Abstract
In order to examine the anodic polarization characteristics of typical structural materials of boiling water reactors (BWRs), the anodic polarization curves of type 316L stainless steel (316L SS) and Alloy 182 were measured in deaerated high purity water at 553 K using the previously reported measurement method which was confirmed suitable for high temperature – high purity water. In order to specify which constituent element determines the dissolution characteristics of these materials, the anodic polarization curves of pure iron, pure nickel, and pure chromium were also surveyed. The anodic polarization curve of 316L SS was determined to have active, passive, and transpassive states which were the same as type 304 SS (304 SS) showed. But, Alloy 182 had different polarization characteristics especially near the corrosion potential as it had no active state. From comparison results of the polarization characteristics of these materials and their constituent elements, the corrosion characteristics of these materials were concluded to be mainly determined by the corrosion characteristics of chromium.
1. Introduction
Anodic polarization curves are widely used for the fundamental evaluation of various corrosion analyses, and they are also used for elucidation of the corrosion mechanism of materials. For example, stress corrosion cracking (SCC) is propagated because the anodic current originates with the metal ionization at a crack tip as a counter reaction to the cathodic current generated by the reduction reaction on the surface of the material. Therefore, measuring anodic polarization curves is applicable to elucidation of the mechanism of several corrosion problems such as SCC by evaluating the dissolution behavior at the surface and crack tip. However, most electrochemical measurement methods in high temperature – high purity water are difficult because high purity water has high electric resistivity. Accordingly, only limited data have been reported for polarization curves under simulated BWR conditions. In order to measure polarization curves in high temperature – high purity water, we previously developed the measurement method which was suitable for high temperature – high purity water [1].
The anodic polarization curves of 304 SS in high temperature – high purity water have been measured by several groups [2–6]. But, the polarization characteristics of recent typical BWR structural materials such as 316L SS and Alloy 182 in high temperature – high purity water have not been studied. And how material classification affects anodic polarization curves in the BWR environment is still unclear. Therefore, it is necessary to clarify the effects that different materials exert on these curves.
Our purpose in this work was to examine the effects of different materials on anodic polarization curves measured in high temperature – high purity water. We measured anodic polarization curves of 316L SS and Alloy 182 which are typical materials used in current BWRs and compared them with curves of 304 SS [1]. In addition, in order to investigate which element determines the corrosion characteristics of those steels and alloys, the anodic polarization curves of iron (Fe), chromium (Cr), and nickel (Ni) were measured as typical constituent elements of the materials. Furthermore, information is limited about the response to various water chemistries for the anodic polarization curves of typical structural materials in high purity water. Hence, we investigated the effect of sulfuric acid as a typical corrosive reagent in BWR coolant in order to promote elucidation of such phenomena as SCC, based on electrochemical information.
2. Experimental procedures
2.1. Test equipment
We used same autoclave connected to the high temperature water loop as previously reported [7]. The potentiostat (Princeton Applied Research, model 283) which has a built-in high input impedance (1013Ω) electrometer was used for measurement of polarization curves.
Before all anodic polarization curve measurements, electrochemical impedance spectroscopy (EIS) measurement was made to estimate the contribution of solution resistance (R sol) to the controlled electrode potential between a working electrode (WE) and reference electrode (RE) at 553 K. In the EIS measurement, a frequency response analyzer (FRA: NF Corporation, model 5095) was connected with the potentiostat.
Monitoring of the electrochemical corrosion potentials (ECPs) of the WE was started after the test water temperature reached 553 K. The ECPs were continuously measured using an electrometer (Keithley, model 6514) for up to 96 h until the ECP reached a stable value at 553 K. The anodic polarization curves were measured after confirming the ECPs remained at a constant value.
2.2. Test electrodes
The shapes and the sizes of each WE are shown in . The chemical compositions of the commercial 316L SS and Alloy 182 are shown in . A Pt wire (WE #1) was used as an inert WE for the EIS measurement. The WEs #4 to #6 were made of commercially available pure metal materials. The purities of Fe, Cr, and Ni were 99.5% (Nilaco, FE-221387), 99.9% (Nilaco, CR-093558), and 99.9% (Nilaco, NI-311385), respectively. All WEs were individually loaded into the autoclave with respect to each measurement.
Table 1. Shapes and sizes of WEs.
Table 2. Chemical compositions (wt%) of WEs.
The WEs of 316L SS, Fe and Ni doubled as the lead wire (ϕ0.5 mm). The WEs of Alloy 182 and Cr were machined into a rectangular solid shape (0.5 × 0.5 × 20 mm) by electric discharge machining from block material. In particular, the block material of Alloy 182 was obtained from a weld joint which was formed by shielded metal arc welding. The surface area of each WE which was in contact with the test water was set at 0.1 cm2. The surface of the machined WEs was polished by emery paper to remove the surface layer formed by machining. The lead wires which were made of Alloy 600 were attached by spot welding only if the machined WEs were employed. All the WEs and the counter electrode (CE) were polished to #2000, and were treated by ultrasonic cleaning in acetone for 5 min, and then in ultrapure water for 5 min. The lead wires and the surface of the WEs except for the exposed region were covered with a heat shrinkable polytetrafluoroethylene (PTFE) tube for electrical insulation.
