Abstract
A group of Monte Carlo simulations has been performed for external neutron dosimetry calculation based on a whole-body mathematical model. The Chinese mathematical phantom (CMP) is a mathematical human body model developed based on methods of ORNL (Oak ridge National Laboratory) mathematical phantom series (OMPS), and data from Chinese reference man and reference Asian man. Fluence-to-absorbed dose conversion coefficients of 24 organs and tissues for monoenergetic neutron beams ranging from 10−9 to 102 MeV were calculated using the Monte Carlo code MCNPX. Irradiation conditions include anterior–posterior, posterior–anterior, right lateral, left lateral, rotational, and isotropic geometries. Results for the different organs are compared with those recommended in International Commission of Radiological Protection (ICRP) publication 74 and results obtained based on the visible Chinese human (VCH) phantom. Overall the consistency among the three sets of data was observed, but significant deviations up to 30–50% were also found in the AP, PA and lateral irradiation conditions. Since CMP represents the Chinese population, this work is helpful as a reference to investigate the difference of the neutron induced organ doses due to the anatomical variation between the Chinese and the Caucasians, and that between the average population and an individual.
1. Introduction
One of the important tasks in radiation protection is to estimate the radiation exposure in practice and ensure the dose below established limits. Since in vivo measurements of organ doses are not possible, the necessity to derive dose quantities from measurable quantities arises. Conversion coefficients, relating ‘operational quantities’ which can be measured at workplaces to ‘protection quantities’ [Citation1], i.e. the absorbed dose in body, are introduced for this purpose by the International Commission of Radiological Protection (ICRP).
Conversion coefficients are determined numerically with the help of computational anthropomorphic models. The reference values for radiation protection provided by the ICRP publication 74 [Citation2] were calculated with mathematical phantoms, which is called ‘MIRD-type’, where the body and the organs or the tissues are composed of simply geometrical shapes [Citation3,Citation4]. With the quick development of computers and medical imaging techniques, researchers have made considerable efforts to develop voxel-based anatomical models based on computer tomography (CT), magnetic resonance imaging (MRI), or color images of individuals for radiation dosimetry [Citation5–Citation14]. More than 30 voxel-based models have been established till now [Citation15], a few of which are based on Chinese specimen, such as VCH phantom and CNMAN [Citation16,Citation17]. These phantoms give better representation for human body compared to the mathematical phantoms since they are made from real anatomical data and consist of millions of small volume elements. However, because of deriving from individual data, usually voxel-based models do not represent the physical characteristic of an average population. On the contrary, the mathematical phantoms based on the reference man data are able to represent the characteristic of an average population. Due to the racial representativeness and fast calculation of the mathematical phantoms, even though the voxel-based phantoms have made a great development and ICRP has formally adopted voxel-based computational phantoms derived from CT image data due to the improved anatomical realism, the mathematical phantoms are still widely used in health physics and medical physics [Citation18–Citation24].
Neutron radiation exposures are very important at nuclear power generation facilities, high-energy electron accelerators, proton and heavy ion accelerators and deep space explorations, where the energy spectra can range from the thermal region to above GeV levels.Dose conversion coefficients for neutron are widely used for the radiation protection at these facilities. Some dosimetric data for low-energy spectrum were derived based on mathematical phantoms representing Caucasians [Citation25,Citation26]. Neutron dose conversion coefficients derived from the MIRD (Medical Internal Radiation Dose Committee)-based simplified model family have been summarized by a joint task group of the ICRP and the International Commission on Radiation Units and Measurements (ICRU), and further have been documented in ICRP Publication 74 [Citation2]. Recently, a study based on the visible Chinese human (VCH) phantom (height 166 cm and 54 kg), a voxel phantom developed from high resolution cryosectional color photographic images of a healthy Chinese adult male cadaver, had been carried out [Citation27]. The absorbed doses in organs were also calculated for idealized irradiation geometries using the Japanese Voxel Phantoms JM (height 171 cm, weight 65 kg) and JF (152 cm, 44 kg) in the energy range from 10−9 to 150 MeV, and compared with the data of ICRP Publication 74 and the VCH phantom [Citation28].
