Abstract
Migration radionuclides in an underground environment are one of the major concerns in the safety assessment of a geological repository. Biofilms can have an impact on the transport of radionuclides in several ways: (1) by acting as a barrier to radionuclide sorption onto geological surfaces, or (2) by providing a sorption site for radionuclides, or (3) by trapping many things, including radionuclides. Little is known about bacterial effects on the biofilm formation deep underground. In this study, we isolated bacterial strains from deep groundwater and evaluated the biofilm formation abilities of these strains by crystal violet assay. Bacterial strains were isolated from ground-water collected at –140 m in the 07-V140-M01 borehole at the Horonobe Underground Research Center, Japan. The crystal violet assay showed that 98% of the isolated strains had biofilm formation abilities under tested conditions. This result suggested that biofilm formation must not be neglected in the study of migration radionuclides in nuclear waste repositories. The isolated strains produced differential amounts of biofilm, although they were identified as the same Pseudomonas species, suggesting that biofilm formation abilities varied at different strain levels. These results support the conclusion that the assessment of biofilm impact on the transport of radionuclides in a geological repository must consider the variation in biofilm formation as a function of strain level.
1. Introduction
In Japan, geological repositories are proposed by the implementing agency, the Nuclear Waste Management Organization of Japan (NUMO), for disposal of high-level radioactive waste that is produced by nuclear power generation. Migration of radionuclides in an underground environment is one of the major concerns in the safety assessment of a geological repository. Host rocks are expected to adsorb radionuclides and thereby reduce their migration in a subsurface environment [Citation1]. Their reaction at the interfaces of rock and ground-water are important for the assessment of a geological repository site. Biofilms are bacterial populations attached to solid surfaces and enclosed in extracellular polymeric substances [Citation2–Citation4]. The rocks' adsorption capacity for radionuclides was decreased by biofilm that had grown on rock surfaces, even though microorganisms possess a high affinity for the accumulation of metal ions [Citation5–Citation8]. Thus, biofilms are important for the safety assessment of a geological repository. But little is known about bacterial effects on the biofilm formation deep underground. In order to obtain the biofilm formation data for deep subsurface bacteria, we isolated bacterial strains from deep groundwater and evaluated the biofilm formation abilities of these strains.
2. Experimental procedure
2.1. Isolation of bacteria
The groundwater sample was collected at –140 m in the 07-V140-M01 borehole at the Horonobe Underground Research Center, which is located in the city of Horonobe, Hokkaido, Japan. The water temperature of the groundwater was 30°C. The collected groundwater was spread on a plate consisting of nutrient broth (Difco) and ager (Wako) and then cultured aerobically at 30°C, which was equal to the temperature of the groundwater at the sampling site. Next, a colony was picked up and spread on a new plate consisting of nutrient broth and ager, and again cultured aerobically at 30°C. This procedure was repeated twice in order to obtain a purified bacterial strain.
2.2. Identification of isolated strains by 16S rRNA gene sequencing
The isolated strains were cultivated overnight. These cultures were applied as templates for Polymerase Chain Reaction (PCR). The 16S rRNA gene was amplified by PCR using PrimeSTAR (Takara Bio Co.) according to the manufacturer's instructions. The primers used for the PCR reactions were 27F (5′-AGAGTTTGATCCTGGCTCAG-3′) [Citation9] and 1525r (5′-AAAGGAGGTGATCCAGCC-3′) [Citation10]. The reaction conditions were as follows: 30 cycles of 98°C for 10 s, 55°C for 5 s and 72°C for 10 s. The PCR products were purified enzymatically and applied for sequencing. The purified PCR products were sequenced with the ABI Big Dye Terminator Cycle Sequencing kit (Applied Biosystems) and an ABI genetic analyzer (Model 3130/3130xl Applied Biosystems). The primer used for the sequencing was 27F (5′-AGAGTTTGATCCTGGCTCAG-3′). The nucleotide sequences of the 44 isolates in this study were deposited in the GenBank nucleotide database under accession numbers AB627863 to AB627906. The obtained sequences were compared with sequences in the NCBI nucleotide sequence database using the Basic Local Alignment Search Tool (BLAST) program [Citation11]. The isolated strains were tentatively identified by the sequences that showed highest similarity to those of the NCBI strains, although further phylogenetic analysis is required to determine detailed phylogenetic affiliations of the isolated strains.
