Abstract
Neutron nuclear data of 99Tc was evaluated, considering cross-sections and spectra provided from recent experiments. The evaluation was made in the incident neutron energy range from 1 keV to 20 MeV, using the optical model and nuclear reaction models. The optical model calculation based on the coupled-channels method was performed for the interaction of neutrons with 99Tc, and potential parameters appropriately chosen reasonably explain the measured data of total cross-section. The cross-section of inelastic scattering, capture, (n, 2n), (n, p), (n, α) and (n, n′α) reactions, and γ-ray emission spectra were calculated on the basis of statistical model with preequilibrium and direct components, and they were compared with available experimental data. It is found that the presently evaluated cross-sections and γ-ray emission spectra well reproduce those experimental values and that there is a large discrepancy among the present result and evaluated data for neutron emission spectra. The obtained capture cross-section increases at the energies below 1 MeV, relative to that in JENDL-4.0. This makes the transmutation efficiency of 99Tc into stable 100Ru by accelerator driven system enhanced. The production cross-section of 99Mo important for the medical use of nuclear diagnostics reduces by 5–30% at the energies above 12 MeV, compared with JENDL-4.0.
1. Introduction
Technetium-99 is a radioactive fission product with a large cumulative fission yield of about 6% [Citation1] in fission reactors. For long half-life of its ground-state (T 1/2 = 2.11 × 105 years), it is regarded as one of the interesting targets for the nuclear transmutation by neutron capture reaction because the transmuted 100Tcwith short half-life of 15.46 s soon β -decays into stable 100Ru.
A metastable isomer of 99Tc with T 1/2 = 6.015 h, 99m Tc, is excited to the nuclear level with excitation energy Ex = 142.68 keV, and it is of great interest to themedical use of nuclear diagnostics. However, its half-life is too short to carry 99m Tc far from production sites, and to inject it into patients. From the viewpoint of transportation, radioactive 99Mo is a useful nuclide since 99m Tc is the daughter nuclide of 99Mo with T 1/2 = 65.94 h. Molybdenum-99 has been generated by the fission reaction on highly enriched uranium at nuclear reactors. Furthermore, the production of 99Mo by capture reactions on 98Mo with reactor neutrons is now planned in the Japan Materials Testing Reactor of Japan Atomic Energy Agency (JAEA). In contrast to the production methods related with nuclear reactors, it is considered that 99Mo can be created by 99Tc(n, p) reaction with fast neutrons from accelerators [Citation2], using 99Tc extracted from radioactive waste in fission reactors as target material.
In JENDL, significant revision of nuclear data for 99Tc was made for the development of JENDL-3.3 [Citation3]. Hence, the revision in the update to JENDL-4.0 [Citation1] was limited to a change in the neutron width of a negative resonance. The γ-ray production data were not included in the course of those revisions. After the release of JENDL-3.3, capture γ-ray spectra and capture cross-sections were simultaneously measured by anti-Compton NaI(Tl) spectrometer at Tokyo Institute of Technology [Citation4]. Thus, it is now possible to make a reliable evaluation of the γ-ray production data. It should be noted that the 99Tc data in ENDF/B-VII.0 [5,6] and JEFF-3.1.1 [Citation7] were recently updated with the inclusion of γ-ray production data.
The objective of this work is to update the nuclear data (cross-sections, angular distributions, and energy spectra) of 99Tc by taking account of information previously not available. In particular, important is the evaluation of capture cross-section in the keV to MeV region for the nuclear transmutation by accelerator driven system (ADS). The revision of 99Tc(n, p) reaction cross-section is also requisite by means of recent experimental data, in order to accurately estimate the produced amount of 99Mo by fast neutrons. Section 2 explains evaluation method and tools. The calculated results are presented and compared with evaluated and available experimental data in Section 3. The conclusion of this evaluation is described in Section 4.
