotor processes. In J. H. Carr & R. B. Shepherd (Eds.), Movement science: Foundations for physical therapy (2nd ed., pp. 111–187). Aspen.
- Goble, D. J., & Brown, S. H. (2008). The biological and behavioral basis of upper limb asymmetries in sensorimotor performance. Neuroscience and Biobehavioral Reviews, 32, 598–610. https://doi.org/10.1016/j.neubiorev.2007.10.006
- Hove, M. J., Spivey, M. J., & Krumhansl, C. L. (2010). Compatibility of motion facilitates visuomotor synchronization. Journal of Experimental Psychology: Human Perception and Performance, 36, 1525–1534. https://doi.org/10.1191/026921501666288152
- Huys, R., Studenka, B. E., Rheaume, N. L., Zelaznik, H. N., & Jirsa, V. K. (2008). Distinct timing mechanisms produce discrete and continuous movements. PLoS Computational Biology, 4(4), e1000061.
- Jäncke, L., Schlaug, G., & Steinmetz, H. (1997). Hand skill asymmetry in professional musicians. Brain & Cognition, 34(3), 424–432.
- Jola, C., Davis, A., & Haggard, P. (2011). Proprioceptive integration and body representation: Insights into dancers' expertise. Experimental Brain Research, 213, 257–265. https://doi.org/10.1007/s00221-011-2743-7
- Kimmele, M. (2010). Lateral bias, functional asymmetry, dance training and dance injuries. Journal of Dance Medicine & Science, 14(2), 58.
- Konczak, J., van der Velden, H., & Jaeger, L. (2009). Learning to play the violin: Motor control by freezing, not freeing degrees of freedom. Journal of Motor Behavior, 41, 243–252.
- Kopiez, R., Galley, N., & Lehmann, A. C. (2010). The relation between lateralisation, early start of training, and amount of practice in musicians: A contribution to the problem of handedness classification. Laterality, 15(4), 385–414. https://doi.org/10.1080/13576500902885975
- Kopiez, R., Jabusch, H.-C., Galley, N., Homann, J.-C., Lehmann, A. C., & Altenmüller, E. (2012). No disadvantage for left-handed musicians: The relationship between handedness, felt constraints and performance-related skills in pianists and string players. Psychology of Music, 40(3), 357–384.
- Krause, V., Pollok, B., & Schnitzler, A. (2010). Perception in action: The impact of sensory information on sensorimotor synchronization in musicians and non-musicians. Acta Psychologica, 133, 28–37. https://doi.org/10.1016/j.actpsy.2009.08.003
- Lopresti-Goodman, S. M., Richardson, M. J., Silva, P. L., & Schmidt, R. C. (2008). Period basin of entrainment for unintentional visual coordination. Journal of Motor Behavior, 40(1), 3–10.
- Marshall, J. (2006). Dancing the elemental body: Butoh and body weather: Interviews with Tanaka Min and Yumi Umiumare. Performance Paradigm, 2, 54–73.
- Moelants, D. (2002). Preferred tempo reconsidered. In C. Stevens, D. Burnham, G. McPherson, E. Schubert, & J. Renwick (Eds.), Proceedings of the 7th International Conference on Music Perception and Cognition, Sydney, Australia (pp. 580–583). Causal Productions.
- Newell, K. M., Deutsch, K. M., Sosnoff, J. J., & Mayer-Kress, G. (2006). Variability in motor output as noise. A default and erroneous proposition? In K. Davids, S. Bennett, & K. Newell (Eds.). Movement system variability. Human Kinetics.
- Newell, K. M., & Ranganathan, R. (2009). Some contemporary issues in motor learning. In D. Sternad (Ed.), Progress in motor control (Vol. 629, pp. 395–404). New York: Springer.
- Nikodelis, T., Kollias, I., & Hatzitaki, V. (2005). Bilateral inter-arm coordination in freestyle swimming: Effect of skill level and swimming speed. Journal of Sports Sciences, 23(7), 737–745. https://doi.org/10.1080/02640410400021955
- Oldfield, R. C. (1971). The assessment and analysis of handedness: The Edinburgh inventory. Neuropsychologia, 9, 97–113. https://doi.org/10.1016/0028-3932(71)90067-4
- Palmer, C., & van de Sande, C. (1995). Range of planning in music performance. Journal of Experimental Psychology: Human Perception & Performance, 21(5), 947–962. https://doi.org/10.1037/0096-1523.21.5.947
- Parrington, L., & Ball, K. (2016). Biomechanical considerations of laterality in sport. In F. Loffing, N. Hagemann, B. Strauss, & C. MacMahon (Eds.), Laterality in sports: Theories and applications. Academic Press.