2.3. Crystal violet assay
The biofilm formation ability of isolated strains was evaluated by the crystal violet assay method using a multi-well plate. Crystal violet is a dye that binds to cells and extracellular polymeric substances of biofilm formed on a well surface. The crystal violet, which is bound to cells and extracellular polymeric substances of biofilm, is eluted by a 100% ethanol solution. The amount of crystal violet dissolved in 100% ethanol is measured by the absorbance at 590 nm. The absorbance measured at 590 nm is determined to be the amount of biofilm formed on the well surface. The detailed procedure of crystal violet assay is described as follows. Nutrient broth was applied as a medium for this assay. For an anaerobic culture, nutrient broth was supplemented with 20 mM sodium nitrate (Wako). The amount of cells in the nutrient broth was measured by absorbance at 600 nm. The isolated strain was grown aerobically in 2 mL nutrient broth in a test tube. This cell solution was then added to a well containing 500 μl of nutrient broth until the value of absorbance at 600 nm of the broth reached 0.03 in order to establish the initial amount of cells in the broth at the starting point of biofilm cultivation. This plate was incubated statically at 30°C under either aerobic or anaerobic conditions. The plate was sealed in an anaerobic box using by an AneroPak environment generator (Mitsubishi Gas Chemical) for anaerobic culturing. After incubation, the nutrient broth was removed from the well and the well was washed with 700 μl of water to remove planktonic cells. Biofilms formed on the well surface were stained for 15 min at room temperature with 500 μl/well of 1% crystal violet (Merck) solution. After staining, the crystal violet solution was removed from the well. The well was washed twice with 700 μl of water and then the biofilm-bounded crystal violet was dissolved in 500 μl of 100% ethanol. The absorbance at 590 nm of the eluent (A590 ) was determined with a spectrophotometer (SmartSpec Plus, Bio-Rad). For measurement of background staining, the same procedure described above was performed without the step of addition of cell solution into a broth. Both the Horo_26 and Horo_15 strains reached maximum biofilm formation at 22 hr (aerobic condition) or 72 hr (anaerobic condition). To evaluate maximum biofilm formation abilities of the isolated strains, we collected all biofilm samples at 22 hr (aerobic condition) or 72 hr (anaerobic condition).
3. Results and discussion
The groundwater sample was collected at –140 m in the 07-V140-M01 borehole at the Horonobe Underground Research Center. The water temperature of the groundwater was 30°C. The borehole is located in the Miocene mudstone of the Koetoi Formation, which trapped paleo-seawater. Forty-four bacterial strains were isolated from the groundwater sample under the conditions described in the experimental procedure. In the safety assessment, the maximum risk for a geological repository must be estimated. The aim of this study is to analyze the maximum ability of deep subsurface bacteria for biofilm formation. Therefore, nutrient broth, which is popular as a medium for sufficient growth of many bacteria, was selected for cultivation of the subsurface bacteria.
The isolated 44 strains were identified by analyzing their 16S rRNA gene sequences. This analysis was performed by the steps described in the experimental procedure. The ID number and species name of the sequences that showed the highest similarity to isolated strains from the NCBI database shown in . Forty-one strains were identified as Pseudomonas species (). It is unclear why the Pseudomonas species was isolated from the groundwater sample. It is known that this species is widely present in various environments, including soil, sea water, sea sediment, animal, and plant, because of the variety of metabolic patterns of this bacterium [Citation12–Citation14]. The groundwater sample used in this study was collected from the borehole located in mudstone that had trapped paleo-seawater. This particular environment might contribute to the presence of Pseudomonas species at the site.
Table 1. Preliminary identification of isolated strains by 16S rRNA gene sequencing. Accession number indicates the ID number of 16S rRNA gene sequence of the strain isolated in this study. The gene sequences of isolated strains were compared with sequences in the NCBI nucleotide sequence database using the Basic Local Alignment Search Tool (BLAST) program [Citation11]. The isolated strains were tentatively identified by the sequences showed highest similarity to those of isolated strains. The ID number and species name of the sequences that showed highest similarity to those of isolated strains were listed in this table.