2. Evaluation method
In this evaluation all reactions, which emit neutrons, protons, deuterons, tritons, 3He, α-particles and γ-rays, were considered if the reaction channels were opened up to 20 MeV. The calculation of neutron-induced reaction cross-sections was done by theoretical nuclear reaction calculation code, CCONE [Citation8]. Although the upper energy of the resolved resonance region was 6 keV in JENDL-4.0, the energy range of the incident neutron was considered from 1 keV to 20 MeV in this work. The lower energy limit presently assumed will be replaced by an up-to-date upper limit of resolved resonance region, when the results of this work are compiled into an ENDF-formatted file [Citation9]. The theoretical models used to calculate the reaction cross-sections were almost the same as those in the previous evaluation of neutron nuclear data for Zn [Citation10] and Cs [Citation11] isotopes. However, since there were a few modifications for the present work, the current setup is described in this section.
2.1. Optical model
Basically, the parameters of optical model potential (OMP) for particle emissions were taken from global OMPs of Koning and Delaroche [Citation12] for neutron and proton, Lohr and Haeberli [Citation13] for deuteron, Becchetti and Greenlees [Citation14] for triton and 3He, and Mcfadden and Satchler [Citation15] for α-particle, but different OMPs were also used for better evaluation as follows.
For neutron OMP of target 99Tc the coupled-channels (CC) optical model based on the rigid rotator model in CCOM code [Citation16] was employed to evaluate the total cross-section and to obtain the neutron transmission coefficient which was applied to statistical model calculation. In the CC calculation the 9/2+ ground-state was coupled with the 11/2+ (Ex = 726.76 keV), 13/2+ (Ex = 761.95 keV) and 15/2+ (Ex = 1526.48 keV) levels. The value of deformation parameter was chosen to be 0.20, whose value is consistent with that of surrounding even–even nuclides derived by Raman et al. [Citation17]
The functional forms of the OMP were taken from Kunieda et al. [Citation18]. The OMP was composed of the realvolume, imaginary surface, imaginary volume, real spin-orbit and imaginary spin-orbit terms. The real volume VR and imaginary surface WD terms are given by the following forms:
Table 1. Values of neutron OMP parameters for real volume and imaginary surface terms for 99Tc.
The resulting neutron total cross-section in the energy range from 10–2 to 20 MeV is shown with theexperimental data of Foster and Glasgow [Citation19] and evaluated data of JENDL-4.0, ENDF/B-VII.0, andJEFF-3.1.1 in . The large discrepancy is found among the present result and the evaluated data in the range of 10−2 to 2 MeV where no experimental information has been obtained yet, and thus, efforts of measurement are expected to resolve this situation. b enlarges the energy range of 1–20 MeV in a for a detailed comparison. The result obtained with the adjusted OMP parameters better reproduces the measured cross-sections than JENDL-4.0.
Figure 1. Total cross-sections for 99Tc in the energy ranges (a) from 10−2 to 20 MeV and (b) from 1 to 20 MeV.
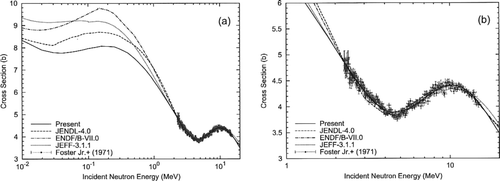
For proton and α-particle emission from Tc isotopes, the OMPs of Perey [Citation20] and Mcfadden and Satchler [Citation15], respectively, were adopted with modified diffuseness parameters of real volume potential, which were changed to 0.52 and 0.45 fm for the proton and α-particle OMPs, respectively. For neutron emissions from 95,97,99Mo the local potentials of Koning and Delaroche [Citation12] were applied.
2.2. Preequilibrium model
The contributions of the preequilibrium process to the reaction cross-section were calculated by applying the two-component exciton model [Citation8]. The global parameterization [Citation21] for the exciton model was adopted. In this prescription the neutron single-particle state density of 100Tc was multiplied by a factor 1.07, which was determined from comparisons with measured cross-sections.
The particle pickup and knockout processes [Citation22] were also included in this evaluation, in order to take into account an additional contribution to the cross-section of (n, α) reaction. The contribution of knockout process was enhanced by a factor of 5.1 to reproduce available experimental data [23,24] of the 99Tc(n, α) reaction cross-section.
The γ-ray emission channel from preequilibrium process was taken into consideration to simulate the direct and semi-direct capture reactions [Citation25].