This work is part of a systematic study for radiation protection for the Chinese population with the Chinese mathematical phantom (CMP), a mathematical human body model developed based on methods of Oak ridge National Laboratory (ORNL) mathematical phantom series, and data from Chinese reference and man reference Asian man [Citation23]. As the anatomical parameters of the Chinese people are different from those of the Caucasians to some extent, it is necessary to obtain the conversion coefficients based on the models which represent the characteristics of the Chinese population. While Chinese voxel-based phantoms only represent individual characteristics and lack flexibility for organ adjustment, CMP represents well for the average Chinese population. Besides, mathematical phantoms require relatively short simulation time. The construction of CMP and the results of the conversion coefficients for external photon irradiation based on CMP have been published [Citation23,Citation24]. It would be valuable to update CMP to comply with the ICRP 103 Publication [Citation29], which is the latest ICRP recommendation. However, so far this work has not been carried out due to the lack of the Chinese reference man data for the organs/tissues such as extrathoracic(ET) region, oral mucosa and lymphatic nodes.
In this work, a new set of conversion coefficients from fluence to absorbed dose are presented for monoenergetic neutron beams from 10−9 to 102 MeV under six different irradiation geometries based on CMP and Monte Carlo code MCNPX. The calculated organ doses are compared under different irradiation geometries and incident neutron energies. Up to now the latest conversion coefficients for some external and internal exposures are shown in graphs in ICRP 110 Publication [Citation30]. These coefficients were obtained with the ICRP reference phantoms, which are voxel phantoms. In this work, the calculated neutron dose conversion coefficients based on CMP are not compared with the results in ICRP 110 Publication as the numerical values have not been distributed yet. Therefore, the results based on CMP are compared with data provided by ICRP publication 74 [Citation2] and results based on VCH phantom [Citation16]. Data provided by ICRP publication 74 were based on the mathematical phantom representing the Caucasians which are generally taller than Chinese in height and heavier in weight, while the latter is a voxel phantom based on a Chinese individual human who is thinner than average. The comparison between the results based on CMP and ICRP74 is expected to reflect the differences between races, while the comparison between the results based on CMP and VCH is expected to reflect the differences between the individual and the average and the differences between the mathematical phantom and the voxel phantom.
2. Materials and methods
2.1. Chinese mathematical phantom
The CMP includes two independent adult Chinese models, male and female, which represent characteristics of Chinese man and woman respectively [Citation23]. The shape equations were developed according to the ONRL mathematical phantom theories. The parameters to describe the organs were mainly taken from the Chinese reference man, which provided a formalized description of some specific physical, chemical, physiological, anatomical, bio-kinetic and other parameters of relevance in radiological protection [Citation31]. Some reference Asian man data were also used as complements due to the incompleteness of the Chinese reference man. In this work, the model of the male adult was adopted in order to compare with the results from other male models. shows the shape of the exterior and internal organs of this model. The body is presented vertically, with the z-axis directed upward towards the head, the x-axis towards the phantom's left (the reader's right) and the y-axis toward the phantom's posterior side. The origin is located at the center of the underside of the trunk section of the phantom. The height and the weight of this model are compared with those of the MIRD phantom which was adopted by ICRP74 and the VCH phantom in .
Table 1. Height and weight of the phantoms compared in this work
2.2. Monte Carlo simulations
The Monte Carlo technique has been widely used in radiological simulations where the physical experiments are either inconvenient or impossible. MCNP is a general-purpose Monte Carlo code designed to transport neutrons, photons, and electrons in arbitrarily assigned three-dimensional geometries. The MCNPX code, used in this work, represents a major extension of the MCNP code, enabling it to track all particles at all energies.
2.3. Dose calculation
The absorbed doses in organs were obtained from MCNPX program as the amount of energy deposited per unit mass. Then the absorbed doses in organs were normalized by incident neutron fluence so as to derive the fluence-to-dose conversion coefficients. Conversion coefficients of 24 different organs and tissues (including a ‘remainder’ composed of the additional organs and tissues), as defined in ICRP publication 60 [Citation1], were calculated.
Six standard configurations of the irradiation source were defined [Citation32,Citation33]. Parallel neutron beams that directed perpendicularly to the longitudinal axis of the body were set for anterior–posterior (AP), posterior–anterior (PA), left-lateral (LLAT) and right-lateral (RLAT) geometries, respectively. A full 360° rotation of neutron beams around the longitudinal axis of the fixed body was set for the rotational (ROT) geometry. Neutron beams projected with no preference in direction, where the particle flux per unit solid angle remained constant, were configured for the isotropic (ISO) geometry. Neutron beams ranging from 10−9 to 102 MeV were simulated.