A zirconia membrane type Ag/Ag2O ECP sensor [7] was used as internal RE. A platinum plate (20 × 10 × t1 mm) with platinum lead wire (ϕ0.5 mm) was used for the CE. The electrode potential of the RE was calibrated based on Pt electrode potential in water with 35 ppb H2 in order to control the electrode potential of the WE based on the SHE scale. The electrode potential of the hydrogen electrode reaction on Pt was employed as the criterion for compensating the electrode potential of the RE. The redox potential of Equation (1) was calculated as −0.496 Vvs.SHE at 35 ppb H2 in 553 K high-purity water using the Nernst equation.
2.3. Test conditions and measurement parameters
Temperature of test water was controlled at 553±1 K and pressure was set at 8.0±0.1 MPa in order to avoid boiling. The test water was circulated at a rate of 1 dm3 · h−1 through the autoclave. Thus, the flow condition in the autoclave was semi-stagnant. Deaerated ultrapure water was employed as test water for measuring anodic polarization curves of 316L SS, Alloy 182, Fe, Cr, and Ni. Deaerated sulfuric acid (H2SO4) aqueous solution was injected into the test water at the inlet of the autoclave using an injection pump when the effect of SO4 2− was studied.
The anodic polarization curves were measured using the previously-developed method [1]. The potential step method was employed, where the electrode potential was held for 1000 s at every 10 mV. Since the narrow potential step interval was used, the present potential step method is close to the linear potential scan method with a scan rate of 0.01 mV · s−1.
The EIS measurement was performed in the same environment as the anodic polarization curve measurements. The sine wave voltage with amplitude of 10 mV overlapping the corrosion potential was applied to the WE with the FRA via the potentiostat in the EIS measurement. Based on a preliminary EIS measurement using a 100 kΩ resistance in the atmosphere at room temperature, we found that the system did not show any distortion when the frequency range was from 0.01 to 100 Hz. Hence, in this work, the response currents and phases could be considered only in the frequency range from 0.01 to 100 Hz.
3. Results
3.1. Solution resistance estimation
In order to estimate the potential control error in the electrode system of this work, the solution resistance was evaluated from the EIS data obtained using the Pt electrode (WE #1). The EIS measurement results which were obtained using Equations (2) and (3) are plotted in as the Nyquist diagram.
A capacitive semi-circle was observed. The point at the inter-section of the horizontal axis with the left end of the semi-circle curve is R sol [8]. From the measurement results, the solution resistance for this electrodes alignment was about 11 kΩ · cm2.
The maximum current density of the anodic polarization curve of 304 SS was estimated at about 10 μA cm−2 [1]. Since the potential control error E err which arises from the IR drop in the solution between the WE and RE was calculated by Equation (4), E err in the electrode system in this experiment was estimated at 0.11 V (= 10 μA cm−2 × 11 kΩ · cm2).
Thus, the potential control accuracy was not significantly affected by the E err, when the current density was less than about 10 μA · cm−2. Therefore, we did not compensate for the IR drop, and only the region below 10 μA · cm−2 is discussed in this work.
3.2. Anodic polarization curves of 316L SS and Alloy 182
3.2.1. 316L SS
shows the anodic polarization curves of 316L SS and 304 SS [1] which were measured in deaerated high temperature – high purity water. The curves indicated 316L SS and 304 SS had the same corrosion characteristics qualitatively. The active, passive, and transpassive states were clearly observed on the polarization curve of 316L SS. The general corrosion rate of 316L SS was lower than that of 304 SS, because the current density of 316L SS was half that of 304 SS below 0.2 Vvs.SHE. But with the current density at potentials higher than 0.2 Vvs.SHE, both 316L SS and 304 SS were in close agreement quantitatively.
Figure 2. Measured anodic polarization curves of type 316L SS, Alloy 182 and type 304 SS [1] in deaerated high temperature – high purity water.
![Figure 2. Measured anodic polarization curves of type 316L SS, Alloy 182 and type 304 SS [1] in deaerated high temperature – high purity water.](/cms/asset/55a701b4-f278-4a3c-bb69-857e04dfc566/tnst_a_649077_o_f0002g.gif)
From this result, characteristic parameters of the anodic polarization curve of 316L SS were estimated () and compared with those reported for 304 SS [1]. The overall behaviors of both anodic polarization curves were rather similar qualitatively. The passivation potential (Epp) of 316L SS was equivalent to that of 304 SS. But, the critical current of passivation (icrit) and the passive current density (ips) were half those of 304 SS. Consequently, passivity was reached more easily and stably for 316L SS compared with 304 SS. And, since the transpassivation potential (E tp) of 316L SS was higher than that of 304 SS by about 0.15 V, the passive state could be kept through a wider potential region. Accordingly, we estimated that 316L SS had good corrosion characteristics compared with 304 SS regarding general corrosion.