2.3. Statistical model
The statistical model in the CCONE code was based on the Hauser-Feshbach formalism [Citation26] with width fluctuation correction [Citation27].
2.3.1. Nuclear level density
The data of discrete levels (excitation energy, spin-parity and γ-branching ratios) were taken from Reference Input Parameter Library, RIPL-3 [Citation28]. The nuclear level density was adopted from the formulation by Gilbert and Cameron [Citation29]. This prescription assumed the constant temperature model for lower excitation energies and the Fermi gas model for higher excitation energies, in order to describe the level density above the highest energy Ec of the adopted discrete levels. The level density in the constant temperature model ρT is expressed by
Table 2. Parameter values in the level density formula.
2.3.2. Gamma-ray strength function
The standard Lorentzian form was adopted for γ-ray strength function of E1 radiation. The parameters for electric giant dipole resonance (GDR) were determined so as to reproduce measured capture γ-ray spectrum [Citation4] for 99Tc. It was found that additional small E1 resonances around 6 and 1 MeV were needed to explain the shape of spectra. The former resonance may be called pygmy one. One of the reasons for introduction of the latter correction might be attributed to the insufficient knowledge of nuclear level for100Tc. The parameters determined for GDR and additional small resonances were listed in . The parameters for GDR and so-called pygmy resonance were also applied to other Tc isotopes. The strength functions for the M1 and E2 radiations were adopted from the prescriptions by Kopecky and Uhl [Citation33]. The calculated capture cross-section was normalized to experimental data [Citation4] by multiplying the γ-ray strength function by a factor 0.76. The resulting γ-ray strength function for s-wave resonances in units of 10−4 is 105.5, being consistent with the compiled ones of Mughabghab [Citation34] (108 ± 8) and RIPL-3 (100 ± 14). The obtained result for capture cross-section will be shown in Section 3.1.
Table 3. Resonance parameters for E1 radiation.
3. Evaluated results
In this section, the calculated reaction cross-sections, and γ-ray and neutron emission spectra are compared with measurements and major evaluated nuclear data libraries (JENDL-4.0, ENDF/B-VII.0, and JEFF-3.1.1).
3.1. Capture cross-section
represents the evaluated cross-section of capture reaction with the data of JENDL-4.0, ENDF/B-VII.0, JEFF-3.1.1 and experiments [4,35–39] in the energy range of 10−3 to 20 MeV. The present result, ENDF/B-VII.0 and JEFF-3.1.1 show good agreement with the recent data of Matsumoto et al. [Citation4] and Kobayashi et al. [Citation39] as shown in b. In contrast, JENDL-4.0 reproduced the cross-section measured by Macklin [Citation38], whose data are found to be smaller than the recent ones. At the higher energy of 14.7 MeV Qaim [Citation35] reported the cross-section of 9 ± 2 mbarn. JENDL-4.0 gives large cross-sections above 10 MeV so as to explain his datum. However, Qaim noted that the large cross-section might result from the contamination of low energy neutrons. The present data, therefore, were evaluated without reference to that of Qaim, but with a small bump around 14 MeV which is caused by the direct and semi-direct capture reactions.
Figure 2. Cross-sections of capture reaction on 99Tc in the energy ranges (a) from 10−3 to 20 MeV and (b) from 10−3 to 1 MeV.
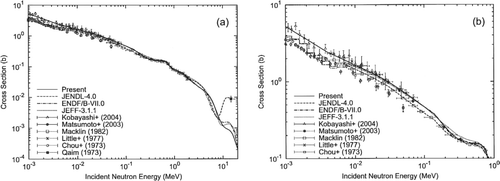
The Maxwellian-averaged cross section (MACS) ofcapture reaction was calculated, combining the resolved resonance data of JENDL-4.0 at 6 keV as shown in , in which also given is the MACS derived from the capture cross-section in JENDL-4.0. It is found that the present result is consistent with the data of Gunsing et al. [Citation40], who obtained the MACS based on a capture experiment in an unresolved resonance region. The calculated MACS is enhanced by 11% at 10 keV and 16% at 30 keV in comparison with that in JENDL-4.0 which is in fairly good agreement with previous data of Winters and Macklin[Citation41]
3.2. (n, p) Reaction cross-section
The cross-sections of 99Tc(n, p) reaction are illustrated in . Recently, Reimer et al. [Citation24] measured the cross-sections in a wide energy range of 8–20 MeV. The present result as well as ENDF/B-VII.0 and JEFF-3.1.1 reasonably reproduces their data. However, experimental data [23,24,35,42] at around 14 MeV provided somewhat larger cross-sections, compared with the present evaluation. JENDL-4.0 only gives cross-sections reasonable to explain the large ones. As a result, the produced amount of 99Mo is by 5–30% smaller than that in the JENDL-4.0 in the energy range between 12 and 20 MeV, whose range isinterested in transmutation of 99Tc in radioactive waste into medically useful 99Mo by D-T neutrons.