As the neutron cross section plays important roles in the calculations, shows the neutron cross section library used for calculation in this work. The S(α,β) scattering was considered for the low-energy neutrons (eg. E < 4 eV), and the corresponding cross section data adopted were from those for light water at 300 K in the TMCCS library.
Table 2. The cross-section library of neutron used for calculation in this work.
3. Results and discussion
3.1. Precision of the results
The statistical uncertainties of the simulation results were dependent on neutron energy and direction. The uncertainties are aimed at <5% in order to get reliable data in the simulation. Pre-runs were carried out to investigate the time consumption and statistical uncertainties of the results in each radiation condition. Considering both the statistical error control and time consumption, finally 5 × 106 particles were simulated for AP, PA, LLAT, and RLAT irradiation, 2 × 108 particles for ROT irradiation and 1 × 108 particles for ISO irradiation. Overall, the precision is satisfactory as the statistical error is lower than 5% for nearly 97% of all fluence-to-absorbed dose conversion coefficient data. The data larger than 5% mainly come from the very low energy range of 10−9–10−8 MeV.As an example, shows the results for thyroid, in the unit of pGy cm2, with the statistical error percentages in parenthesis.
Table 3. Fluence-to-dose conversion coefficients (pGy cm2) for thyroid of CMP under various geometries with the statistical error percentages given in parentheses
3.2. Energy dependence
The shapes of the curves showing the fluence-to-absorbed dose conversion coefficients depending on neutron energy are more or less similar for all the organs and tissues, in accordance with the varying predominance of particle interaction types. The data for stomach are shown as an example in . The whole energy range can be divided into three parts approximately. Within the low energy part under 1 eV, most of the direct energy deposition is contributed by the gamma ray during capture of the thermalized neutrons. In particular, the 2.2 MeV photons that are emitted via the 1H(n,γ)2D reaction play a significant role in the deposition of energy. At energy range between 1 eV and 10 keV, the energy deposited by recoil protons from elastic scattering on hydrogen becomes important. Both the photons generated by the neutron capture and the recoil protons from elastic scattering on hydrogen contribute to the energy deposition. The cross section of neutron capture decreases with the increasing neutron energy, but the energy of the recoil protons from elastic scattering on hydrogen increases, the counteraction of the two mechanism leads to the relatively flat curve. At the high energy range above about 1 MeV, the production of charged particles by nuclear reactions such as (n,D), (n,T), etc. becomes dominant for the deposition of energy and sharp increasing curve is observed.
3.3. Comparison with data in ICRP74 and results based on VCH phantom
As mentioned in the introduction part, the results in this work were compared with data in ICRP74 publication and results based on the VCH phantom. Anatomical variation of models mainly comes from the shape and size of each organ and tissue, material composition and the location of the organs and tissues, etc. The differences of the dose conversion coefficients based on the three models mainly come from the different physical characteristic of the races of the Mongolians and Caucasians or the anatomical variation (shapes and sizes of organs and bodies) among models. The use of voxel phantoms can reduce the uncertainties of doses caused by the differences of organ and body shapes compared to real human body. However, body sizes are limited, and not always applied for the whole population. On the contrary, the mathematical phantoms can reduce the uncertainties caused by the differences of body sizes. However, body and organ shapes of the mathematical phantoms are not similar to real human. Results of typical organs under different irradiation geometry are analyzed.
Generally, the calculated neutron fluence-to-absorbed dose conversion coefficients in selected organs display agreement in the results based on CMP, VCH phantom, and ICRP 74 data, especially as far as the energy dependence is concerned. However, the deviations of up to 50% for some organs at certain energy are also observed.
3.3.1. AP and PA irradiation
The fluence-to-absorbed dose conversion coefficients are compared in AP and PA irradiation geometry and the largest discrepancy is found in the data for lung and bladder. Results for lung are compared among all the three models, while those for bladder are compared only between CMP data and the ICRP74 data due to the lack of the published data based on the VCH phantom, as plotted in .
Figure 3. Comparison of fluence-to-absorbed dose conversion coefficients from ICRP 74, the VCH phantom, and CMP for lung and bladder under AP and PA irradiation geometry.
