Abstract
The characterization of callosal white matter is crucial for understanding the relationship between brain structure and bimanual motor function. An earlier literature review established this. With advancements in neuroimaging and data modeling, we aim to provide an update on the existing literature. Firstly, we highlight new CC parcellation approaches, such as functional MRI- and atlas-informed tractography and in vivo histology. Secondly, we elaborate on recent insights into the CC’s role in bimanual coordination, drawing evidence from studies on healthy young and older adults, patients and training-related callosal plasticity. We also reflect on progress in the field and propose future perspectives to inspire research on the underlying mechanisms of structural-functional interactions.
Introduction
Since the field of neuroscience is moving forward at a fast rate with enormous advancements being made regarding neuroimaging techniques and data modeling, new knowledge is created quickly. White matter (WM) in particular has attracted increasing scientific attention in recent times, supporting the working hypothesis that characterizing WM in the brain in great detail is critical to our understanding of brain function and behavior. With this trend moving forward, it is necessary to update our previous literature review on the role of the corpus callosum (CC), the largest WM structure in the brain, in bimanual motor control (Gooijers & Swinnen, Citation2014). Based on the literature search performed in 2014, with a large focus on the role of specific subsections of the CC for bimanual performance, we concluded that interhemispheric fibers in the CC subpart (likely) connecting bilateral premotor and motor areas are without doubt of great relevance. However, multiple works also indicated the importance of more anterior (genu) and more posterior (splenium) CC subparts. So, although some success was obtained in associating specific CC subregions with different expressions of bimanual motor control, conclusive statements could not be made. Several suggestions for improvement were put forward: (I) controlling various nonspecific factors influencing the CC, (II) recognizing task features and strategies, (III) better agreement among CC partitioning schemes, (IV) further technological advances in diffusion metrics, techniques and tracking methods, and (V) integration of insights across neuroimaging and neurophysiological approaches. Since then, progress has been made mainly with regards to suggestions III-IV which we will discuss further on. This update aims to further increase our understanding of the specificity of structure-function relationships in systems neuroscience and, more specifically, the relationship between callosal substructures and bimanual coordination.
Subdivision Schemes of the CC
In our search for understanding interhemispheric communication and its role in motor behavior in particular, parcellation of the CC and the characterization of its microstructural properties is of critical importance. Since our previous report (Gooijers & Swinnen, Citation2014), new methods and models have been developed and applied to further improve the subdivision of the CC along its length, which has resulted in new approaches. More specifically, whereas previously geometrically informed partitioning schemes took the overhand, nowadays more and more work on callosal partitioning refers to functional meaning based on a) an atlas or subject-specific functional MRI (fMRI) data (even to the level of individual body parts in callosal motor tracts) and/or b) in vivo microstructural characteristics (i.e., in vivo histology). We will address both methods in the section below.
fMRI- and Atlas-Informed Callosal Tractography
As included in our previous literature review (Gooijers & Swinnen, Citation2014), we wish to emphasize that all callosal partitioning schemes are intended to reveal a functionally-correct subdivision. However, how scientists arrive at these functionally-relevant segments has evolved considerably over time. Particularly the subdivision schemes which are based on a combination of (individual) fMRI and WM tractography manage to assign callosal fibers to their intended function effectively. This trend had already started in 2014 when we published our first literature review, and has continued since. For example, in 2019, Domin and Lotze applied a connectivity-based approach with functional seeds obtained from the Human Connectome Project to achieve functionally relevant regions (Human Connectome Project; see Van Essen and Ugurbil (Citation2013) (dataset) and Van Essen et al. (Citation2013)). In particular, they were able to recover body part-specific regions of interest within the area of the CC containing fibers conveying motor-related information. Spatially distinct subregions of the CC were created for the tongue/larynx (anterior inferior midbody), head/face (inferior posterior midbody - medial), lower limbs (superior posterior midbody), and upper limbs (inferior posterior midbody). Domin and Lotze (Citation2019) argued that one possible explanation for these high-level subdivisions could be the varying requirements for interhemispheric information transfer to allow efficient movement. For example, a large number of fibers with large diameters (found in the posterior body of the CC) might be of critical importance for faster interhemispheric communication while performing skills that require specific coordination between both hands. To move forward, a critical step is to obtain biologically meaningful measures from the interhemispheric WM tracts (that are specific to different parts of the body), and then establish a correlation between these metrics and behavioral measures, such as bimanual performance.
In our recently published work (Gooijers et al., Citation2021), we have made significant steps in this direction. Specifically, we performed interhemispheric WM tractography based on seeds obtained from individual fMRI activations in response to hand and foot flexion-extension movements. In line with Domin and Lotze (Citation2019), although in subject-space, we revealed callosal hand and foot fibers in the posterior body with callosal hand fibers localized more inferior relative to callosal foot fibers (see ) (Gooijers et al., Citation2021). Such studies clearly demonstrate the existence of a fine-grained parcellation that matches with the level of individual effectors. The development of such newly proposed regions in the CC allows for the accurate mapping of structural connectivity between bilateral motor cortices and to determine the fine-grained microstructure of these functionally-relevant fiber pathways.
FIGURE 1. Copied with permission from Gooijers et al. (Citation2021). Tracked callosal motor fibers (red: hand M1, blue: Feet M1, green: SMA) are visualized on individual FA maps. The in-set shows callosal motor fibers in a midsagittal view.
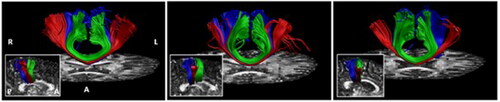
Finally, it is noteworthy to highlight a newly available atlas that includes partitioning of the CC in seven subregions in vivo in humans (Radwan et al., Citation2022). Segments refer to prefrontal, premotor, motor, sensory, parietal, occipital and temporal areas and are obtained through the application of probabilistic spherical deconvolution tractography. Thus, whereas previous atlases for the human brain were limited to the genu, callosal body and splenium, improvements in fiber tracking techniques in combination with the integration of their functional meaning, have now resulted in an alternative segmentation of callosal subparts (see ). The use of functional regions of interest from such a high-quality brain atlas offers unique opportunities to advance research into interactions between brain structure and function. This especially yields new opportunities when it is not feasible to incorporate individual fMRI data to inform interhemispheric WM tractography.
FIGURE 2. Copied with permission from Radwan et al. (Citation2022). Multiple projections of the semitransparent MNI pial surface. A-B: prefrontal CC, C-D: PMC and SMA CC, E-F: motor CC, G-H: sensory CC, I-J: parietal CC, K-L: occipital CC, M-N: temporal CC.
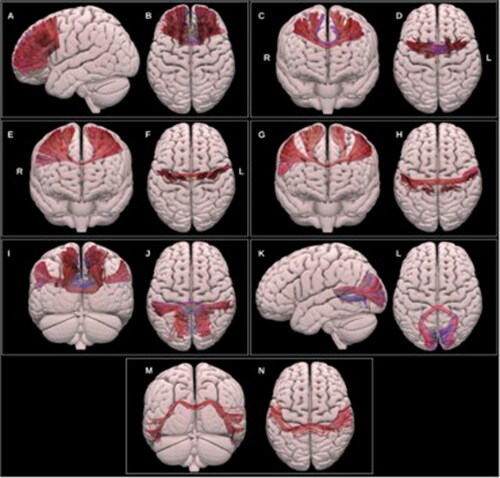
Callosal Subdivisions Based on in Vivo Histology
In 2019, in vivo histology at high resolution was used to suggest callosal partitioning. More specifically, by applying T1 relaxometry, Lee et al. (Citation2019) determined T1 values across the length of the CC, reflecting myelinated axonal size and myelin density. In accordance with ex vivo histology work of Aboitiz et al. (Citation1992), results revealed higher T1 values in the more posterior segments of the CC, whereas lower T1 values were present in the anterior half of the CC. Furthermore, compared to a geometric partitioning scheme such as the one proposed by Witelson (Citation1989), the authors argue that their methods could result in an improved dissociation between callosal parts, particularly at the level of the splenium. Interestingly, regions of interest that were created based on the callosal T1 values appeared to project to functionally meaningful cortical areas, as determined by means of probabilistic diffusion tractography and therefore could reflect an interesting partitioning method. As this method (i.e., T1 relaxometry) makes use of microstructural properties of the callosal WM (i.e., axonal density and diameter), and previously mentioned fMRI-informed partitioning schemes make use of diffusion tractography, these methods are complementary. More specifically, whereas the first informs us about communication functionality (e.g., large axonal diameter reflecting high conduction velocity) of callosal subparts, the latter informs about functional directionality. Thus, the work by Lee et al. (Citation2019) provides a technique that allows to partition the CC based on microstructural properties that might underlie the tractography-based subdivision schemes that are proposed to date.