3.3. Other reaction cross-sections
The production cross-sections of metastable isomer (Ex = 142.68 keV) by inelastic scattering are calculated and compared to JEFF-3.1.1 and experimental data [23,24,35,43,44] in . In order to explain the measured cross-sections, DWBA calculation to 142.68 keV-level using neutron local OMP [Citation12] for 99Tc was performed with angular momentum transfer L = 4 and normalization parameter β′ = 0.14. The calculated result is in good agreement with the measured data, although Reimer et al. [Citation24] provided somewhat smaller cross-sections below 5 MeV with respect to the present evaluation and JEFF-3.1.1. It is found that the measured cross-section of Qaim [Citation35] is large relative to those of Reimer et al.
Figure 5. Cross-sections of inelastic scattering on 99Tc. Production cross-sections of 142.68 keV-level in present and JEFF-3.1.1 data are compared with experimental ones [23,24,35,43,44].
![Figure 5. Cross-sections of inelastic scattering on 99Tc. Production cross-sections of 142.68 keV-level in present and JEFF-3.1.1 data are compared with experimental ones [23,24,35,43,44].](/cms/asset/e8d31e7f-934f-4354-aae9-fdca4b0cf8fa/tnst_a_649085_o_f0005g.gif)
also shows the calculated cross-sections ofinelastic scattering on 99Tc along with the data of Qaim [Citation35], JENDL-4.0, ENDF/B-VII.0 and JEFF-3.1.1. The cross-section reported by Qaim at 14.7 MeV was not directly based on his experiment, and was deduced from the nuclear structural similarity between 99Tc-99m Tc and 93Nb-93m Nb systems. The present evaluation gives smaller cross-section than the datum, while ENDF/B-VII.0 reasonably explains the derived value. In contrast, JENDL-4.0 has much larger cross-section above 12 MeV.
compares the calculated cross-sections of 99Tc(n, 2n) reaction with JENDL-4.0, ENDF/B-VII.0, JEFF-3.1.1 and the result of Qaim [Citation35]. JENDL-4.0 has a small cross-section at 14 MeV. This is due to the normalization to the cross-section presented by Qaim. It should be, however, noted that the (n, 2n) reaction cross-section of Qaim was based on some estimations. The cause of the small cross-section comes from the deduced large one of inelastic scattering. The present evaluation and JEFF-3.1.1 have similar excitation function and give almost same cross-section (1.52 barn) at 14 MeV.
The cross-section obtained for 99Tc(n, α) reaction is compared with measured ones [23,24,35,42–44] and evaluated data in , and it is consistent with the data measured by Reimer et al. [Citation24] and Ikeda et al. [Citation23]. The experiments by Qaim [Citation35], Goldstein [Citation43] and Filatenkov and Chuvaev [Citation42] give relatively larger cross-sections in comparison with evaluated data. JEFF-3.1.1 has an excitation function which is different from the present result and other evaluations.
represents the calculated cross-section of99Tc(n, n′α) reaction together with measured ones [23,35,44] and evaluated data. The present result is consistent with the upper limit given by Golchert et al. [Citation44] and with the data of Ikeda et al. [Citation23]. The cross-sections in evaluated data are also almost reasonable, compared with the experimental data at around 14MeV. Including the cross-section in this evaluation, they, however, show a difference by 10 mbarn at 20MeV.