Table 3. Characteristic parameters of anodic polarization curves of typical structural material of BWRs.
3.2.2. Alloy 182
also shows the measured polarization curve of Alloy 182. The passive and transpassive states were clearly observed on this curve. Spontaneous passivation without active dissolution was observed on this anodic polarization curve as well, indicating that the corrosion characteristics of Alloy 182 differed significantly from 304 SS and 316L SS at low potential region around − 0.4 Vvs.SHE. Since Alloy 182 did not have an active state, the passive state was observed for a wider potential region compared with 304 SS and 316L SS, which was also seen from E pp and E tp values listed in . Accordingly, we estimated that the general corrosion characteristics of Alloy 182 for the low potential region around − 0.4 Vvs.SHE were better than those of 304 SS and 316L SS. However, the transpassive state was observed just as it was for 304 SS and 316L SS in the high potential region above − 0.2 Vvs.SHE. Accordingly, we estimated that the corrosion characteristics of Alloy 182 were the same as those of 304 SS and 316L SS only above − 0.2 Vvs.SHE.
3.3. Anodic polarization curves of the pure metals
In order to confirm which elements, Fe, Cr, or Ni, determined the anodic characteristics of Fe- and Ni-base corrosion-resistant alloys, we measured the anodic polarization curves of the pure metals. Those measurement results are shown in . The polarization curves of each pure metal had complicated characteristics like those of a passive metal. Some undulations which were likely due to the transition of dependence of oxide film characteristics on potential [9] were observed for each pure metal. In the figure, the ECP range of the BWR from the hydrogen water chemistry (HWC) condition (ca.-0.5 Vvs.SHE) to the normal water chemistry (NWC) condition (ca.0.2 Vvs.SHE) is also illustrated. Current densities of pure metals were high and ordered as: Fe ca.10 μA · cm−2, Ni ca. 1.5 μA · cm−2 and Cr ca. 0.3 μA · cm−2 at low potential less than − 0.10 Vvs.SHE. Chromium was electrochemically inert compared with both Fe and Ni in the ECP region below − 0.10 Vvs.SHE. On the other hand, all pure metals showed high current density of approximately 6 to 11 μA · cm−2 at potentials above − 0.10 Vvs.SHE. In high temperature – high purity water, Cr had distinctive corrosion characteristics that changed two orders of magnitude bordering on − 0.10 V vs. SHE.
Figure 3. Measured anodic polarization curves of Fe, Cr, and Ni in deaerated high temperature – high purity water with thermodynamically stable species of Fe, Cr, and Ni.
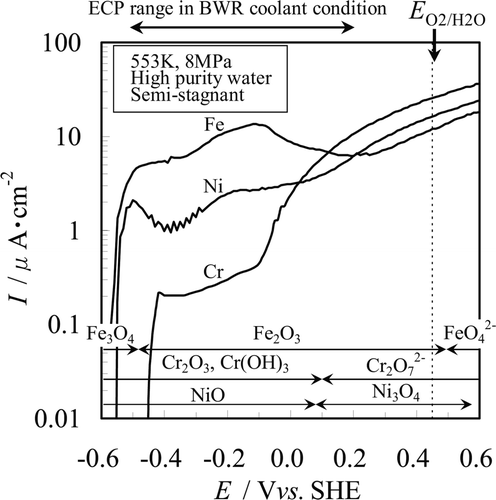
3.3.1. Iron
The anodic current density of Fe increased rapidly to 14 μA · cm−2 as potential was gained from corrosion potential E corr (ca. − 0.56 Vvs.SHE) and it had an active dissolution peak at around − 0.11 Vvs.SHE. Then the current density decreased to 6.2 μA · cm−2, and a trough was observed around 0.20 Vvs.SHE which seemed to correspond to a passive state. The current density increased as potential was gained once again in the region higher than ca.0.25 Vvs.SHE. Accordingly, we found that the current density (equal to general corrosion rate) of Fe remained high around 6 to 14 μA · cm−2 over the potential range examined ca. − 0.5 to 0.6 Vvs.SHE.
3.3.2. Chromium
The E corr of Cr was − 0.45 Vvs.SHE, and this value was 0.11 V higher than that of both Fe and Ni. The current density of Cr had a significantly low value around 0.2 to 0.4 μA · cm−2 in the low potential region from − 0.45 to − 0.10 Vvs.SHE, and the active dissolution peak was not observed. Though the current density of Cr in the low potential region showed the tendency to rise slowly with increasing potential, we concluded that the passivation occurred. On the other hand, the current density increased rapidly as potential increased in the region above −0.10 Vvs.SHE, indicating that transpassive dissolution occurred in the same manner as 304 SS, 316L SS and Alloy 182.