Thus, compared to the work that was included in our literature review in 2014, progress has been made in segmenting the CC by function. Specifically, the use of individual fMRI, or atlas based fMRI if the former is not available, is recommended over the application of geometric subdivision schemes. Moving from geometrically informed partitioning schemes to functionally informed CC subdivisions is particularly helpful for understanding the role of callosal white matter fibers in bimanual coordination as it will increase the specificity of the relationship. For example, being able to extract the parietal and occipital fibers from the splenium of the CC, is of interest when we aim to explain performance on a visually guided bimanual coordination task. Also, as the SMA and premotor cortex are known to be involved in bimanual coordination performance (Swinnen, Citation2002), white matter tracts particularly connecting these bilateral secondary motor areas are of interest to directly link with bimanual motor performance. Also, by applying advanced imaging techniques to obtain biologically meaningful WM metrics (such as axonal diameter, density, myelin content) across the entire length of the CC, a more optimal mapping can be proposed. Evidently, when investigating the role of WM in particular subregions of the CC, one should be aware of the applied subdivision, as this may impact the interpretations of the findings substantially. Hence, in the following sections, in which we review recent literature on the association between callosal WM and bimanual coordination performance, we are mindful of the applied segmentation scheme.
The Role of the CC in Bimanual Coordination: Structural-Functional Interactions
Having covered the evolution of research on callosal segmentations over the past years, we will now discuss the functional role of interhemispheric WM pathways for performing bimanual tasks based on studies published since 2014. Evidence for its particular importance has emerged from studies on healthy (young) adults, patient populations, the aging population, and in the context of bimanual training.
Healthy (Young) Adults
As extensively described in our previous review (Gooijers & Swinnen, Citation2014), a wide range of studies have explored the relationship between motor behavior and WM microstructural properties. Although these studies have tremendously moved the field forward, emphasizing the important role of the CC in bimanual motor control, the biological interpretation hereof is limited. That is, since fractional anisotropy (FA), a typically applied diffusion-tensor-based metric, is sensitive to multiple tissue properties, it remains unknown what aspects of callosal WM in particular contribute to bimanual motor behavior. Therefore, in our recent work we investigated the particular importance of specific WM microstructural metrics such as axonal density and apparent axonal radius to shed new light on the structural underpinnings of the relationship between callosal WM and bilateral motor behavior (Gooijers et al., Citation2021). Findings revealed a possible association between larger apparent axonal radii of callosal motor fibers connecting functionally informed bilateral hand areas (using fMRI) and faster motor speed while tapping both hands simultaneously in an in-phase mode. Furthermore, faster normalized reaction times for bilateral foot movements were on trend level related to higher axonal density values in callosal foot motor fibers (informed by individual fMRI data). Although FA additionally appeared very sensitive to pick up these associations, apparent axonal radius for hand fibers, and axonal density for foot fibers (i.e. providing information on fiber geometry) are promising to explain the neurobiological source behind the observed findings in FA. This, undoubtedly contributes to deeper insights into the mechanisms underlying brain-behavior interactions.
Also, recently Lazari et al. (Citation2021) conducted an assessment of WM-behavior relationships by means of FA and metrics that are more sensitive to myelin (such as Magnetization Transfer (MT), R1 (longitudinal relaxation rate), R2 (transverse relaxation rate)). First, in line with previous studies, a robust relationship between FA within the anterior half of the CC (no callosal subdivision applied) and bimanual coordination was reported. More specifically, lower FA values were linked to better performance, i.e. shorter total time needed for 30 taps, on an alternating finger tapping task (Lazari et al., Citation2021). It is, however, noteworthy that literature regarding the specific direction of the relationship between FA and motor behavior is still equivocal. Second, the authors provided further evidence against the idea of a generalizable microstructural signature for behavioral performance. For example, whereas FA was linked to alternating bimanual finger tapping, other modalities more sensitive to myelin in particular (i.e., MT, R1 and R2) obtained from the CC were not. As no general pattern was found across the WM metrics of interest, one could argue that myelination of interhemispheric axons is not the driving mechanism for this structure-function relationship. Instead, it is possible that fiber orientation (i.e., characterizing angular variation of fibers (Zhang et al., Citation2012)) within the CC contributes to the significant association between FA and bimanual performance. Such works emphasize the necessity for advanced multi-modal imaging to detect the underlying biological sensitivities contributing to (bimanual) motor behavior.
During the past decade, the implementation of noninvasive brain stimulation techniques (such as advanced transcranial magnetic stimulation (TMS) measures) in combination with improved structural quantification methods of the CC have also served to shed more light on the complex structure-function relationship between CC substructures and bimanual performance. In that regard, Wahl et al. (Citation2016) reported that in healthy participants, higher FA between callosal hand motor fibers and stronger short-interval interhemispheric inhibition was associated with higher temporal offsets (i.e., over- or under estimation of the required relative phase) between hands in the out-of-phase conditions while performing a visually cued bimanual temporal coordination task. Recently, a new measure of transcallosal conduction delay to study hemispheric asymmetries and cortical connectivity was introduced by Bortoletto et al. (Citation2021). Transcallosal conduction delay was quantified by the joined application of TMS and electroencephalography (EEG). Specifically, a single biphasic TMS pulse over the left or right hemisphere, targeting the primary motor cortex (M1) or the dorsal premotor cortex (PMd), was applied. This pulse generated a secondary neural response in contralateral homologous regions connected through the CC, i.e. TMS-evoked potentials. These potentials were recorded with high temporal precision through EEG. The amplitude and latency of this secondary response reflect the strength and conduction delay of the connection, respectively. The authors observed an inverse relationship between transcallosal conduction delay between M1s and mean diffusivity (MD) of the callosal body (CC was divided into three segments). More specifically, the higher the MD, which describes the amount of free diffusion, the shorter the latency or transcallosal conduction delay between both M1s. Furthermore, the authors revealed that this new measure of transcallosal conduction delay could predict (temporal) bimanual performance on an in-phase bimanual thumb-opposition task. Interestingly, shorter conduction delays between homologous motor areas did not lead to better performance per se, but rather the asymmetry in conduction delays between both M1s, i.e. shorter delays from M1 in the dominant to the nondominant hemisphere and longer delays from M1 in the nondominant to dominant hemisphere, was predictive of better bimanual performance, namely shorter interhand intervals between finger taps. Hence, their findings appear to support the statement that temporal features in the communication between bilateral cortices are critical for the bimanual motor performance outcome, which in turn is made possible through WM tracts in the body of the CC.