3.4. Emission spectra
While the present evaluation considered the emission spectra of neutron, proton, deuteron, triton, 3He, α-particle and γ-ray, the evaluated results of capture γ-ray and neutron emission spectra are given in this section.
The calculated capture γ-ray spectra are compared in with the experimental values of Matsumoto et al. [Citation4], who measured the spectra with incident neutron energies of 8-90 (average: 45), 190, 330 and 540 keV. The present result with supplemental two small E1 resonances listed in reasonably reproduces the measured capture spectra. The spectra with 45 and 540 keV neutrons evaluated in ENDF/B-VII.0 and JEFF-3.1.1 are also plotted in . ENDF/B-VII.0 shows almost flat spectra in the energy range from 0.5 to 3–4 MeV, and then, rapid decrease above 3–4 MeV. In JEFF-3.1.1 the spectra at lower emission energies are in agreement with the measurement. However, the deviation from the experimental values becomes large with increasing γ-ray energies. It should be noted that JENDL-4.0 does not contain the γ-ray production data.
Figure 9. Capture γ-ray spectra on 99Tc. The measured data represented by solid and open circles are taken from Matsumoto et al. [Citation4]. The spectra at 45 and 540 keV in evaluated data of ENDF/B-VII.0 and JEFF-3.1.1 are included for comparison.
![Figure 9. Capture γ-ray spectra on 99Tc. The measured data represented by solid and open circles are taken from Matsumoto et al. [Citation4]. The spectra at 45 and 540 keV in evaluated data of ENDF/B-VII.0 and JEFF-3.1.1 are included for comparison.](/cms/asset/34a60063-c9b4-44c5-8e99-6cf1d8e731e0/tnst_a_649085_o_f0009g.gif)
The calculated neutron emission spectrum at 14MeV is plotted with those of evaluated data in . There has been no experimental information yet. Hence, it is found that the present calculation shows a significant difference from the spectra of evaluated data. In JENDL-4.0, the spectrum has anunreasonable gap at 5 MeV, below which the contribution of neutrons emitted in (n, 2n) reaction is important. The main reason of this shape is that thecross-section of (n, 2n) reaction at 14 MeV was adjusted to a value of 1.23 barn, which is very small in comparison with the presently derived cross-section as seen in . ENDF/B-VII.0 provides spectrum larger than the present evaluation in the energy range from 3 to 12.5 MeV. This result indicates that the contribution of neutrons emitted from the preequilibrium process is larger in the ENDF/B-VII.0 evaluation. JEFF-3.1.1 gives an emission spectrum similar to the present one below 5 MeV, while in 5-12.5 MeV the preequilibrium process shows a larger contribution than in the present evaluation. It should be noted that a pseudo resonance in continuum level is assumed both in ENDF/B-VII.0 and JEFF-3.1.1, but its presence and strength remain still uncertain.
4. Conclusion
The neutron nuclear data of 99Tc were evaluated in the incident neutron energy range from 1 keV to 20 MeV, taking into account recently measured data. Theevaluation was performed by using the CC optical model code, CCOM, and nuclear reaction calculation code, CCONE. The optical model calculation with the adjusted OMP parameters reproduces the available experimental data of total cross-section. The statistical model calculations including the contributions of preequilibrium and direct processes were carried out to obtain the reaction cross-sections and spectra of neutron, proton, deuteron, triton, 3He, α-particle and γ-ray emissions.
The present evaluation made use of the measured data for cross-sections of inelastic scattering, capture, (n, 2n), (n, p), (n, α), and (n, n′α) reactions, and for γ-ray spectra. The calculated cross-sections reasonably explain the experimental values. In comparison with JENDL-4.0, the resulting capture cross-section is enhanced below 1 MeV. In particular, it is larger by over 10% below 200 keV. This result indicates that thetransmutation of 99Tc into stable 100Ru by ADS becomes efficient, relative to the estimation based on the data of JENDL-4.0. The reliable 99Tc(n, p) reaction cross-section is required to estimate the produced amount of 99Mo which β-decays into 99m Tc, with T 1/2 = 65.94 h. The present calculation gives smaller cross-sections than those in JENDL-4.0. This result leads to the reduction in the production efficiency of 99Mo if T(d, n) reaction is considered as neutron source.