Accordingly, the corrosion behavior of Cr was divided into two contrasting characteristics. Corrosion rate of Cr was low due to passivation in the potential region lower than − 0.10 Vvs.SHE. However, it became high because of the acceleration of the dissolution reaction shown in Equation (5) above − 0.10 V vs. SHE.
3.3.3. Nickel
The E corr of Ni was − 0.55 Vvs.SHE which was near the E corr of Fe. A small active dissolution peak was observed at ca. − 0.50 Vvs.SHE. And, current density was decreased as potential increased. A trough like that seen for the passive state of 304 SS and 316L SS was observed at − 0.38 Vvs.SHE on the anodic polarization curve of Ni, and the current density rose again with a further increase in potential like the polarization curves of both 304 SS and 316L SS.
3.4. Effect of sulfuric acid addition
(a)–(c) shows the polarization curves of 304 SS, 316L SS and Alloy 182 measured in 1 ppm sulfuric acid aqueous solution which simulated the environment inside a crevice in a structural material such as a crack tip of SCC. Since H2SO4 was used to maintain the concentration of SO4 2−, only Equations (6) and (7) were taken into consideration. By the addition of 1 ppm sulfuric acid, the solution pH was estimated to decrease slightly from 5.6 to 4.9.
Figure 4. Effects of sulfuric acid on anodic polarization curves of typical BWR structural materials.
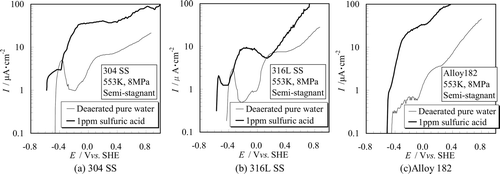
The polarization curves acquired in pure water are plotted in the same figure as reference data. The passive state remained only on the polarization curves of both SSs in the H2SO4 condition whereas the current density of Alloy 182 increased monotonically by H2SO4 addition. The passive state was observed for 304 SS and 316L SS at the potential region of − 0.42 to −0.38 Vvs.SHE and − 0.47 to − 0.41 Vvs.SHE, respectively. Increasing behaviors of the current density were caused by acceleration of the metal dissolution shown by Equation (8). Although Alloy 182 had a wide passivity region in high purity water, it had low resistance against sulfuric acid compared with the SSs.
We saw from these figures that anodic current increased when H2SO4 was present, whereby ECP should shift to the more negative direction. As a result, although ECP is the most important index for corrosive environment estimation, it would not be suitable to evaluate the corrosive environment only using ECPs. To assess the corrosive environment, proper water chemistry control techniques and management are needed.
4. Discussion
4.1. Diagnostic characteristics for the polarization curves of pure metals
The meanings of each current peak for the pure metals are discussed later based on features of each polarization curve previously shown in . The measured polarization curves of pure metals are compared with the potential range where stable chemical species exist, which was obtained from the potential-pH diagrams [10] of Fe-H2O, Cr-H2O and Ni-H2O systems at 573 K and pH = 5.6. In addition, the upper limit potential of the “potential window” of water (E O2/H2O) is represented by the dotted line parallel to the vertical axis in .
4.1.1. The polarization behavior of pure Fe
The stable chemical species of Fe are Fe3O4 and Fe2O3 in the potential region of the BWR environment. An oxide film which consists of Fe3O4 and/or Fe2O3 is formed on the Fe surface according to Equations (9)–(12) depending on the potential.
Dissolution rate of Fe is mitigated in the region where iron oxide (for example Fe2O3) can exist stably depending on the potential-pH diagram at 573K [10]. It has been reported that 99% of the dissolved metal elements remained at the surface as an oxide film according to immersion test results (temperature, 561 K; flow velocity, 1 cm · s−1; period, 1000 h) for carbon steel [11]. Consequently, almost all Fe ionized according to Equation (9) remains at the surface as Fe3O4 or Fe2O3, and these species govern the shape of the anodic polarization curve of Fe in the following manner.
1. | Anodic current of metal dissolution (Equation (9)) is decreased by Fe3O4 or Fe2O3 covering the bare surface. | ||||
2. | Anodic current is increased by oxidation of Fe3O4 to Fe2O3 (Equation (12)). |
The reason for the current density rising in the potential region from − 0.5 to − 0.1 Vvs.SHE is presumed due to factor (ii) being significant. If the protective ability of the oxide film of Fe3O4 and Fe2O3 is not high enough, the dissolution reaction (Equation (9)) continues to occur in this region. The reason for the current density falling in the potential region from − 0.1 to 0.3 Vvs.SHE is presumed due to factor (i) being significant. It seems reasonable that once a thicker Fe2O3 film with high chemical stability is formed, the dissolution reaction is inhibited. The current density rise from the oxygen generation reaction described by Equation (13) is overlapped in the polarization curve of Fe only above ca. 0.4 V vs. SHE.