- Pierce, M. A. (2006). The effects of learning procedure, tempo, and performance condition on transfer of rhythm skills in instrumental music. Journal of Research in Music Education, 40(4), 295. https://doi.org/10.2307/3345837
- Preatoni, E., Hamill, J., Harrison, A. J., Hayes, K., Van Emmerik, R. E. A., Wilson, C. & Rodano, R. (2013). Movement variability and skills monitoring in sports. Sports Biomechanics, 12(2), 69–92. https://doi.org/10.1080/14763141.2012.738700
- Ramsay, J. R. E., & Riddoch, M. J. (2001). Position-matching in the upper limb: professional ballet dancers perform with outstanding accuracy. Clinical Rehabilitation, 15(3), 324–330.
- Repp, B. (1999). Control of expressive and metronomic timing in pianists. Journal of Motor Behavior, 31(2), 145–164. https://doi.org/10.1080/00222899909600985
- Repp, B. H. (2003). Rate limits in sensorimotor synchronization with auditory and visual sequences: The synchronization threshold and the benefits and costs of interval subdivision. Journal of Motor Behavior, 35, 355–370. https://doi.org/10.1080/00222890309603156
- Repp, B. H. (2005). Sensorimotor synchronization: a review of the tapping literature. Psychonomic Bulletin & Review, 12, 969–992. https://doi.org/10.3758/BF03206433
- Repp, B. H. (2011). Comfortable synchronization of cyclic drawing movements with a metronome. Human Movement Science, 30(1), 18–39. https://doi.org/10.1016/j.humov.2010.09.002
- Repp, B. H., & Doggett, R. (2007). Tapping to a very slow beat: A comparison of musicians and nonmusicians. Music Perception, 24(4), 367–376.
- Repp, B. H., & Penel, A. (2004). Rhythmic movement is attracted more strongly to auditory than to visual rhythms. Psychological Research, 68, 252–270.
- Repp, B. H., & Steinmann, S. R. (2010). Simultaneous event-based and emergent timing: Synchronization, continuation, and phase correction. Journal of Motor Behavior, 42, 111–126.
- Repp, B. H., & Su, Y.-H. (2013). Sensorimotor synchronization: A review of recent research (2006-2012). Psychonomic Bulletin & Review, 20, 403–452. doi: 10.3758/s13423-012-0371-2
- Schlaug, G., Jäncke, L., Huang, Y., Staiger, J. F., & Steinmetz, H. (1995). Increased corpus callosum size in musicians. Neuropsychologia, 33, 1047–1055.
- Schmidt, R. A. (1975). A schema theory of discrete motor skill learning. Psychological Review, 82(4), 225–260. https://doi.org/10.1037/h0076770
- Schmidt, R. A., Lee, T., Winstein, C., Wulf, G., & Zelaznik, H. (2019). Motor control and learning. A behavioral emphasis (6th ed.). Human Kinetics.
- Schoonderwaldt, E., & Altenmüller, E. (2014). Coordination in fast repetitive violin-bowing patterns. PLoS One, 9(9), e106615.
- Simmons, R. (2005). Neuromuscular responses of trained ballet dancers to postural perturbations. International Journal of Neuroscience, 115, 1193–1203. https://doi.org/10.1080/00207450590914572
- Studenka, B. E., & Zelaznik, H. N. (2011). Circle drawing does not exhibit auditory–motor synchronization. Journal of Motor Behavior, 43(3), 185–191.
- Thullier, F., & Moufti, H. (2004). Multi-joint coordination in ballet dancers. Neuroscience Letters, 369(1), 80–84.
- van den Tillaar, R., & Ettema, G. (2006). A comparison between novices and experts of the velocity-accuracy trade-off in overarm throwing. Perceptual and Motor Skills, 103(2), 503–514. https://doi.org/10.2466/pms.103.2.503-514
- van Noorden, L., & Moelants, D. (1999). Resonance in the perception of musical pulse. Journal of New Music Research, 28, 43–66. https://doi.org/10.1076/jnmr.28.1.43.3122
- Varlet, M., Marin, L., Issartel, J., Schmidt, R. C., & Bardy, B. G. (2012). Continuity of visual and auditory rhythms influences sensorimotor coordination. PloS one, 7(9), e44082.
- Veale, J. F. (2014). Edinburgh Handedness Inventory - Short Form: A revised version based on confirmatory factor analysis. Laterality, 19, 164–177. https://doi.org/10.1080/1357650X.2013.783045
- Wöllner, C., & Cañal-Bruland, R. (2010). Keeping an eye on the violinist: Motor experts show superior timing consistency in a visual perception task. Psychological Research, 74, 579–585.
- Zendel, B. R., Ross, B., & Fujioka, T. (2011). The effects of stimulus rate and tapping rate on tapping performance. Music Perception, 29, 65–78.