The biofilm formation ability of the isolated strain was evaluated by the crystal violet assay method using a multi-well plate [Citation15]. The A 590 values are shown in . Ninety-eight percent of the isolated strains showed a two-fold or greater difference in the A 590 values between the strains and the background when grown under aerobic conditions with 95% confidence (a). The same was true when the strains were grown anaerobically (b). These results strongly suggest that 98% of the isolated strains considered in this study have biofilm formation abilities under the tested conditions. This result indicates that most of the deep subsurface bacteria isolated from the Horonobe site have biofilm formation abilities, at least under the tested conditions, although it is not certain whether the isolated strains form biofilm under the environmental conditions present at the Horonobe site. Biofilms growing on rock surfaces decrease the rocks' adsorption capacity for radionuclides [Citation7,Citation8]. The biofilm formation by most of the strains isolated from the deep subsurface suggests that biofilm formation must not be neglected in the study of migration radionuclides in nuclear waste repositories. The Pseudomonas species is capable of aerobic and anaerobic growth with oxygen and nitrate as the terminal electron acceptor [Citation16–Citation18]. Because dissolved oxygen left in buffer materials and backfill materials in nuclear waste repositories are consumed by geochemical and microbial processes [Citation19], the growth conditions for subsurface bacteria shift from aerobic to anaerobic. The Pseudomonas strains in this study showed biofilm growth under both aerobic and anaerobic conditions ( and ), indicating that this species play an important role in biofilm formation, although isolated strains were cultivated in nutrient rich conditions in this study. Although 93% of the isolated strains were affiliated to same genus Pseudomonas, they showed differential A 590 values (aerobic condition, 0.30–1.44; anaerobic condition, 0.29–1.73) ( and ). This result indicates that biofilm formation abilities of these strains varied with strain level. Therefore the strain level-biofilm formation ability must be considered when assessing the impact of biofilm on the transport of radionuclides in a geological repository. In Pseudomonas aeruginosa, the deficient strain of flgK, pilY and pilB genes encoding flagellar and pili did not develop biofilm [Citation15]. Elucidation of the compositions of these genes for the strains tested in this study may help in the development of a genetic marker for rapid evaluation of biofilm formation abilities in deep subsurface environments. To more closely model the sampling site, the experimental system will need to use rocks and ground-water for biofilm cultivation instead of a multi-well plate and nutrient broth. Furthermore, analysis of biofilm formation by archaea will be required to understand the biofilm formation property at the site.
Figure 1. Absorbance measured at 590 nm after treated by crystal violet assay for the isolated strains under aerobic (a) and anaerobic (b) conditions. Data are the average of the absorbance at 590 nm of each strain obtained from duplicate experiments. Error bars indicate standard deviations from the means. White bar indicates background. Asterisks indicate the strains that did not show 2-fold or greater difference in the A 590 values between the strains and the background with 95% confidence.
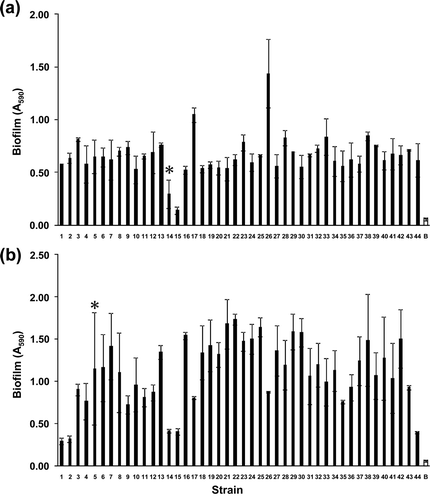
Acknowledgements
This work was supported by a grant from the Ministry of Economy, Trade and Industry of Japan.
References
- Neretnieks , I. 1980 . Diffusion in the rock matrix: an important factor in radionuclide retardation? . J. Geophys. Res , 85 : 4379 – 4397 .
- Costerton , J. W. , Lewandowski , Z. , Caldwell , D. E. , Korber , D. R. and Lappin-Scott , H. M. 1995 . Microbial biofilms . Annu. Rev. Microbiol , 49 : 711 – 745 .
- Costerton , J. W. , Stewart , P. S. and E.P. 1999 . Greenberg, Bacterial biofilms: a common cause of persistent infections . Science , 284 : 1318 – 1322 .
- Davey , M. E. and O'Tool , G. A. 2000 . Microbial biofilms: from ecology to molecular genetics . Microbiolo. Mol. Biol Rev , 64 : 847 – 867 .