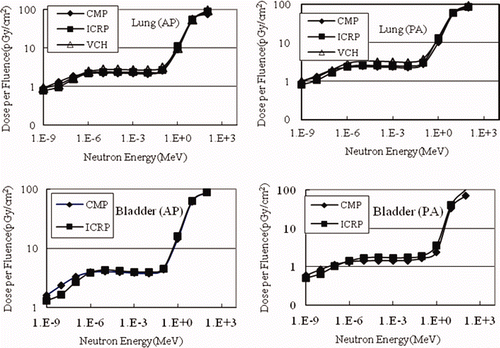
Lung data of CMP are reported 25% lower than that of the VCH phantom under AP irradiation and 40% lower under PA irradiation. Although the weight of the VCH phantom (54 kg) is much lower than CMP (60 kg), the thickness of the thorax in the VCH phantom (18.7 cm) is almost the same with that of CMP (18.7 cm). Therefore, this difference should be attributed to the shape and the position of lung in these two phantoms. The difference of the dose conversion coefficients between the two phantoms in PA irradiation (40%) is larger than that in AP irradiation (25%). It suggests that lung in the VCH phantom may locate in a more posterior position compared to that in CMP. And the fact that lung in CMP is described with equations and the lung in the VCH phantom is obtained from medical image data of a real person also contributes to this difference.
ICRP data for lung are similar with results of CMP under PA irradiation, a bit lower on average under AP irradiation, especially at low energy. A maximum difference of 35% was reported at about 10−8 MeV. It can be explained since lung is located at the back of the thorax, and the larger size of the male MIRD phantom (trunk thickness 20 cm) which was adopted by ICRP compared to CMP (trunk thickness 18.7 cm) leads to better shielding effects to low-energy neutrons. Penetrating ability of neutron increases in accordance with energy, thus deviations become less obvious at high energy range. Similar trend is observed on the data for bladder, except that it locates in the front of the body. The main difference between the CMP data and ICRP data occurs under PA irradiation and 30% higher results of CMP are reported.
3.3.2. RLAT and LLAT irradiation
Under lateral irradiations, a large discrepancy of the fluence-to-absorbed dose conversion coefficients for lung and some asymmetric organs, such as the rectum and the liver, is observed among the CMP, VCH phantom, and ICRP74 data, as shown in . The data for rectum are only compared between the CMP data and ICRP74 data as the VCH data is not available. The data for lung are only compared between the CMP data and VCH data as the ICRP74 data only gives the average value for the LLAT and RLAT irradiations.
Figure 4. Comparison of fluence-to-absorbed dose conversion coefficients from ICRP 74, the VCH phantom, and CMP for rectum and liver under LLAT and RLAT irradiation geometry.
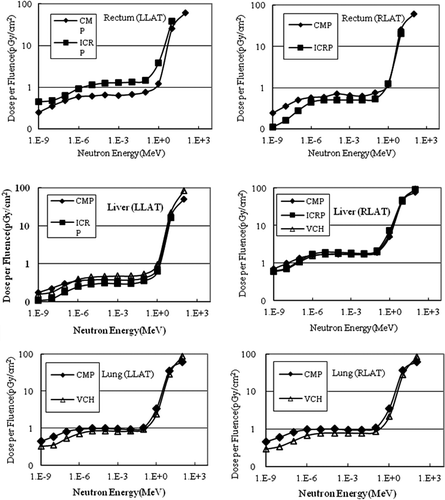
At low and medium neutron energy range, the CMP data is generally lower (about 35%) than the ICRP74 data under LLAT irradiation, and higher (about 20%) than the ICRP74 data under RLAT irradiation for rectum. Results of both phantoms tend to agree with each other in high energy region since neutron penetration ability increases. Rectum is located in the left part inside the trunk. The difference between the dose conversion coefficients for rectum in these two mathematical phantoms indicate that rectum may locate at a more right position in CMP than in the MIRD phantom.
As for the liver, an organ located in the right of the body, results of CMP, VCH phantom, and ICRP74 data is in good agreement under RLAT irradiation. But under LLAT irradiation, the results of VCH phantom are 20% higher on average than those of CMP. And the results of CMP are about 20% higher than ICRP74 data. It can be explained that the trunk size of the Caucasians are generally larger than the Mongolians, and the width of the thorax of CMP (28.5 cm) is larger than the VCH phantom (26.6 cm). On the other hand, the liver in the mathematical phantom was surrounded by a thick layer of soft tissue, which also increases the actual transportation distance of neutrons. But the voxel phantom like VCH constructed from medical image data obtained from real persons, can give a more realistic anatomic description of human body than the mathematical phantoms.