4.1.2. The polarization behavior of pure Cr
The stable chemical species of Cr in the potential region found in the BWR environment are Cr2O3, Cr(OH)3 and Cr2O7 2−. Both Cr2O3 and Cr(OH)3 are formed on the Cr surface in the potential region below − 0.10 Vvs.SHE according to Equations (5) and (14)–(16).
Therefore, the reason why the current density was low below − 0.1 Vvs.SHE is that Cr2O3 and Cr(OH)3 have good protection characteristics against dissolution of base metal. On the other hand, it seems to be the transpassive dissolution that causes the rapid increase of current density above − 0.10 Vvs.SHE. Since there are no stable solid phase species for the high potential region greater than about 0.1 Vvs.SHE, Cr dissolves as Cr2O7 2− according to Equation (5).
As Sugimoto [12] reported the Cr fraction on the surface of Fe–Cr alloy was formed as passive film. Tanno and Yashiro [13] also analyzed the depth profile of Cr in the oxide film formed on 304 SS in 561 K high purity water for 90 h. Their results indicated that Cr concentration decreased when 304 SS was immersed in water including 200 ppb dissolved oxygen, but it increased in water including less than 5 ppb dissolved oxygen.
Those results can be explained thermodynamically; Cr content decreased in the high oxygen concentration condition (high potential) because Cr is dissolved easily as Cr2O7 2− in the transpassive state, on the other hand, Cr content increased in low oxygen concentration condition (low potential) because hardly any Cr dissolves in the passive state. This agrees with our present results.
As mentioned above, the effect of Cr addition to an alloy on corrosion characteristics illustrates that the corrosion rate will be decelerated only below − 0.1 Vvs.SHE. On the other hand, the corrosion rate will be accelerated above − 0.1 Vvs.SHE.
4.1.3. The polarization behavior of pure Ni
The stable chemical species of Ni in the potential region found in the BWR environment are NiO and Ni3O4. At potentials from −0.55 to 0.08 Vvs.SHE, NiO is formed on the surface of Ni according to Equation (18). Ni3O4 is formed above 0.08 Vvs.SHE according to Equation (19). Ni can exist as stable solid phase species over an extensive potential region in water. It can be presumed that the anodic polarization curve of Ni was determined by the current rise from the reactions shown below and the characteristics of NiO and Ni3O4 such as protection against dissolution of base metal, electric conductivity and solubility.
The polarization curve of Ni has complicated behavior including more undulations than Fe and Cr have (). It is known that the anodic oxidation of Ni proceeds in two stages due to formation of higher order oxide of Ni [14] such as Ni3O4. The start potential for a gradual increase in anodic current, about 0.0 V, is close to the equilibrium potential of NiO/Ni3O4.
4.2. The influence of constituent elements of SSs and Alloy 182
The meanings of each peak which were observed in the anodic polarization curves of both SSs and Alloy 182 are examined based on the anodic polarization curves of Fe, Cr, and Ni as typical constituents of structural materials.
4.2.1. 316L SS
shows the anodic polarization curves of Fe, Cr, and Ni compared to the anodic polarization curve of 316L SS. Stable chemical species of Fe, Cr, and Ni for each potential region are summarized in . The following points can be understood with respect to each potential region by comparing and . Discussion about a secondary passive state was not made here because the influence of IR drop is not negligible for high current density close to 10μA · cm−2.
Figure 5. Governing elements of anodic polarization curve of 316L SS for (a) active state, (b) passive state, and (c) transpassive state.
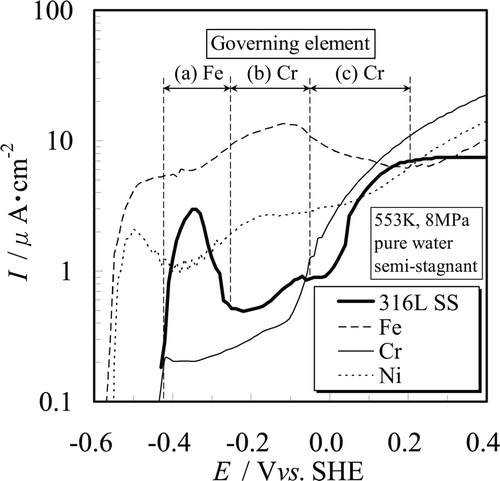
Table 4. The stable chemical species of Fe, Cr, and Ni in each region of both SSs.
(a) Active state. The active dissolution peaks are observed around − 0.4 Vvs.SHE in the case of both SSs. This potential is found in the Cr2O3 and Cr(OH)3 stable region, and also in the NiO stable region. Therefore, both Cr and Ni are in the passive state at − 0.4 Vvs.SHE. Although Fe is in the Fe2O3 stable region, the potential − 0.4 Vvs.SHE is near the equilibrium potential of Fe3O4/Fe2O3. And, the peak current density of SSs at the active state is about half of the current density of Fe at the corresponding potential. We conclude that the active state of both SSs is characterized by Fe dissolution current (Equation (9)) overlapped with the Fe2O3 formation current (Equation (12)).