In summary, studies covering the (young) adult population underscore the relationship between bimanual performance and interhemispheric WM microstructural properties mainly of tracts connecting (limb-specific) callosal motor fibers as well as more anterior subparts of the CC (Gooijers et al., Citation2021; Lazari et al., Citation2021; Wahl et al., Citation2016). It is noteworthy that the direction of the effects is not consistent, i.e. higher (Gooijers et al., Citation2021) as well as lower FA levels are linked with better performance (Gooijers et al., Citation2021; Lazari et al., Citation2021; Wahl et al., Citation2016). In light of these equivocal findings, it is important to note that attempts were made to better understand the importance of WM from a biological perspective (Gooijers et al., Citation2021; Lazari et al., Citation2021). That is, metrics that move beyond FA such as axonal density, apparent axonal radius and measures more sensitive to myelin, appear less sensitive to pick up associations with bimanual performance, but provide new information suggesting that varying biological effects may define WM structure and behavior interactions.
Pathology
Since our work in 2014, no works have been published on primary pathologies affecting the CC such as callosal agenesis or partial/complete callosotomy, in which a relationship with bimanual function has been studied. There is, however, new evidence on the role of callosal connections that stems from secondary pathologies in which the CC is (in)directly affected as a ‘consequence’ of a more general pathological condition. Overall, these findings are in accordance with the statement that the CC is of critical importance for bimanual performance. This is demonstrated in recent works (in addition to studies that were already included in Gooijers and Swinnen (Citation2014)) through significant associations between WM measures such as FA, MD, axial diffusivity (AD), radial diffusivity (RD), cross-sectional area and fiber/streamline count of callosal sections and bimanual performance (deficits) in a variety of pathologies, e.g., cerebral palsy (Hung et al., Citation2019; Robert et al., Citation2021; Weinstein et al., Citation2014; Weinstein et al., Citation2015), Tourette’s syndrome (Martino et al., Citation2017, Citation2019), preterm birth (Hollund et al., Citation2018), stroke (Hawe et al., Citation2013; Lench et al., Citation2020; Varghese et al., Citation2022), multiple sclerosis (Bonzano et al., Citation2014), and autism spectrum disorders (Barbeau et al., Citation2015). Each study is discussed in detail below. Besides, being able to determine in more detail which interhemispheric fibers are involved in bimanual tasks reflecting everyday life activities, is of clinical importance. For example, think of predicting bimanual function and/or recovery after a stroke or disease progression in patients with multiple sclerosis, as well as using this knowledge as an important tool for delineating functionally relevant interhemispheric fibers in case of surgical planning.
In children with cerebral palsy, Hung et al. (Citation2019) observed an association between the temporal performance on a kinematic bimanual drawer-opening task and CC diffusion properties. Specifically, AD of the whole CC as well as number of voxels and number of streamlines of the splenium (according to the Witelson (Citation1989) CC subdivision) were negatively correlated with (a) total movement time and (b) goal synchronization (i.e. time difference between the offset of the right and left hand) of the bimanual task. Hence, better temporal bimanual coordination performance, i.e. shorter total movement time and shorter time differences, was associated with higher AD of the whole CC and higher number of voxels and streamlines in the splenium in particular. The association between AD and temporal performance was somehow surprising, since previous studies investigating the link between microstructural properties of the CC and bimanual coordination in cerebral palsy failed to indicate a relationship between AD and bimanual task performance (Weinstein et al., Citation2014). However, Weinstein et al. (Citation2014) observed that a higher number of fibers in the splenium (according to the Witelson (Citation1989) CC subdivision) correlated with better scores on the assisting hand assessment. More recently, Robert et al. (Citation2021) found that bimanual function in children with cerebral palsy, measured as scores on the assisting hand assessment, correlated positively with (a) the number of streamlines of the whole CC as well as of the genu, midbody and splenium (according to the Witelson (Citation1989) CC subdivision) and with (b) FA values of the whole CC and genu, but not in the midbody and splenium. This is in line with the work of Weinstein et al., who observed that better assisting hand assessment scores were associated with lower MD of the genu and midbody (Weinstein et al., Citation2015), and increased number of fibers in the splenium (Weinstein et al., Citation2014). Overall, these studies suggested that the microstructural organization across the CC in children with cerebral palsy is associated with bimanual function.
Robert et al. (Citation2021) also investigated the relationship between callosal microstructural organization and behavioral improvements in bimanual function as a result of intensive rehabilitation in children with cerebral palsy. The authors found that therapy-related behavioral changes in assisting hand assessment scores correlated with (a) the number of streamlines for the whole CC, midbody and splenium when therapy consisted of 90 h constraint-induced movement therapy and (b) FA of the midbody when therapy consisted of 90 h of hand-arm bimanual intensive therapy (Robert et al., Citation2021). Specifically, children demonstrating a reduced number of streamlines in the CC, showed larger (constraint-induced movement) therapy-related benefits on bimanual function.
Martino et al. (Citation2017) investigated the link between bimanual motor function and WM integrity in adults with Tourette syndrome. Notably, although patients demonstrated higher FA values in interhemispheric WM tracts connecting bilateral M1 and bilateral supplementary motor area (SMA) obtained by means of constrained spherical deconvolution, neither for the patient nor the control group was there a significant association between FA values of these WM tracts and bimanual coordination performance. More recent work of the same group similarly showed higher callosal FA values in patients with Tourette syndrome relative to controls, and additionally revealed that the higher the FA value between SMAs, which is reflective of better structural organization of these transcallosal connections, the lower the motor timing accuracy of the right hand on the bimanual version of motor timing sequential task (Martino et al., Citation2019). The authors argue that the pathology is characterized by abnormal growth of callosal WM as reflected by higher FA values, which accelerates interhemispheric transfer, which in turn results in suboptimal movement lateralization, also in a bimanual context.
Hollund et al. (Citation2018) examined the relationship between WM microstructure and motor function, more specifically fine and gross motor function, in young adults born preterm with very low birth weight in comparison with a group of term-born controls. Considering the scope of this review, we will only discuss results related to the triangle test from the movement assessment battery for children-2 (MABC-2) as this test assesses bimanual coordination. The goal of this task is to form a triangle out of three strips, nuts and bolts with both hands as fast as possible. The authors observed that lower FA in callosal WM tracts connecting bilateral M1s was associated with lower performance on the triangle task in adults with very low birth weight. Of note, this association was found in the lateral parts of these fibers characterized by more crossing fibers (corresponding with the centrum semiovale) and not in the medial parts of these fibers known to have less crossing fibers. This study implicates that inter-individual variability in bimanual function has structural correlates in young adults with very low birth weight, particularly in lateral callosal WM fibers connecting bilateral M1s.
Movement dysfunction observed in patients with unilateral brain injury may be associated with injury-related changes in callosal WM tracts. In that regard, Hawe et al. (Citation2013) extracted FA values and cross-sectional volume of five callosal regions (according to Hofer and Frahm (Citation2006)), in adult and pediatric hemiplegia patients as well as in age-matched controls. In this study, the timing of injury affected callosal microstructure and this was associated with involuntary mirror movements during a bilateral torque task in patients with pediatric hemiplegia only. More specifically, in the pediatric hemiplegia group, reductions in volume of the CC region projecting to premotor and SMAs as well as M1s were associated with greater levels of involuntary interlimb coupling. These findings may explain the greater bimanual dysfunction and difficulties observed in patients with pediatric as compared to adult hemiplegia.