The dose conversion coefficients for lung is interesting as the data of CMP are larger in both the LLAT and RLAT irradiation conditions than those of the VCH phantom. The difference is up to 30%. It could be attributed to the lack of arms in CMP whereas the upper arms in the VCH phantom provide the shielding for the lung inside the trunk.
3.3.3. ROT and ISO irradiation
The ROT and ISO geometries are to some extent the combinations of other four idealized geometries. Generally, the fluence-to-absorbed dose conversion coefficients show smaller differences among the data based on CMP, VCH phantom and ICRP74 under ISO and ROT irradiation. It could be ascribed to the homogenous dose distributions within phantoms resulted by these specific irradiation geometries. The results for two typical widely distributed organs, bone surface and skin, are shown in .
Figure 5. Comparison of fluence-to-absorbed dose conversion coefficients from ICRP 74, the VCH phantom, and CMP for bone surface and skin under ROT and ISO irradiation geometry.
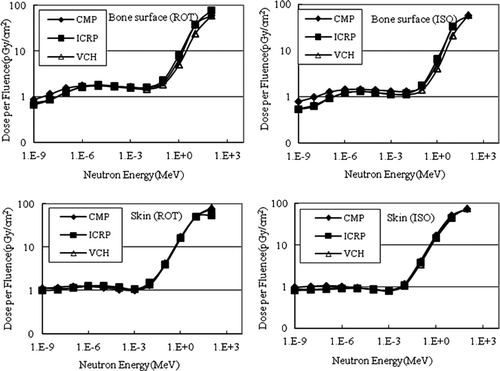
Most prominent deviations appear at energy higher than 100 keV for the bone surface data between the voxel phantom VCH and the two mathematical phantoms. These results are consistent with the previous study [Citation27]. It can be inferred that the more profound embedment between the skeleton and its surrounding medium due to the natural combining style in voxel phantom, especially at positions like the thoracic cage where the abundant intermediate space is present for soft tissue to adhere and get through, provides more protection than that of the mathematical phantoms. Skin dose are almost the same within the three phantoms, which could be ascribed to both the comparatively uniform space distributions of skin and the property of these two specific irradiation geometries that result in rather homogeneous dose distributions within phantoms.
4. Conclusions
Fluence-to-dose conversion coefficients of 24 organs and tissues for external monoenergetic neutron beams from 10−9 to 102 MeV were calculated based on the CMP using Monte Carlo code MCNPX under six standard radiation conditions. Results were compared with the data recommended in ICRP publication 74 and those based on the VCH phantom.
Differences between the CMP and ICRP74 data mainly appear on asymmetric organs for low-energy neutrons such as bladder in the frontal radiation and liver in the lateral radiation. Asymmetric distribution of organs inside the body results in different shielding thickness, which impacts more on the low energy neutrons than the high energy ones. Maximum difference is observed in the data for lung and bladder under AP and PA irradiation and in the data for liver and rectum under lateral irradiation. Results for lung and bladder of CMP are about 30% higher than ICRP74 data under AP and PA irradiation respectively, which could be ascribed to the larger size of the male MIRD phantom (trunk thickness 20 cm) compared to CMP (trunk thickness 18.7 cm). Discrepancy up to 36% and 44% for liver and rectum are observed under LLAT and RLAT irradiation between the CMP data and the ICRP74 data respectively. The difference of the data for liver could be attributed to the larger trunk size of the Caucasians than that of the Mongolians. The difference of the data for rectum indicates that rectum may locate at a more right position in CMP than in the MIRD phantom. Hardly any big discrepancy exists under ISO and ROT irradiation due to their uniformity.