(b) Passive state. Although the current density of Fe increases with the potential rise to − 0.10 Vvs.SHE, the passive state is observed from ca. − 0.3 to 0 Vvs.SHE for 316L SS. The current density of 316L SS is one order of magnitude lower than that of Fe and is rather close to that of Cr. Therefore, Cr2O3 and Cr(OH)3 which formed on the surface according to Equations (15) and (16) can suppress dissolution of base metal, and consequently the current densities on this potential region can be kept at a low level. From this we conclude that Cr plays an important role in determining corrosion characteristics of the SSs even in high temperature – high purity water.
(c) Transpassive state. The current densities increase with increasing potential from 0 to 0.20 Vvs.SHE for 316L SS. The start potential of transpassivity E tp and the slope of current densities vs. potential in this potential region of both SSs are in good agreement with the behavior of Cr. Accordingly, the transpassive state is caused by the transpassive dissolution of Cr (Equation (5)).
From these results, we see that the electrochemical characteristics of both SSs in high temperature – high purity water are characterized mainly by the dissolution properties of Cr and Fe. The role of Ni regarding any effect on the electrochemical characteristics of both SSs is thought to be less important than that of the other two elements. Therefore, the anodic polarization curves of both SSs change significantly around − 0.1 Vvs.SHE which is the transition potential from the passive to the transpassive state of Cr.
Hosokawa and Nagase [15] reported the fractions of Fe, Cr, Ni in the oxide film which was formed on the surface of 304 SS after 1000 h in 553 K high purity water. Their results indicated that the oxide film consisted of two layers. The outer layer was of Fe-rich oxides, and the inner layer was of Cr-rich oxides, even under the NWC condition (high ECP) or the HWC condition (low ECP). And, Ni had the lowest fraction in both layers under both conditions. Consequently, Fe and Cr played essential roles in the anodic polarization properties of both SSs. The results of Hosokawa and Nagase are in close agreement with our results derived from anodic polarization curve measurements.
In conclusion, the anodic polarization curves of both 304 SS and 316L SS can be defined based on the dissolution property of Cr, and the property of Fe (Equations(9) and (12)) overlaps the dissolution curve only in the active state. In the passive and transpassive states, corrosion behavior is strongly governed by the polarization property of Cr.
It might be generally accepted that the corrosion behaviors of the SSs are mainly determined by the polarization property of Cr. However, the current density of 316L SS is about one half that of 304 SS at the active and passive states even though Cr concentration of 316L SS (16.88 wt%) is 1.3 wt% lower than that in 304 SS (18.18 wt% [1]). The 316L SS contains 2.13 wt% molybdenum (Mo), and this may account for the observed decrease in the current density of 316L SS in the active and passive states.
It is known that the current density of a SS in the active and passive states is decreased by the addition of a small amount of Mo [16] into SS base metal. The polarization curves of Fe-18Cr-xMo alloys (0 ≦ x ≦ 8 wt%) measured in 1 kmol · m−3 H2SO4 aqueous solution at room temperature showed that the i crit of Fe-18Cr-2Mo alloy was 1/10 lower than that of Fe-18Cr (0 wt%Mo) alloy [17]. Similarly, the polarization curves of Fe-18Cr-xMo alloys (0 ≦ x ≦ 10 wt%) measured in 1 kmol · m-3 HCl aqueous solution at room temperature also indicated that the current density of Fe-18Cr-2Mo alloy was one-tenth to one-quarter that of Fe-18Cr (0 wt%Mo) alloy [18].
As for the effect of Cr concentration, according to the measured polarization curves of Fe-xCr alloys (0 ≦ x ≦ 100 wt%) in 0.1 kmol · m−3 Na2SO4 aqueous solution at room temperature, the i crit and the i ps of Fe-20Cr alloy were almost the same values (ca. 10 A · m−2, ca. 10−2 A · m−2) as those of Fe-15Cr alloy [19]. Hence, we can assume that the polarization characteristics of SSs containing 18.18 and 16.88 wt% Cr do not differ significantly with each other.
It has also been reported that the current density of 316L SS is approximately one half the value of 304L SS in the active–passive transition region in 0.5 kmol · m−3 Na2SO4 aqueous solution at 523 K [20]. The effect of the addition of Mo on the anodic dissolution of SSs in high temperature aqueous systems is greater than that of the Cr concentration. A detailed discussion on the effect of Mo in conjunction with the effect of pH deserves further study.