With respect to chronic stroke, Lench et al. (Citation2020) explored the relationship between injury-related changes in callosal WM and bimanual function assessed using kinematic measures during two reaching and grasping tasks. FA and mean kurtosis (MK) were extracted from three CC tracts connecting bilateral (1) SMAs, (2) pre-SMAs and (3) M1s obtained through the application of multi-shell, multi-tissue constrained spherical deconvolution. The authors found associations between both FA and MK CC metrics, especially the portion of the CC connecting SMAs, and kinematic measures (i.e. hand asymmetry and trunk displacement) during the bimanual tasks in patients with chronic stroke. This suggests that the microstructural organization of the CC fibers connecting secondary motor areas following chronic stroke contributes to the observed disruptions of cooperative coordination of both hands. Another study investigated whether callosal WM microstructure can predict bimanual performance on a letter-envelope task in chronic stroke survivors (Varghese et al., Citation2022). Microstructural status was quantified with FA of five callosal regions according to the Hofer and Frahm (Citation2006) parcellation scheme. Callosal FA of fibers connecting bilateral prefrontal and premotor area was associated with movement time in chronic stroke survivors who selected a bimanual strategy to perform the letter-envelope task. More specifically, lower FA values in the frontal regions of the CC were predictive of slower movement times. No association was found for patients who chose a unimanual strategy. These results may pave the way for the use of callosal microstructural properties as a predictive tool for bimanual function in chronic stroke survivors.
Declines in upper limb and/or bimanual motor function are not unusual in patients with multiple sclerosis. Hence, in a population of patients with multiple sclerosis, Bonzano et al. (Citation2014) investigated whether a 2-month upper limb intervention consisting of task-oriented exercises (both unimanual and bimanual) as compared to a control intervention consisting of passive mobilization of the upper limb joints, can change motor performance on various unimanual tasks as well as a finger sequencing task involving both hands. Furthermore, DTI metrics such as FA, MD, AD and RD were obtained from the entire CC to examine intervention-related changes in WM microstructure. At a behavioral level, performance on the bimanual coordination task remained stable from pre to following the upper limb intervention. However, bimanual performance decreased during the control intervention. Similarly, whereas callosal WM microstructure declined, i.e. a decrease in FA and an increase in RD, from pre to post in the control intervention, this was preserved following the upper limb intervention. Authors argue that during the voluntary movement training in addition to sensorimotor fibers which are also important for passive movement, also frontal, parietal and posterior callosal areas connected by frontal and posterior CC fibers are active. This might have resulted in more structural plasticity processes. Although possible, note that a direct structure-function relationship was not established in this study, and that no CC parcellation was applied.
Finally, callosal WM properties, i.e. relative CC size and diffusion weighted imaging (DWI) metrics FA, MD, RD and AD, were linked to behavioral interhemispheric performance in adults with autism spectrum disorder (Barbeau et al., Citation2015). Behavioral interhemispheric performance was tested with the Purdue pegboard test in which two variants to assess bimanual coordination were used. In adults with autism spectrum disorder, the size of the CC area connecting bilateral occipital areas was negatively associated with bimanual performance on the Purdue pegboard task; whereas the size of more anterior (motor) CC regions was positively associated with performance on the Purdue pegboard test in healthy controls. Hence, the authors argue that for adults with autism spectrum disorder, CC regions projecting to visual areas play a dominant role in bimanual visuomotor processing, i.e. patients use an alternative, more perceptual strategy; whereas in healthy controls, bimanual task performance is related to more anterior and motor-related CC regions.
In conclusion of the pathology section, the contradiction in reported findings regarding the direction of the effects is noticeable. Whereas in the majority of the works “better” or more WM, reflected as either an increase in FA (Hollund et al., Citation2018; Robert et al., Citation2021; Varghese et al., Citation2022), and/or a greater callosal cross-sectional area (Hawe et al., Citation2013), a larger number of WM streamlines or fibers (Hung et al., Citation2019; Robert et al., Citation2021; Weinstein et al., Citation2014), or a decrease in the diffusion-related measures (Weinstein et al., Citation2015) (such as MD, AD, RD), is related to better/improved bimanual performance, other works relate the same WM measures to poorer/impaired bimanual performance (Barbeau et al., Citation2015; Hung et al., Citation2019; Lench et al., Citation2020; Martino et al., Citation2019). The sources of such discrepancies may be manifold. For example, there are differences in the bimanual tasks at hand, the pathology of interest, the age of the study population, the adopted techniques and dependent variables quantifying WM micro- and macrostructure.
Remarkable is the application of WM metrics obtained only through traditional diffusion imaging and modeling techniques. More specifically, with the exception of a few works applying constrained spherical deconvolution tractography (Lench et al., Citation2020; Martino et al., Citation2017, Citation2019), these studies have all adopted the diffusion tensor model to inform tractography and obtain traditional diffusion metrics such as FA, MD, AD and RD. Over the past years, however, it has become increasingly clear that the diffusion tensor model does not perform optimally in voxels including multiple fiber orientations, and that the traditional metrics are not sufficiently able to capture the underlying microstructural characteristics of WM (Assaf et al., Citation2013; Beaulieu, Citation2009; Jones et al., Citation2013). Thus, when applying these traditional models and metrics in a patient population, there is limited information on what specific biological aspects of WM impairments might contribute to compromised bimanual function. That is, it remains unclear how the pathology has affected the callosal WM (e.g., decrease in myelin content, decrease in axonal density, axonal swelling, cell death), and therefore what particular aspects of callosal WM drive suboptimal interhemispheric transfer of information, and consequently bimanual performance. Evidently, this limits statements on the association between callosal WM and bimanual motor performance. As a result of technological advancements (e.g., high angular resolution diffusion imaging (Tuch et al., Citation2002), diffusion MRI with multiple diffusion weightings (multi-shell MR imaging), diffusion weighted STimulated Echo Acquisition Mode (STEAM (De Santis et al., Citation2016)) and image analyses that bypass traditional diffusion tensor limitations (for tractography) (e.g., Neurite Orientation Dispersion and Density Imaging (Zhang et al., Citation2012), Composite Hindered and Restricted Model of Diffusion (Assaf & Basser, Citation2005), Constrained Spherical Deconvolution (Raffelt et al., Citation2017; Tournier et al., Citation2004) allowing for Fixel-Based Analysis (FBA) (Raffelt et al., Citation2017)), it is nowadays possible to quantify detailed characteristics of WM in vivo which are more specific to neurobiologically interpretable tissue properties. Importantly, many of such advanced analyses methods can be applied to diffusion protocols that run on typical MR systems (3 T) used for clinical work.
Finally, whereas some studies identified bilateral motor areas through fMRI and/or the use of an atlas, and applied these as seeds for WM tractography (Lench et al., Citation2020; Martino et al., Citation2017, Citation2019), a division of the CC into three or maximum five (Hofer & Frahm, Citation2006) segments was often adopted in the past work. The use of such segmentation approaches based on geometry, to a certain extent disregarding function, hampers the mapping of WM callosal structure to bimanual motor function. Hence, at the moment it is difficult to make specific statements regarding the role of functionally-relevant callosal subregions in the control of particular bimanual actions based on the existing patient work. To resolve this problem, the use of functional regions of interest, e.g., connecting bilateral SMAs and/or M1s, as was done by Lench et al. (Citation2020), and Martino et al. (Citation2017, Citation2019), is preferred and will undoubtedly move the field of research forward.