The difference between the data from CMP and the VCH phantom is contributed by the difference of the average anatomical data and an individual, and the difference between the mathematical phantom and the voxel phantom. The data from CMP for lung is up to 40% lower than those from the VCH phantom under PA irradiation. It suggests that lung in the VCH phantom may locate in a more posterior position compared to that in CMP.Discrepancy up to 40% is also observed for the data of liver under LLAT irradiation. It can be explained by both the larger width of the thorax of CMP (28.5 cm) compared to that of the VCH phantom (26.6 cm) and the thick layer of soft tissue surrounded the liver in the mathematical phantom. In contrast to the large dose discrepancy in lateral geometries, smaller differences within 10% were usually observed for most organs in ROT and ISO geometries between CMP and the VCH phantom due to the uniformity in these two specific irradiation geometries.
The CMP well represents the characteristic of the Chinese population. This work based on CMP, and the comparison with the data recommended in ICRP74 and the data based on a Chinese voxel phantom, VCH, is helpful as a reference to investigate the difference of the neutron induced organ doses due to the anatomical variation between the Chinese and the Caucasians, and that between the average population and an individual.
Acknowledgment
This work was supported by the Chinese National Science Foundation Project 11105081 and 11075091.
References
- International Commission on Radiological Protection . 1991 . Recommendations of the International Commission on Radiological Protection , Oxford , , UK : Pergamon Press . ICRP Publication 60
- International Commission on Radiological Protection . 1996 . Conversion Coefficients for Use in Radiological Protection Against External Radiation , Oxford , , UK : Pergamon Press . ICRP Publication 74
- Kramer , R. , Zankl , M. , Williams , G. and Drexler , G. 1982 . The Calculation of Dose from External Photon Exposures Using Reference Human Phantoms and Monte Carlo Calculations,(I) the Male (ADAM) and Female (EVA) Adult Mathematical Phantoms , Neuherberg , , Germany : GSF-National Research Center for Environment and Health . GSF-Bericht S-885
- Snyder , W.S. , Ford , M.R. and Warner , G.G. 1978 . Estimates of Specific Absorbed Fractions for Photon Sources Uniformly Distributed in Various Organs of a Heterogeneous Phantom , New York : Society of Nuclear Medicine . MIRD Pamphlet No. 5 Revised
- Zankl , M. , Veit , R. , Petoussi , N. , Mannweiler , E. , Wittmann , A. and Drexler , G. 1994 . Realistic computerized human phantoms . Adv. Space Res , 14 : 423 – 431 .
- Zubal , I.G. , Harrel , C.R. and Smith , E.0. 1994 . Computerized three-dimensional segmented human anatomy . Med. Phys , 21 : 299 – 302 .
- Jones , D.G. 1997 . A realistic anthropomorphic phantom for calculating organ doses arising from external photon irradiation . Radiat. Prot. Dosim , 72 : 21 – 29 .
- Dimbylow , P.J. 1997 . FDTD calculations of the whole-body averaged SAR in an anatomically realistic voxel model of the human body from 1 MHz to 1 GHz . Phys. Med. Biol , 42 : 479 – 490 .
- Xu , X.G. , Chao , T.C. and Bozkurt , A. 2000 . VIP-Man, an image-based whole-body adult male model constructed from color photographs of the visible human project for multi-particle Monte Carlo calculations . Health Phys , 78 : 476 – 486 .
- Chao , T.C. , Bozkurt , A. and Xu , X.G. 2001 . Organ dose conversil coefficients for 0.1–10 MeV electrons calculated for the VIP-Man tomographic model . Health Phys , 81 : 203 – 221 .
- Saito , K. , Wittmann , A. and Koga , S. 2001 . Construction of a computed tomographic phantom for a Japanese male adult and dose calculation system . Radiat. Environ. Biophys , 40 : 69 – 76 .
- Petoussi-Henss , N. , Zankl , M. and Fill , U. 2002 . The GSF family of voxel phantoms . Phys. Med. Biol , 47 : 89 – 106 .
- Tomoaki , N. , Soichi , W. , Kiyoko , S. , Etsuo , K. , Satoshi , W. , Masao , T. and Yukio , Y. 2004 . Development of realistic high-resolution whole-body voxel models of Japanese adult males and females of average height and weight, and application of models to radio-frequency electromagnetic-field dosimetry . Phys. Med. Biol , 49 : 1 – 15 .
- Lee , C. , Park , S.H. and Lee , J. 2006 . Development of the two Korean adult tomographic computational phantoms for organ dosimetry . Med. Phys , 33 : 380 – 390 .