The γ-ray emission spectra were evaluated by comparing the present calculation with the capture γ-ray spectra of Matsumoto et al. [Citation4] measured in theincident energy range from 8–90 to 540 keV. The obtained results nicely reproduce the measurements. Itis found that the difference in neutron emission spectra between the calculated result and evaluated data at 14MeV is significant in the secondary energy range from 3 to 12.5 MeV. Hence, experimental work is needed to resolve the disagreement.
The presently evaluated nuclear data of 99Tc including γ-ray emission spectra will be available withup-to-date resolved resonance parameters in the ENDF-6 format.
Acknowledgments
The author would like to thank Dr. T. Matsumoto of The National Institute of Advanced Industrial Science and Technology (AIST) for sending the measured capture γ-ray spectrum data. The author is also grateful to the members ofNuclear Data Center, JAEA, for valuable comments.
References
- Shibata , K. , Iwamoto , O. , Nakagawa , T. , Iwamoto , N. , Ichihara , A. , Kunieda , S. , Chiba , S. , Furutaka , K. , Otuka , N. , Ohsawa , T. , Murata , T. , Matsunobu , H. , Zukeran , A. , Kamada , S. and Katakura , J. 2011 . JENDL-4.0: A new library for nuclear science and engineering . J.Nucl. Sci. Technol , 48 : 1 – 30 .
- Nagai , Y. and Hatsukawa , Y. 2009 . Production of 99Mo for nuclear medicine by 100Mo(n, 2n) 99Mo . J. Phys. Soc. Jpn , 78 : 033201-1 – 033201-4 .
- Shibata , K. , Kawano , T. , Nakagawa , T. , Iwamoto , O. , Katakura , J. , Fukahori , T. , Chiba , S. , Hasegawa , A. , Murata , T. , Matsunobu , H. , Ohsawa , T. , Nakajima , Y. , Yoshida , T. , Zukeran , A. , Kawai , M. , Baba , M. , Ishikawa , M. , Asami , T. , Watanabe , T. , Watanabe , Y. , Igashira , M. , Yamamuro , N. , Kitazawa , H. , Yamano , N. and Takano , H. 2002 . Japanese evaluated nuclear data library version 3 revision-3: JENDL-3.3 . J. Nucl. Sci. Technol , 39 : 1125 – 1136 .
- Matsumoto , T. , Igashira , M. and Ohsaki , T. 2003 . Measurement of keV-neutron capture cross sections and capture gamma-ray spectra of 99Tc . J. Nucl. Sci. Technol , 40 : 61 – 68 .
- Rochman , D. , Herman , M. , Mughabghab , S.F. and Obložinský , P. 2008 . New evaluation of the 99Tc neutron-induced cross sections for the ENDF/B-VII.0 Library . Nucl. Sci. Eng , 158 : 68 – 77 .
- Chadwick , M.B. , Oblozinsky , P. , Herman , M. , Greene , N.M , McKnight , R.D , Smith , D.L , Young , P.G , MacFarlane , R.E , Hale , G.M , Frankle , S.C , Kahler , A.C , Kawano , T , Little , R.C , Madland , D.G , Moller , P , Mosteller , R.D , Page , P.R , Talou , P , Trellue , H , White , M.C , Wilson , W.B , Arcilla , R , Dunford , C.L , Mughabghab , S.F , Pritychenko , B , Rochman , D , Sonzogni , A.A , Lubitz , C.R , Trumbull , T.H , Weinman , J.P , Brown , D.A , Cullen , D.E , Heinrichs , D.P , McNabb , D.P , Derrien , H , Dunn , M.E , Larson , N.M , Leal , L.C , Carlson , A.D , Block , R.C , Briggs , J.B , Cheng , E.T , Huria , H.C , Zerkle , M.L , Kozier , K.S , Courcelle , A , Pronyaev , V and van der Marck , S.C . 2006 . ENDF/B-VII.0: Next generation evaluated nuclear data library for nuclear science and technology . Nucl. Data Sheets , 107 : 2931 – 3060 .