Age-Related Callosal Changes
In the context of aging, there is converging evidence of bimanual performance detriments in bimanual coordination with advancing age. Although some studies reported a link between these performance detriments on the one hand and a deterioration of CC structures on the other hand (for a review, see Gooijers & Swinnen, Citation2014), it is fair to say that this relationship is rather complex. Moreover, studies on WM microstructural properties in general, and in the aging brain in particular (because of the age-related WM atrophy), face considerable methodological limitations. More specifically, diffusion tensor models used in those studies are confounded by partial volume effects in voxels with complex fiber geometries (Jeurissen et al., Citation2013). To overcome this limitation, a statistical analysis framework, namely Fixel-Based Analysis (FBA) was developed. The framework was originally proposed by Raffelt et al. (Citation2017) in which fixel is referring to an individual fiber population within a voxel, enabling researchers to quantify WM characteristics at the level of individual fiber bundle populations rather than the whole voxel (Dhollander et al., Citation2021). For an in-depth discussion with regard to methodology, applications, challenges and opportunities of FBA, the review paper of Dhollander et al. (Citation2021) can be visited. Colleagues from our research group implemented FBA to study age-related differences in WM fiber-specific metrics and the relationship with age-related changes in bimanual motor skills (Zivari Adab et al., Citation2020). In this study, participants were required to rotate both hands (each hand controlling a dial) simultaneously in order to follow a target trajectory, which was visually presented on a screen. As the left dial represented the participants’ cursor to move vertical on the screen and the right dial represented horizontal movements, participants were required to move each dial with precise speed and direction. Multiple target trajectories were presented which varied in terms of bimanual coordination complexity. With FBA, we identified altered WM microstructure (i.e., lower fiber density, FD), WM macrostructure (i.e., smaller cross-sectional area in fiber bundles, FC), and changes in the combined FD and FC metric (i.e. lower FDC incorporating both the micro- and macrostructural effects) with advancing age. These age-related observations were more pronounced in anterior as compared to posterior brain WM. Interestingly, age-related decrements in bimanual performance were mediated particularly by age-related variations in FDC in the interhemispheric tract connecting bilateral prefrontal cortices, via the genu. More confirming evidence was recently published by Seer et al. (Citation2022). Including a large sample of older adults (n = 84; 60-85 years of age), adopting the same bimanual task as in Zivari Adab et al. (Citation2020), the authors reported that higher FDC values in callosal WM tracts connecting bilateral M1s and higher FD values in callosal WM tracts connecting prefrontal areas, were associated with better performance on a visuomotor (bimanual) tracking task. These findings underscore the contribution of changes in micro- and macrostructural WM of interhemispheric pathways (conveying information between prefrontal as well as motor cortices) to suboptimal bimanual performance with advancing age.
Another interesting approach to unravel the importance of age-related changes in intra- and interhemispheric WM pathways for bimanual motor control was taken by Babaeeghazvini et al. (Citation2019). Resting state functional connectivity (assessed by means of electroencephalography) and structural connectivity (assessed by means of DWI) between ipsi- and contralateral motor-related brain areas (i.e. M1 and PMd) were associated with bimanual performance after a 4-day training program. Although different task variants were used in this study, the bimanual task set-up was the same as the one used by Zivari Adab et al. (Citation2020). A stepwise regression model revealed the importance of both structural and functional interhemispheric connectivity for bimanual performance in younger and older adults. More specifically, lower kurtosis anisotropy values (kurtosis equivalent of FA) between right PMd and left M1 (i.e. heterotopic interhemispheric callosal connection) were associated with better performance on the simplest motor subtask, i.e. when both hands were required to rotate at equal speeds in young adults only. With regards to functional connectivity, more neural (phase) synchronization (i.e. a higher weighted phase-lag index) between both M1s was associated with poorer performance on the more complex task variant in older adults only. When linking structural with functional connectivity, intra-hemispheric neural synchronization between left PMd and M1 correlated significantly with kurtosis anisotropy values of the left M1-right PMd, and left M1-right M1 connections in the older adults only. With the latter phase synchronization between M1s being predictive of poor bimanual performance of the complex task variant (where decoupling between both hands was required) in older adults, the authors suggested that age-related changes in inter-hemispheric microstructural organization may account for bimanual performance declines through deteriorations in neural synchronization. Although future studies including mediation analyses (with functional connectivity measures as the mediating factor between callosal WM and bimanual performance) are needed to reinforce this statement, age-related changes in callosal WM at the level of premotor cortex and M1, may be accountable for a decrease in neural phase synchronization possibly responsible for poor bimanual performance.
Along the same lines, Fujiyama et al. (Citation2016) revealed unique interactions between intra- and interhemispheric structural WM and neurophysiological function (assessed by means of TMS), ultimately accounting for bimanual skill performance in the elderly. The visuomotor task set-up was similar to the one used in Zivari Adab et al. (Citation2020), however, target lines were presented in a way that both hands were required 1) to rotate at equal speed (isofrequency (simpler) task variant; 1:1) or 2) to decouple in which one hand had to move three times faster than the other hand (non-isofrequency (more complex) task variants; 3:1 and 1:3 in which the left and right hand were the fastest rotating hand, respectively). Through applying an advanced WM tractography approach (i.e., constrained spherical deconvolution (Tournier et al., Citation2007)), FA values of motor-related interhemispheric pathways, i.e. WM tracts from dorsolateral prefrontal cortex (DLPFC), PMd and M1 to contralateral M1, were obtained. Seeds were determined using an atlas-based approach and selected because of their known involvement in complex bimanual movements. In older adults, lower FA values in all WM pathways seeded from the left hemisphere and terminating in right M1 (i.e. left DLPFC-right M1; left PMd-right M1; left M1-right M1) were associated with poorer performance (i.e. higher target error scores) in the 3:1 task variant. No associations between WM characteristics and bimanual performance were observed in young adults. This was the first study to reveal that characteristics of heterotopic callosal WM tracts, that is between non-M1 and M1 regions, are relevant to perform a complex bimanual task. To assess if these associations between callosal WM structure and bimanual performance were mediated by interhemispheric function, TMS was applied. Interhemispheric function was determined during bimanual movement preparation, as preparatory processes are often affected in the aging population. Interestingly, the association between bimanual performance and FA of the callosal pathways connecting left DLPFC and right M1 disappeared after controlling for interhemispheric function, whereas the association between with FA values of left PMd-right M1 fibers remained significant. In other words, age-related declines in the functional regulation of interhemispheric inhibition between the left DLPFC and contralateral M1, i.e. reduced ability of the left DLPFC to disinhibit the right M1, while preparing a bimanual movement were related to changes in underlying WM microstructure (i.e. lower FA values in interhemispheric WM tracts connecting these heterotopic brain areas), likely accounting for poorer bimanual control.
Finally, Zivari Adab et al. (Citation2018) conducted a study to investigate the relationship between the microstructural organization of WM pathways involving M1 and PMd, and stages of learning the same bimanual visuomotor task as described earlier, however, different task variants (i.e. frequency ratios) were used. The authors utilized DWI and probabilistic constrained spherical deconvolution based tractography to reconstruct WM pathways between the left and right M1s (M1-M1), left PMd and right M1 (LPMd-RM1), and right PMd and left M1 (RPMd-LM1) in both younger and older adults. Consistent with previous findings, the authors observed a decrease in microstructural organization, as reflected by lower FA values, in all reconstructed interhemispheric pathways due to aging. Additionally, higher FA values between bilateral M1s were predictive of greater performance gains during the fast-learning stage (pretest), while higher FA values in the heterotopic pathway from the right PMd to the left M1 were predictive of greater performance gains during the slow-learning stage (pre versus posttest), regardless of age.