4.2.2. Alloy 182
compares the anodic polarization curves of Fe, Cr, and Ni to that of Alloy 182. The stable chemical species of Fe, Cr, and Ni in each potential region are summarized in . The anodic polarization curve of Alloy 182 is consistent with the behavior of Cr qualitatively in that no active state is observed. This is due to the suppression of the Fe dissolution (Equation (9)) and Fe2O3 formation (Equation (12)) currents. Since the Fe concentration of Alloy 182 is 1/7 times lower (11 wt%) compared to 304 SS and 316L SS, it seems that the peak originating from dissolution and oxidation of Fe does not emerge on the anodic polarization curve of Alloy 182. The following points are seen with respect to each potential region by comparing and .
Figure 6. Governing elements of anodic polarization curve of Alloy 182 for (a) passive state and (b) transpassive state.
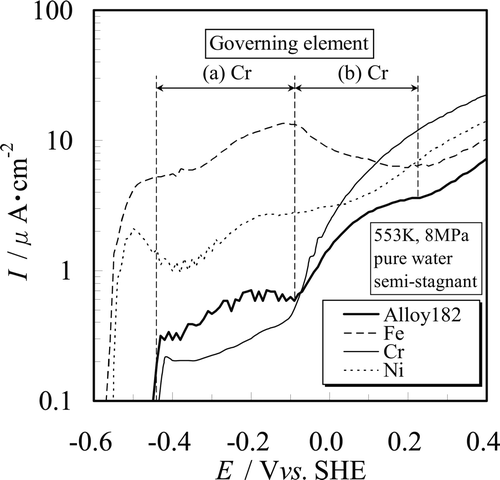
Table 5. The stable chemical species of Fe, Cr, and Ni in each region of Alloy 182.
(a) Passive state. The passive state of Alloy 182 observed in the potential range from − 0.41 to − 0.09 Vvs.SHE is in accord with the passive state of Cr within ±0.01 V. However, i ps of Alloy 182 is from 0.3 to 0.7 μA · cm−2 which is two times higher than that of Cr. Therefore, it seems that the passive state of Alloy 182 mainly consists of the i ps of Cr which overlaps the current of the NiO formation reaction as shown in Equation (18).
(b) Transpassive state. The current density increasing behavior is observed due to transpassive dissolution in the potential range from − 0.09 to about 0.24 Vvs.SHE. The E tp (− 0.09 Vvs.SHE) and the current density increasing behavior are in good agreement with those of Cr. Therefore, the transpassive state of Alloy 182 also originates with the dissolution reaction of Cr to Cr2O7 2− as shown in Equation (5) as well as for both SSs.
The results show that the anodic polarization curve of Alloy 182 is dominated by Cr more prominently than the curves of both SSs because the Fe concentration of Alloy 182 is lower than that in both SSs.
Our present study indicates that the corrosion characteristics of 304 SS, 316L SS, and Alloy 182 as typical structural materials of BWRs are mainly determined by the polarization property of Cr particularly at the passive and transpassive states. And, the corrosion characteristics are also affected by the property of Fe only for 304 SS and 316L SS in the active state. The polarization behavior of Alloy 182 completely differs from that of both SSs especially at low potential around − 0.5 Vvs.SHE. Furthermore, the current density increment of Alloy 182 is higher than for both SSs in the experiment when 1ppm H2SO4 was present. This indicates that the corrosion rate of Alloy 182 in H2SO4 aqueous solution is higher than that of both SSs. Corrosion characteristics of Alloy 182 cannot be equated to those of SSs. It is necessary to evaluate the various corrosion phenomena according to the material of interest. Furthermore, in order to control and manage various corrosion problems of 304 SS, 316L SS, and Alloy 182 in BWRs, the potential should be controlled within the range where transpassive dissolution of Cr cannot occur.
5. Conclusion
In order to determine fundamental polarization characteristics of typical structural materials of BWRs, the anodic polarization curves of 316L SS and Alloy 182 were measured in 556 K high purity water which simulated BWR conditions. The conclusions are summarized below.
1. | The anodic polarization curve of 316L SS was similar to that of 304 SS. But, the anodic polarization curve of Alloy 182 was not similar to those of the SSs, especially in the low potential region near the corrosion potential. The corrosion characteristics of Alloy 182 cannot be equated with those of SSs. The various corrosion phenomena should be evaluated for the material of interest. | ||||
2. | The anodic polarization curves of Fe, Cr, and Ni were measured to estimate which of the elements mainly determined the polarization characteristics of the structural materials. Results suggested that the corrosion characteristics of the typical structural materials in the BWR environment were mainly determined by the polarization property of Cr. | ||||
3. | The effect of sulfuric acid as a typical corrosive species was also determined. Alloy 182 had a high corrosion rate in the presence of sulfuric acid compared with both 304 SS and 316L SS. The anodic current increased when H2SO4 was present, whereby ECP should shift to the more negative direction. It is not suitable to evaluate the corrosive environment only using ECPs of structural materials of BWRs. To assess the corrosive environment, proper water chemistry control techniques and management are needed. |
References
- Tachibana , M. , Ishida , K. and Wada , Y. 2009 . Study of polarization curve measurement method for type 304 stainless steel in BWR high temperature-high purity water . J. Nucl. Sci. Technol , 46 : 132 – 141 .