- Santamarina , A. , Bernard , D. and Rugama , Y. 2009 . TheJEFF-3.1.1 nuclear data library JEFF Report 22
- Iwamoto , O. 2007 . Development of a comprehensive code for nuclear data evaluation, CCONE, and validation using neutron-induced cross sections for uranium isotopes . J.Nucl. Sci. Technol , 44 : 687 – 697 .
- Herman , M. and Trkov , A. 2009 . ENDF-6 formats manual, data formats and procedures for the evaluated nuclear data file, ENDF/B-VI and ENDF/B-VII BNL-90365-2009
- Iwamoto , N. 2007 . New evaluation of neutron nuclear data for zinc isotopes . J. Nucl. Sci. Technol , 44 : 1131 – 1141 .
- Iwamoto , N. 2011 . Neutron nuclear data evaluation of cesium isotopes for JENDL-4.0 . J. Nucl. Sci. Technol , 48 : 1238 – 1249 .
- Koning , A.J. and Delaroche , J.P. 2003 . Local and global nucleon optical models from 1 keV to 200 MeV . Nucl. Phys , A713 : 231 – 310 .
- Lohr , J.M. and Haeberli , W. 1974 . Elastic scattering of 9-13 MeV vector polarized deuterons . Nucl. Phys , A232 : 381 – 397 .
- Becchetti , F.D. Jr. and Greenlees , G.W. 1971 . “ A General Set of 3He and Triton Optical-Model Potentials for A > 40, E < 40 MeV ” . In Polarization Phenomena in Nuclear Reactions , Edited by: Barshall , H.H. and Haeberli , W. 682 – 683 . Madison : University of Winsconsin Press .
- McFadden , L. and Satchler , G.R. 1966 . Optical-model analysis of the scattering of 24.7 MeV alpha particles . Nucl. Phys , 84 : 177 – 200 .
- Iwamoto , O. 2003 . Program CCOM – coupled channels optical model calculation with automatic parameter search JAERI-Data/Code 2003-020, Japan Atomic Energy Research Institute
- Raman , S. , Nestor , C.W. , Jr. and Tikkanen , P. 2001 . Transition probability from the ground to the first-excited 2+ state of even-even nucleus . Atom. Data Nucl. Data Tables , 78 : 1 – 128 .
- Kunieda , S. , Chiba , S. , Shibata , K. , Ichihara , A. Sukhovitskii , E.Sh. Systematical nucleon-induced optical model analysis for medium and heavy nuclei with coupled-channel framework . Proceedings of International Conference on Nuclear Data forScience and Technology 2007 . pp. 227 – 230 . Les Ulis Cedex A .
- Foster , D.G. Jr. and Glasgow , D.W. 1971 . Neutron total cross sections, 2.5–15 MeV. I. experimental . Phys. Rev , C3 : 576 – 603 .
- Perey , F.G. 1963 . Optical-model analysis of proton elastic scattering in the range of 9 to 22 MeV . Phys. Rev , 131 : 745 – 763 .
- Koning , A.J. and Duijvestijn , M.C. 2004 . A global pre-equilibrium analysis from 7 to 200 MeV based on the optical model potential . Nucl. Phys , A744 : 15 – 76 .
- Kalbach , C. 1977 . The Griffin model, complex particles and direct nuclear reactions . Z. Phys , A283 : 401 – 411 .
- Ikeda , Y. , Cheng , E.T. , Konno , C. and Maekawa , H. 1994 . Measurement of neutron activation cross sections for the 99Tc(n, p) 99Mo, 99Tc(n, α) 96Nb, 99Tc(n, n′α) 95Nb, and 99Tc(n, n′) 99m Tc Reactions at 13.5 and 14.8 MeV . Nucl. Sci. Eng , 116 : 28 – 34 .
- Reimer , P. , Koning , A.J. , Plompen , A.J.M. , Qaim , S.M. and Sudar , S. 2009 . Neutron induced reaction cross sections for the radioactive target nucleus 99Tc . Nucl. Phys , A815 : 1 – 17 .
- Akkermans , J.M. and Gruppelaar , H. 1985 . Analysis of continuum gamma-ray emission in precompound-decay reactions . Phys. Lett , 157B : 95 – 100 .