Taken together, the literature investigating the role of age-related callosal WM deterioration in bimanual motor control has made great progress over the years. By applying advanced diffusion analyses methods such as FBA, more specific WM details can be obtained, including fiber density and fiber cross-section. Based on the reported findings, it appears that particularly fiber density alone and/or in combination with fiber cross-section contributes to the observed age-related difference in bimanual coordination performance (Seer et al., Citation2022; Zivari Adab et al., Citation2020). Importantly, based on a functionally-informed callosal segmentation, WM metrics of interhemispheric prefrontal and motor pathways significantly contribute to bimanual motor performance. Important to note is that a complex visuomotor bimanual coordination task was adopted in these studies, requiring some degree of cognitive control, particularly in the older population. Also, these studies provided critical new information by demonstrating that not only homotopic interhemispheric tracts (i.e. connecting homologues cortices), but also heterotopic tracts (i.e. connecting non-homologues cortices; here between PMd and M1) significantly contribute to bimanual performance (Babaeeghazvini et al., Citation2019; Fujiyama et al., Citation2016; Zivari Adab et al., Citation2018). Finally, we wish to emphasize the importance and usefulness of multi-modal neuroimaging research. Such studies have enormous scientific value as the interplay between callosal WM microstructure, interhemispheric function and bimanual behavior is investigated providing important new knowledge on the importance of WM fiber bundles for human motor behavior.
Training-Related Callosal Plasticity
With regard to training-related callosal plasticity and bimanual coordination, musicians are often used as a model for use-dependent plasticity as they are experts in bimanual control. In line with studies in non-experts (e.g. Fling et al., Citation2013; Fling & Seidler, Citation2012; Wahl et al., Citation2007), a structure-function relationship was reported in a group of musicians (Vollmann et al., Citation2014). Based on voxel-wise maps, callosal WM was assessed as one large structure. A clear association was observed between interhemispheric inhibition (assessed through TMS as a measure of functional connectivity) and FA in an area of the CC likely including WM pathways projecting to the bilateral hand knobs (i.e., inferior posterior midbody). Interestingly, while this relationship was evident across musicians and non-musicians, it was not generalizable to all musical training regimes and types of instruments played (e.g., string versus piano players), suggesting that use-dependent plasticity and the link between structural and functional connectivity is training-regime and instrument specific (Vollmann et al., Citation2014). Although this association hints toward an important role of the inferior posterior midbody segment of the CC for bimanual coordination, a direct association with bimanual performance was not investigated.
In other studies adopting a relatively short bimanual training regime, no significant changes in callosal WM were reported (e.g. Irmen et al., Citation2020). Irmen and colleagues adopted a virtual reality endoscopic bimanual training over a period of five days and revealed an increase in FA of WM in the vicinity of the ventral premotor areas only. No changes in callosal WM were observed. Importantly, relative to the work on musicians having over six years of musical practice (Vollmann et al., Citation2014), here the authors investigated short-term WM changes induced by bimanual motor training. It is likely that WM changes may not yet have occurred in this large WM structure over this short period of time. Also, by adopting FA as the main outcome measure, it is possible that changes in for example myelination, axonal diameter and density did indeed occur, but were not picked up by this summary measure which does not reveal such biological processes. Therefore, we argue that longitudinal bimanual training regimes including WM measurements pre-, during and post-training are needed to investigate whether and which particular divisions of the CC are particularly involved in this specific motor skill.
Looking Back and Forward
The present review aimed at providing an update on the existing literature regarding the role of the CC in bimanual coordination. Driven by the rapidly evolving neuroimaging techniques and analyses models, emphasis was placed on the importance of functionally-relevant callosal segmentation schemes and the inclusion of WM metrics beyond FA in the search for structure-function interactions between CC substructures and bimanual function (see ). In other words, especially in the healthy (aging) population, i.e. not in clinical work, we noticed a shift from purely establishing an association between callosal WM and bimanual function, toward understanding the underlying mechanisms driving this structure-function relationship. Studies that have already applied such advanced methods revealed (a) an important role of anterior (non-motor) as well as motor (sometimes body part specific) related callosal WM tracts for bimanual coordination performance (including homotopic and heterotopic connections)(Babaeeghazvini et al., Citation2019; Barbeau et al., Citation2015; Fujiyama et al., Citation2016; Gooijers et al., Citation2021; Hawe et al., Citation2013; Hollund et al., Citation2018; Lazari et al., Citation2021; Lench et al., Citation2020; Martino et al., Citation2019; Robert et al., Citation2021; Seer et al., Citation2022; Varghese et al., Citation2022; Zivari Adab et al., Citation2020), (b) that particularly fiber bundle density and cross-section of interhemispheric WM tracts contributed to this association (Barbeau et al., Citation2015; Gooijers et al., Citation2021; Hawe et al., Citation2013; Seer et al., Citation2022; Zivari Adab et al., Citation2020), and (c) that investigating structural interhemispheric connectivity in combination with functional connectivity (by means of EEG, fMRI and/or TMS) appeared particularly useful as the interplay between callosal WM microstructure and interhemispheric function allows us to better understand through which functional mechanisms structural WM contributes to bimanual performance (Babaeeghazvini et al., Citation2019; Bortoletto et al., Citation2021; Fujiyama et al., Citation2016; Wahl et al., Citation2016). Thus, considering the healthy (aging) literature, since 2014 a more coherent fMRI-based approach for CC partitioning has been applied (suggestion III), advanced diffusion acquisition techniques and modeling approaches have been applied to move beyond the traditional WM metrics thereby contributing to a better understanding of the biological underpinnings of this particular structure-function relationship (suggestion IV), and lastly neurophysiological and neuroimaging metrics have been combined to assess the interplay between WM microstructure, brain function and bimanual motor behavior (suggestion V). Despite this progress, a continuation of this shift is necessary especially in clinical populations to further refine our understanding of the specific relationship between callosal WM bundles and bimanual motor control.
FIGURE 3. Involvement of CC subparts and WM metrics in bimanual performance. This conceptual figure depicts that the majority of the works published on the association between the CC and bimanual performance since 2014 emphasizes the particular importance of the midbody including secondary and primary motor areas, followed by the anterior and then the posterior CC sections (indicated by the density of the hatching reflecting the number of papers reporting a significant association). Additionally, a relationship with bimanual motor behavior based on fractional anisotropy, a measure that is predominantly extracted from CC WM, is biologically difficult to interpret as it is sensitive to a wide variation of tissue properties such as myelination, axon diameter, fiber bundle cross-section, fiber orientation, and vascular morphology (Lazari et al., Citation2021). A suggestion is made to study more biologically relevant WM metrics when associating with behavior.
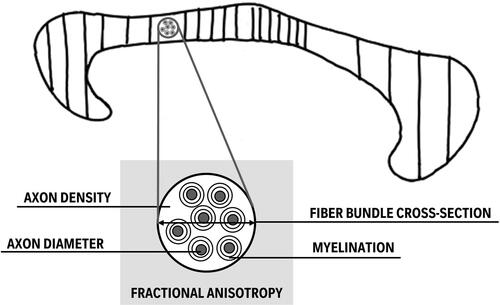
Disclosure Statement
No potential conflict of interest was reported by the authors.