- Zaidi , H. and Xu , X.G. 2007 . Computational anthropomorphic models of the human anatomy: the path to realistic Monte Carlo modeling in radiological sciences . Annu. Rev. Biomed. Eng , 9 : 1 – 1 .
- Zhang , G.Z. , Luo , Q.M. , Zeng , S.Q. and Liu , Q. 2008 . The development and application of the visible Chinese human model for Monte Carlo dose calculations . Health Phys , 94 : 118 – 125 .
- Zhang , B.Q. , Ma , J.Z. , Liu , L.Y. and Cheng , J.P. 2007 . CNMAN, a Chinese adult male voxel phantom constructed from color photographs of a visible anatomical data set . Radiat. Prot. Dosim , 124 : 130 – 136 .
- Lodwick , J.C. and Spitz , B.H. 2008 . Monte Carlo simulation of an anthropometric phantom used for calibrating in vivo K-XRF spectroscopy measurements of stable lead in bone . Health Phys , 95 : 744 – 753 .
- Dewji , S. , Hertel , N. and Scarboro , S. 2008 . Assessing internal contamination after an RDD event using readily available NaI detectors, The 53rd Annual Meeting of the Health Physics Pittsburgh , PA
- Stratakis , J. , Damilakis , J. and Gourtsoyiannis , N. 2005 . Organ and effective dose conversion coefficients for radiographic examinations of the pediatric skull estimated by Monte Carlo methods . Eur. Radiol , 15 : 1948 – 1958 .
- Krstic , D. and Nikezica , D. 2007 . External exposure of deciduous tooth enamel to photons: dose conversion coefficients for standard radiation fields . Radiat. Environ. Biophys , 46 : 339 – 348 .
- Park , S. , Lee , C. and Lee , J.K. 2007 . Dosimetry calculation for internal electron source using Korean adult stylized phantom . IFMBE Proc , 14 : 2102 – 2105 .
- Qiu , R. , Li , J.L. , Zhang , Z. , Wu , Z. , Zeng , Z. and Fan , J.J. 2008 . Photon SAF calculation based on the Chinese mathematical phantom and comparison with the ORNL phantoms . Health Phys , 95 : 716 – 725 .
- Qiu , R. , Li , J.L. , Zhang , Z. , Liu , L.Y. , Bi , L. and Ren , L. 2009 . Does conversion coefficients based on the Chinese mathematical phantom and MCNP code for external photon irradiation . Radiat. Prot. Dosim , 134 : 3 – 12 .
- Stewart , R.D. , McDonald , J.C. , Tanner , J.E. and Harty , R. 1993 . Calculation of neutron fluence-to-dose conversion factors for extremities . Radiat. Prot. Dosim , 42 : 279 – 286 .
- Mares , V. , Leuthold , G. and Schraube , H. 1997 . Organ doses and dose equivalents for neutrons above 20 MeV . Radiat. Prot. Dosim , 70 : 391 – 400 .
- Zhang , G.Z. , Liu , Q. and Luo , Q.M. 2007 . Monte Carlo simulations for external neutron dosimetry based on the visible Chinese human phantom . Phys. Med. Biol , 52 : 7367 – 7383 .
- Satoh , D. , Sato , K. , Takahashi , F. and Endo , A. 2010 . Monte Carlo simulation using Japanese voxel phantoms to analyze the contribution of particle types and their energy distributions to organ doses upon external neutron exposure . Nucl. Sci. Technol , 47 : 62 – 69 .
- International Commission on Radiological Protection . 2007 . Recommendations of the International Commission on Radiological Protection ICRP Publication 103, Elsevier Press, Oxford, UK
- International Commission on Radiological Protection . 1991 . Recommendations of the International Commission on Radiological Protection ICRP Publication 110, Elsevier Press, Oxford, UK
- Wang , J.X. , Chen , R.S , Zhu , H.D , Zhou , Y.Z and Ma , R.W . 1998 . Data of Anatomical Physiological and Metabolic Characteristics for Chinese Reference Man , Beijing : Atomic Energy Press .
- International Commission on Radiological Protection . 1975 . Task Group on Reference Man , Oxford , , UK : ICRP Publication 23, Pergamon Press .
- International Commission on Radiation Units and Measurements . 1998 . Conversion Coefficients for Use in Radiological Protection Against External Radiation ICRU Report 57, Bethesda , MD, ICRU