- Kozai , N. , Ohnuki , T. , Sakamoto , F. , Suzuki , Y. , Tanaka , K. , Iefuji , H. and Sakai , T. 2011 . Accumulation of Co in yeast cells under metabolically active condition-Implication for role of yeast in migration of radioactive Co- . J. Nucl. Sci. Technol , 48 : 1206 – 1213 .
- Ishii , N. , Koiso , H. , Takeda , H. and Uchida , S. 2010 . Partitioning of 14C into solid, liquid, and gas phases in various paddy soils in Japan . J. Nucl. Sci. Technol , 47 : 238 – 243 .
- Anderson , C. , Pedersen , K. and Jakobsson , A. M. 2006 . Autoradiographic comparisons of radionuclide adsorption between subsurface anaerobic biofilms and granitic host rocks . Geomicrobiol. J , 23 : 15 – 29 .
- Anderson , C. , Jakobsson , A. M. and Pedersen , K. 2007 . Influence of in situ biofilm coverage on the radionuclide adsorption capacity of subsurface granite . Environ. Sci. Technol , 41 : 830 – 836 .
- Mincer , T. J. , Jensen , P. R. , Kauffman , C. A. and Fenical , W. 2002 . Widespread and persistent populations of a major new marine actinomycete taxon in ocean sediments . Appl. Environ. Microbiol , 68 : 5005 – 5011 .
- Maruyama , A. , Taniguchi , R. , Tanaka , H. , Ishiwata , H. and Higashihara , T. 1997 . Low-temperature adaptation of deep-sea bacteria isolated from the Japan Trench . Marine Biol , 128 : 705 – 711 .
- Pearson , W. R. and Lipman , D. J. 1988 . Improved tools for biological sequence comparison . Proc. Natl. Acad. Sci. USA , 85 : 2444 – 2448 .
- Hardalo , C. and Edberg , S. C. 1997 . Pseudomonas aeruginosa: assessment of risk from drinking water . Crit. Rev. Microbiol , 23 : 47 – 75 .
- Boniek , D. , Figueiredo , D. , Pylro , V. S. and Duarte , G. F. 2010 . Characterization of bacterial strains capable of desulphurization in soil and sediment samples from Antarctica . Extremophiles , 14 : 475 – 481 .
- Stover , C. K. , Pham , X. Q. , Erwin , A. L. , Mizouchi , S. D. , Warrener , P. , Hickey , M. J. , Brinkman , F. S.L. , Hufnagle , W. O. , Kowalik , D. J. , Lagrou , M. , Garber , R. L. , Goltry , L. , Tolentino , E. , Westbrock-Wadman , S. , Yuan , Y. , Brody , L. L. , Coulter , S. N. , Folger , K. , Kas , R. , Larbig , K. , Lim , R. , Smith , K. , Spencer , D. , Wong , G.K.-S. , Wu , Z. , Paulsen , I. T. , Reizer , J. , Saier , M. H. , Hancock , R. E.W. , Lory , S. and Olson , M. V. 2000 . Complete genome sequence of Pseudomonas aeruginosa PAO1, an opportunistic pathogen . Nature , 406 : 959 – 964 .
- O'Tool , G. A. and Kolter , R. 1998 . Flagellar and twitching motility are necessary for Pseudomonas aeruginosa biofilm development . Mol. Microbiol , 30 : 295 – 304 .
- Koike , I. and Hattori , A. 1975 . Growth yield of a denitrifying bacterium, Pseudomonas denitrificans, under aerobic and denitrifying conditions . J. Gen. Microbiol , 88 : 1 – 10 .
- Koike , I. and Hattori , A. 1975 . Energy yield of denitrification: an estimate from growth yield in continuous cultures of Pseudomonas denitrificans under nitrate-, nitrite- and nitrous oxide-limited conditions . J. Gen. Microbiol , 88 : 11 – 19 .
- Williams , H. D. , Zlosnik , J. E. and Ryall , B. 2007 . Oxygen, cyanide and energy generation in the cystic fibrosis pathogen Pseudomonas aeruginosa . Adv. Microb. Physiol , 52 : 1 – 71 .
- Yang , C. , Samper , J. , Molinero , J. and Bonilla , M. 2007 . Modelling geochemicaland microbial consumption of dissolved oxygen after backfilling a high level radioactive waste repository . J. Contaminant Hydrol , 93 : 130 – 148 .