Additional information
Funding
References
- Aboitiz, F., Scheibel, A. B., Fisher, R. S., & Zaidel, E. (1992). Fiber composition of the human corpus callosum. Brain Research, 598(1–2), 143–153. https://doi.org/10.1016/0006-8993(92)90178-c
- Assaf, Y., Alexander, D. C., Jones, D. K., Bizzi, A., Behrens, T. E. J., Clark, C. A., Cohen, Y., Dyrby, T. B., Huppi, P. S., Knoesche, T. R., Lebihan, D., Parker, G. J. M., Poupon, C., Anaby, D., Anwander, A., Bar, L., Barazany, D., Blumenfeld-Katzir, T., De-Santis, S., … Zhou, F.-L. (2013). The connect project: Combining macro- and micro-structure. NeuroImage, 80, 273–282. https://doi.org/10.1016/j.neuroimage.2013.05.055
- Assaf, Y., & Basser, P. J. (2005). Composite hindered and restricted model of diffusion (charmed) mr imaging of the human brain. NeuroImage, 27(1), 48–58. https://doi.org/10.1016/j.neuroimage.2005.03.042
- Babaeeghazvini, P., Rueda-Delgado, L. M., Zivari Adab, H., Gooijers, J., Swinnen, S., & Daffertshofer, A. (2019). A combined diffusion-weighted and electroencephalography study on age-related differences in connectivity in the motor network during bimanual performance. Human Brain Mapping, 40(6), 1799–1813. https://doi.org/10.1002/hbm.24491
- Barbeau, E. B., Lewis, J. D., Doyon, J., Benali, H., Zeffiro, T. A., & Mottron, L. (2015). A greater involvement of posterior brain areas in interhemispheric transfer in autism: Fmri, dwi and behavioral evidences. NeuroImage. Clinical, 8, 267–280. https://doi.org/10.1016/j.nicl.2015.04.019
- Beaulieu, C. (2009). The biological basis of diffusion anisotropy. In H. Johansen-Berg & E. J. Behrens (Eds.), Diffusion MRI: From quantitative measurement to in vivo neuroanatomy. (pp. 105–126) Academic Press. https://doi.org/10.1016/B978-0-12-374709-9.X0001-6
- Bonzano, L., Tacchino, A., Brichetto, G., Roccatagliata, L., Dessypris, A., Feraco, P., Lopes De Carvalho, M. L., Battaglia, M. A., Mancardi, G. L., & Bove, M. (2014). Upper limb motor rehabilitation impacts white matter microstructure in multiple sclerosis. NeuroImage, 90, 107–116. https://doi.org/10.1016/j.neuroimage.2013.12.025
- Bortoletto, M., Bonzano, L., Zazio, A., Ferrari, C., Pedullà, L., Gasparotti, R., Miniussi, C., & Bove, M. (2021). Asymmetric transcallosal conduction delay leads to finer bimanual coordination. Brain Stimulation, 14(2), 379–388. https://doi.org/10.1016/j.brs.2021.02.002
- De Santis, S., Jones, D. K., & Roebroeck, A. (2016). Including diffusion time dependence in the extra-axonal space improves in vivo estimates of axonal diameter and density in human white matter. NeuroImage, 130, 91–103. https://doi.org/10.1016/j.neuroimage.2016.01.047
- Dhollander, T., Clemente, A., Singh, M., Boonstra, F., Civier, O., Duque, J. D., Egorova, N., Enticott, P., Fuelscher, I., Gajamange, S., Genc, S., Gottlieb, E., Hyde, C., Imms, P., Kelly, C., Kirkovski, M., Kolbe, S., Liang, X., Malhotra, A., … Caeyenberghs, K. (2021). Fixel-based analysis of diffusion mri: Methods, applications, challenges and opportunities. NeuroImage, 241, 118417. https://doi.org/10.1016/j.neuroimage.2021.118417
- Domin, M., & Lotze, M. (2019). Parcellation of motor cortex-associated regions in the human corpus callosum on the basis of human connectome project data. Brain Structure & Function, 224(4), 1447–1455. https://doi.org/10.1007/s00429-019-01849-1
- Fling, B. W., Benson, B. L., & Seidler, R. D. (2013). Transcallosal sensorimotor fiber tract structure-function relationships. Human Brain Mapping, 34(2), 384–395. https://doi.org/10.1002/hbm.21437
- Fling, B. W., & Seidler, R. D. (2012). Fundamental differences in callosal structure, neurophysiologic function, and bimanual control in young and older adults. Cerebral Cortex (New York, N.Y. : 1991), 22(11), 2643–2652. https://doi.org/10.1093/cercor/bhr349
- Fujiyama, H., Van Soom, J., Rens, G., Gooijers, J., Leunissen, I., Levin, O., & Swinnen, S. P. (2016). Age-related changes in frontal network structural and functional connectivity in relation to bimanual movement control. The Journal of Neuroscience : The Official Journal of the Society for Neuroscience, 36(6), 1808–1822. https://doi.org/10.1523/JNEUROSCI.3355-15.2016
- Gooijers, J., De Luca, A., Zivari Adab, H., Leemans, A., Roebroeck, A., & Swinnen, S. P. (2021). Indices of callosal axonal density and radius from diffusion mri relate to upper and lower limb motor performance. NeuroImage, 241, 118433. https://doi.org/10.1016/j.neuroimage.2021.118433
- Gooijers, J., & Swinnen, S. P. (2014). Interactions between brain structure and behavior: The corpus callosum and bimanual coordination. Neuroscience and Biobehavioral Reviews, 43, 1–19. https://doi.org/10.1016/j.neubiorev.2014.03.008
- Hawe, R. L., Sukal-Moulton, T., & Dewald, J. P. (2013). The effect of injury timing on white matter changes in the corpus callosum following unilateral brain injury. NeuroImage. Clinical, 3, 115–122. https://doi.org/10.1016/j.nicl.2013.08.002
- Hofer, S., & Frahm, J. (2006). Topography of the human corpus callosum revisited–comprehensive fiber tractography using diffusion tensor magnetic resonance imaging. NeuroImage, 32(3), 989–994. https://doi.org/10.1016/j.neuroimage.2006.05.044
- Hollund, I. M. H., Olsen, A., Skranes, J., Brubakk, A. M., Håberg, A. K., Eikenes, L., & Evensen, K. A. I. (2018). White matter alterations and their associations with motor function in young adults born preterm with very low birth weight. NeuroImage. Clinical, 17, 241–250. https://doi.org/10.1016/j.nicl.2017.10.006
- Hung, Y. C., Robert, M. T., Friel, K. M., & Gordon, A. M. (2019). Relationship between integrity of the corpus callosum and bimanual coordination in children with unilateral spastic cerebral palsy. Frontiers in Human Neuroscience, 13, 334. https://doi.org/10.3389/fnhum.2019.00334
- Irmen, F., Karabanov, A. N., Bögemann, S. A., Andersen, K. W., Madsen, K. H., Bisgaard, T., Dyrby, T. B., & Siebner, H. R. (2020). Functional and structural plasticity co-express in a left premotor region during early bimanual skill learning. Frontiers in Human Neuroscience, 14, 310. https://doi.org/10.3389/fnhum.2020.00310
- Jeurissen, B., Leemans, A., Tournier, J. D., Jones, D. K., & Sijbers, J. (2013). Investigating the prevalence of complex fiber configurations in white matter tissue with diffusion magnetic resonance imaging. Human Brain Mapping, 34(11), 2747–2766. https://doi.org/10.1002/hbm.22099
- Jones, D. K., Knösche, T. R., & Turner, R. (2013). White matter integrity, fiber count, and other fallacies: The do’s and don’ts of diffusion mri. NeuroImage, 73, 239–254. https://doi.org/10.1016/j.neuroimage.2012.06.081
- Lazari, A., Salvan, P., Cottaar, M., Papp, D., Jens van der Werf, O., Johnstone, A., Sanders, Z. B., Sampaio-Baptista, C., Eichert, N., Miyamoto, K., Winkler, A., Callaghan, M. F., Nichols, T. E., Stagg, C. J., Rushworth, M. F. S., Verhagen, L., & Johansen-Berg, H. (2021). Reassessing associations between white matter and behaviour with multimodal microstructural imaging. Cortex; a Journal Devoted to the Study of the Nervous System and Behavior, 145, 187–200. https://doi.org/10.1016/j.cortex.2021.08.017