- Hauser , W. and Feshbach , H. 1952 . The inelastic scattering of neutrons . Phys. Rev , 87 : 366 – 373 .
- Moldauer , P.A. 1980 . Statistics and the average cross section . Nucl. Phys , A344 : 185 – 195 .
- Capote , R. , Herman , M. , Oblozinsky , P. , Young , P.G. , Goriely , S. , Belgya , T. , Ignatyuk , A.V. , Koning , A.J. , Hilaire , S. , Plujko , V.A. , Avrigeanu , M. , Bersillon , O. , Chadwick , M.B. , Fukahori , T. , Ge , Zhigang , Han , Yinlu , Kailas , S. , Kopecky , J. , Maslov , V.M. , Reffo , G. , Sin , M. , Soukhovitskii , E.Sh. and Talou , P. 2009 . RIPL – Reference Input Parameter Library for Calculation of Nuclear Reactions and Nuclear Data Evaluations . Nucl. Data Sheets , 110 : 3107 – 3214 .
- Gilbert , A. and Cameron , A.G.W. 1965 . A composite nuclear level density formula with shell corrections . Can. J. Phys , 43 : 1446 – 1496 .
- Mengoni , A. and Nakajima , Y. 1994 . Fermi-gas model Parametrization of nuclear level density . J. Nucl. Sci. Technol , 31 : 151 – 162 .
- Audi , G. , Wapstra , A.H. and Thibault , C. 2003 . The AME2003 atomic mass evaluation (II) . Nucl. Phys , A729 : 337 – 676 .
- Myers , W.D. and Swiatecki , W.J. 1966 . Nuclear masses and deformations . Nucl. Phys , 81 : 1 – 60 .
- Kopecky , J. and Uhl , M. 1990 . Test of gamma-ray strength functions in nuclear reaction model calculations . Phys. Rev , C41 : 1941 – 1955 .
- Mughabghab , S.F. 2006 . Atlas of Neutron Resonances: Resonance Parameters And Thermal Cross Sections Z = 1–100 , Amsterdam : Elsevier Science .
- Qaim , S.M. 1973 . Nuclear reaction cross-sections for 14.7 MeV neutrons on 99Tc . J. Inorg. Nucl. Chem , 35 : 3669 – 3675 .
- Chou , J.-C. and Werle , H. 1973 . (n, γ)-cross-section measurements of 99Tc, Eu, Sm and Fe in the energy range 1 eV to 50 keV with a slowing-down time spectrometer . J.Nucl. Energy , 27 : 811 – 823 .
- Little , R.C. and Block , R.C. 1977 . Neutron capture cross-section measurements of 99Tc up to 80 keV . Trans. Am. Nucl. Soc , 26 : 574
- Macklin , R.L. 1982 . Technetium-99 neutron capture cross section . Nucl. Sci. Eng , 81 : 520 – 524 .
- Kobayashi , K. , Lee , S. , Yamamoto , S. and Kawano , T. 2004 . Neutron capture cross-section measurement of 99Tc by linac time-of-flight method and the resonance analysis . Nucl. Sci. Eng , 146 : 209 – 220 .
- Gunsing , F. , Leprêtre , A. , Mounier , C. , Raepsaet , C. , Bastian , C. , Corvi , F. and Gonzalez , J. 2001 . Stellar neutron capture cross section of 99Tc . Nucl. Phys , A688 : 496 – 498 .
- Winters , R.R. and Macklin , R.L. 1987 . Maxwellian-averaged neutron capture cross sections for 99Tc and 95–98Mo . Astrophys. J , 313 : 808 – 812 .
- Filatenkov , A.A. and Chuvaev , S.V. 2001 . Measurement of a set of badly known neutron induced cross sections , St.Petersburg : Khlopin Radiev Institute . Technical Report RI-258
- Goldstein , G. 1966 . Activation cross sections for the reactions of 14.8-MeV neutrons with 99Tc . J. Inorg. Nucl. Chem , 28 : 676 – 678 .
- Golchert , N.W. , Gardner , D.G. and Sedlet , J. 1965 . Cross section of some reactions of 99Tc with 14.1MeV neutrons . Nucl. Phys , 73 : 349 – 352 .