- Hirayama , H. , Yamashina , T. and Nakamura , S. 1985 . SCC susceptibility and oxide film characteristics of type 304 stainless steel under controlled potential in high-temperature-high-purity water . Boshoku Gijutsu , 34 : 86 – 91 . [in Japanese]
- Hishida , M. , Takabayashi , J. and Kawakubo , T. 1985 . Polarization curve measurement in high purity water at elevated temperatures . Corrosion , 41 : 570 – 574 .
- Kim , Y.J. 2001 . Role of hydrogen peroxide and its decomposition in BWRs . Proceedings 10th international conference on environmental degradation of materials in nuclear power systems-water reactors, NACE . August 5–9 2001 . [CD-ROM]
- Yeh , T.K. 2005 . The impact of oxygen and hydrogen recombination efficiency on the effectiveness of NMCA in reducing the corrosion potential in boiling water reactors . Proceedings 12th international symposium on environmental degradation of materials in nuclear power systems – water reactor, TMS . August 15–18 2005 . pp. 715 – 726 .
- Uchida , S. , Morishima , Y. and Hirose , T. 2007 . Effects of hydrogen peroxide on corrosion of stainless steel (VI)—effects of hydrogen peroxide and oxygen on anodic polarization properties of stainless steel in high temperature pure water . J. Nucl. Sci. Technol , 44 : 758 – 766 .
- Sakai , M. , Takahashi , T. and Watanabe , R. 2001 . Durability of electrical insulation and water-seal properties of the ECP sensor junctures used under BWR water chemistry conditions with neutron/gamma ray irradiation . Proceedings 10th international symposium on environmental degradation of materials in nuclear power system-water reactor, NACE . August 5–9 2001 . [CD-ROM]
- Itagaki , M. Denkikagaku impedance-hou / genri · sokutei · kaiseki (Electrochemical Impedance Method / fundamental · measurement · analysis) 83 Maruzen , , Tokyo ISBN 978–4–621–08000–9 C3043 (2008) [in Japanese]
- Pourbaix , M. 1974 . Atlas of electrochemical equilibria in aqueous solutions , 2nd English ed. , Houston , TX : National Association of Corrosion Engineers .
- Chen , C.M. , Akai , K. and Theus , G.J. 1983 . Computer calculated potential pH diagrams to 300°C Vol. 2: Handbook of diagrams The Babcock & Wilcox Company, Ohio 44601 Electric Power Research Institute-Report, NP–3137
- Honda , T. , Kashimura , E. and Ohashi , K. 1987 . Corrosion of ferrous materials and deposition of trace metal ions in high temperature and high purity water . Boshoku Gijutsu , 36 : 267 – 274 . [in Japanese]
- Sugimoto , K. 2008 . Passive films on stainless steels — present state of analysis and understanding . Zairyo-to-Kankyo , 57 : 375 – 384 . [in Japanese]
- Tanno , K. and Yashiro , H. 1986 . Corrosion of stainless steel in high temperature water and its fundamental chemistry . Hyomen , 24 : 535 – 552 . [in Japanese]
- Sato , N. Denkyoku Kagaku Vol. 2 (Electrode Chemistry Vol. 2), Nittetsu Engineering Information Center 389 Tokyo ISBN 4-930825-06-7 C3043 (1993) [in Japanese]
- Hosokawa , H. and Nagase , M. 2004 . Investigation of cobalt deposition behavior with zinc injection on stainless steel under BWR conditions . J. Nucl. Sci. Technol , 41 : 682 – 689 .
- Cihal , V. 1984 . Intergranular Corrosion of Steels and Alloys , New York : Elsevier Science Publishing Co., Inc .
- Rockel , M.B. 1973 . The effect of molybdenum on the corrosion behavior of iron-chromium alloys . Corrosion , 29 : 393 – 396 .
- Sugimoto , K. 2009 . Kinzoku Fushoku-Kogaku , Tokyo : Uchida-Rokakuho . [in Japanese]
- Hara , N. and Sugimoto , K. Metal Databook , 3rd ed. , 372 Maruzen , , Tokyo : The Japan Institute of Metals ed . ISBN 4-621-03825-7 (1993) [in Japanese]
- Sugimoto , K. , Soma , S. and Hosoya , K. Kouon-kouatsu suiyouekichuu-ni okeru austenite sutainnless kou-no anode bunkyoku kyokusen (The anodic polarization curves of the austenite stainless steel in high temperature – high pressure aqueous solutions) . Proceedings of Spring Technical Meeting ’81, Japan Society of Corrosion Engineering, A-110 . pp. 34 – 37 . [in Japanese]