- Lee, B. Y., Zhu, X. H., Li, X., & Chen, W. (2019). High-resolution imaging of distinct human corpus callosum microstructure and topography of structural connectivity to cortices at high field. Brain Structure & Function, 224(2), 949–960. https://doi.org/10.1007/s00429-018-1804-0
- Lench, D. H., Hutchinson, S., Woodbury, M. L., & Hanlon, C. A. (2020). Kinematic measures of bimanual performance are associated with callosum white matter change in people with chronic stroke. Archives of Rehabilitation Research and Clinical Translation, 2(4), 100075. https://doi.org/10.1016/j.arrct.2020.100075
- Martino, D., Delorme, C., Pelosin, E., Hartmann, A., Worbe, Y., & Avanzino, L. (2017). Abnormal lateralization of fine motor actions in tourette syndrome persists into adulthood. PloS One, 12(7), e0180812. https://doi.org/10.1371/journal.pone.0180812
- Martino, D., Hartmann, A., Pelosin, E., Lagravinese, G., Delorme, C., Worbe, Y., & Avanzino, L. (2019). Motor timing in tourette syndrome: The effect of movement lateralization and bimanual coordination. Frontiers in Neurology, 10, 385. https://doi.org/10.3389/fneur.2019.00385
- Radwan, A. M., Sunaert, S., Schilling, K., Descoteaux, M., Landman, B. A., Vandenbulcke, M., Theys, T., Dupont, P., & Emsell, L. (2022). An atlas of white matter anatomy, its variability, and reproducibility based on constrained spherical deconvolution of diffusion mri. NeuroImage, 254, 119029. https://doi.org/10.1016/j.neuroimage.2022.119029
- Raffelt, D. A., Tournier, J. D., Smith, R. E., Vaughan, D. N., Jackson, G., Ridgway, G. R., & Connelly, A. (2017). Investigating white matter fibre density and morphology using fixel-based analysis. NeuroImage, 144(Pt A), 58–73. https://doi.org/10.1016/j.neuroimage.2016.09.029
- Robert, M. T., Gutterman, J., Ferre, C. L., Chin, K., Brandao, M. B., Gordon, A. M., & Friel, K. (2021). Corpus callosum integrity relates to improvement of upper-extremity function following intensive rehabilitation in children with unilateral spastic cerebral palsy. Neurorehabilitation and Neural Repair, 35(6), 534–544. https://doi.org/10.1177/15459683211011220
- Seer, C., Adab, H. Z., Sidlauskaite, J., Dhollander, T., Chalavi, S., Gooijers, J., Sunaert, S., & Swinnen, S. P. (2022). Bridging cognition and action: Executive functioning mediates the relationship between white matter fiber density and complex motor abilities in older adults. Aging, 14(18), 7263–7281. https://doi.org/10.18632/aging.204237
- Swinnen, S. P. (2002). Intermanual coordination: From behavioural principles to neural-network interactions. Nature Reviews. Neuroscience, 3(5), 348–359. https://doi.org/10.1038/nrn807
- Tournier, J. D., Calamante, F., & Connelly, A. (2007). Robust determination of the fibre orientation distribution in diffusion mri: Non-negativity constrained super-resolved spherical deconvolution. NeuroImage, 35(4), 1459–1472. https://doi.org/10.1016/j.neuroimage.2007.02.016
- Tournier, J. D., Calamante, F., Gadian, D. G., & Connelly, A. (2004). Direct estimation of the fiber orientation density function from diffusion-weighted mri data using spherical deconvolution. NeuroImage, 23(3), 1176–1185. https://doi.org/10.1016/j.neuroimage.2004.07.037
- Tuch, D. S., Reese, T. G., Wiegell, M. R., Makris, N., Belliveau, J. W., & Wedeen, V. J. (2002). High angular resolution diffusion imaging reveals intravoxel white matter fiber heterogeneity. Magnetic Resonance in Medicine, 48(4), 577–582. https://doi.org/10.1002/mrm.10268
- Van Essen, D., & Ugurbil, K. (2013). Human connectome project (1U54MH091657-01) [Data Set]. WU-Minn Consortium.
- Van Essen, D. C., Smith, S. M., Barch, D. M., Behrens, T. E., Yacoub, E., Ugurbil, K., & Consortium, W. U.-M H, WU-Minn HCP Consortium (2013). The wu-minn human connectome project: An overview. NeuroImage, 80, 62–79. https://doi.org/10.1016/j.neuroimage.2013.05.041
- Varghese, R., Chang, B., Kim, B., Liew, S. L., Schweighofer, N., & Winstein, C. J. (2022). Corpus callosal microstructure predicts bimanual motor performance in chronic stroke survivors: A preliminary cross-sectional study. Topics in Stroke Rehabilitation, 1–9. https://doi.org/10.1080/10749357.2022.2095085
- Vollmann, H., Ragert, P., Conde, V., Villringer, A., Classen, J., Witte, O. W., & Steele, C. J. (2014). Instrument specific use-dependent plasticity shapes the anatomical properties of the corpus callosum: A comparison between musicians and non-musicians. Frontiers in Behavioral Neuroscience, 8, 245. https://doi.org/10.3389/fnbeh.2014.00245
- Wahl, M., Lauterbach-Soon, B., Hattingen, E., Hübers, A., & Ziemann, U. (2016). Callosal anatomical and effective connectivity between primary motor cortices predicts visually cued bimanual temporal coordination performance. Brain Structure & Function, 221(7), 3427–3443. https://doi.org/10.1007/s00429-015-1110-z
- Wahl, M., Lauterbach-Soon, B., Hattingen, E., Jung, P., Singer, O., Volz, S., Klein, J. C., Steinmetz, H., & Ziemann, U. (2007). Human motor corpus callosum: Topography, somatotopy, and link between microstructure and function. The Journal of Neuroscience : The Official Journal of the Society for Neuroscience, 27(45), 12132–12138. https://doi.org/10.1523/JNEUROSCI.2320-07.2007
- Weinstein, M., Green, D., Geva, R., Schertz, M., Fattal-Valevski, A., Artzi, M., Myers, V., Shiran, S., Gordon, A. M., Gross-Tsur, V., & Bashat, D. B. (2014). Interhemispheric and intrahemispheric connectivity and manual skills in children with unilateral cerebral palsy. Brain Structure & Function, 219(3), 1025–1040. https://doi.org/10.1007/s00429-013-0551-5
- Weinstein, M., Myers, V., Green, D., Schertz, M., Shiran, S. I., Geva, R., Artzi, M., Gordon, A. M., Fattal-Valevski, A., & Ben Bashat, D. (2015). Brain plasticity following intensive bimanual therapy in children with hemiparesis: Preliminary evidence. Neural Plasticity, 2015, 798481. https://doi.org/10.1155/2015/798481
- Witelson, S. F. (1989). Hand and sex differences in the isthmus and genu of the human corpus callosum. A postmortem morphological study. Brain, 112(Pt(3), 799–835. https://doi.org/10.1093/brain/112.3.799
- Zhang, H., Schneider, T., Wheeler-Kingshott, C. A., & Alexander, D. C. (2012). Noddi: Practical in vivo neurite orientation dispersion and density imaging of the human brain. NeuroImage, 61(4), 1000–1016. https://doi.org/10.1016/j.neuroimage.2012.03.072
- Zivari Adab, H., Chalavi, S., Beets, I. A. M., Gooijers, J., Leunissen, I., Cheval, B., Collier, Q., Sijbers, J., Jeurissen, B., Swinnen, S. P., & Boisgontier, M. P. (2018). White matter microstructural organisation of interhemispheric pathways predicts different stages of bimanual coordination learning in young and older adults. The European Journal of Neuroscience, 47(5), 446–459. https://doi.org/10.1111/ejn.13841
- Zivari Adab, H., Chalavi, S., Monteiro, T. S., Gooijers, J., Dhollander, T., Mantini, D., & Swinnen, S. P. (2020). Fiber-specific variations in anterior transcallosal white matter structure contribute to age-related differences in motor performance. NeuroImage, 209, 116530. https://doi.org/10.1016/j.neuroimage.2020.116530