Abstract
Breakthrough mode liquid chromatography was employed to study calcium isotope fractionation. Highly porous silica beads, the inner pores of which were embedded with a benzo-18-crown-6 ether resin or a benzo-15-crown-5 ether resin, were used as column packing material. For both the resins, enrichment of heavier isotopes of calcium was observed in the frontal part of their respective calcium chromatograms. The values of the isotope fractionation coefficient were on the order of 10−3 and 10−4 for the benzo-18-crown-6 ether and benzo-15-crown-5 ether resins, respectively. The observed isotope fractionation coefficient was dependent on the concentration of hydrochloric acid in the calcium feed solution; a higher hydrochloric acid concentration resulted in a smaller fractionation coefficient value. The present calcium isotope effects were most probably mass dependent, indicating they came from isotope effects based on molecular vibration. Molecular orbital calculations supported the present experimental results in a qualitative fashion.
1. Introduction
Naturally occurring calcium (Ca) consists of six isotopes, 40Ca, 42Ca, 43Ca, 44Ca, 46Ca, and 48Ca, among which 40Ca with double magic numbers is the most abundant. Ca isotopes have applications in various research fields ranging from medicine to astrophysics. For instance, 48Ca is an important nuclide to synthesize super-heavy elements and is to be used in research on neutrino-less double beta decay [Citation1]. Due to its low natural abundance (0.19%), however, it must be concentrated prior to applications. 47Ca, a radioisotope of Ca, which is produced from 48Ca by neutron bombardment, has certain medical and biological research applications because of its short half-life (four days) and convenient β and γ spectra [Citation2].
Several methods for Ca isotope separation and enrichment have been reported. The electromagnetic method (Calutron) is too costly and impractical for large-scale production of enriched Ca isotopes. Another method is a redox system method which is based on reported Ca isotope effects between two different oxidation states of Ca (0 and + 2) for the redox systems with host materials of Ca insertion being mercury (Hg) [Citation3] or graphite [Citation4]. The former redox system with aneffective isotope fractionation coefficient, ε, of 8 × 10−3 for the 48Ca/40Ca isotopic pair is attractive for small pre-enrichment of heavier Ca isotopes [Citation3], but the use of toxic Hg entails biological and environmental problems and this system would be difficult to apply to large-scale isotope enrichment. The redox system with graphite [Citation4] to obtain enriched Ca isotopes is even less technically feasible than the Hg system.
In general, chromatographic processes based on chemical exchanges are desirable for large-scale production of enriched isotopes due to operational simplicity and easy accumulation of small single-stage isotope fractions. As chromatographic column packing materials, those having crown ethers and cryptands as functional groups and ion exchange resins including chelating resins, have been reported. Ion exchange chromatographic processes using strongly acidic cation exchange resins showed only small ε values and are likely impractical [Citation5,Citation6]. Processes using chromatographic columns packed with polymer-bound cryptands, although showing ε values on the order of 10−3, are also impractical as a consequence of slow exchange rates [Citation7–Citation9].
A chromatographic Ca isotope separation process with a crown ether resin was shown to be an improvement over those with cryptands [Citation9,Citation10]. Recently, accumulation of Ca isotope fractions was developed for liquid chromatography using benzo-18-crown-6 ether resin as column packing material, and ε values on the order of 10−3 were reported [Citation11,Citation12]. Liquid chromatography with crown ether resins may be a promising process for large-scale production of enriched Ca isotopes.
In the present paper, which constitutes a part of our study on isotope effects of the group 2 metals in crown ether systems [Citation13], we investigated Ca isotope fractionation in chromatographic systems with crown ether resins in detail. In addition, we conducted molecular orbital (MO) calculations for elucidation of the Ca isotope effects observed in those experiments. The chromatographic experiments and MO calculations and their results are described here.
2. Experimental
2.1. Crown ether resins and other materials
Two kinds of crown ether resins, benzo-18-crown-6 ether (B18C6) resin, and benzo-15-crown-5 ether (B15C5) resin, both synthesized at the Research Laboratory for Nuclear Reactors, Tokyo Institute of Technology, were employed. The B18C6 resin, embedded in the inner pores of highly porous silica beads (diameter: 40 to 60 μm), is a copolymer ofphenol and monobenzo-18-crown-6 ether that provide functional groups [Citation14]. The B15C5 resin is similar to the B18C6 resin except that the functional groups are monobenzo-15-crown-5 ether instead of monobenzo-18-crown-6 ether [Citation11]. All reagents were of analytical-reagent grade and used without further purification.
2.2. Measurements of distribution coefficients (Kd) ofCa
Distribution coefficients of Ca are defined as
For the B15C5 resin, a similar experimental procedure was followed except that the resin column height was 100 cm instead of 30 cm for the B18C6 resin.
The distribution coefficient was calculated using the equation
2.3. Ca isotope fractionation experiments
Chromatography was done in the breakthrough mode using two or five Pyrex glass columns (100 cm length and 0.8 cm diameter) packed with the resin andconnected in series with polytetrafluoroethylene (PTFE) tubes (1 mm diameter). The resin column was first washed with HCl solution with the same HCl concentration as that of Ca feed solution. The aqueous HCl solution containing CaCl2 (feed solution) of the natural abundances of the Ca isotopes was introduced continuously from the top of the first column at a constant flow rate controlled with a high-pressure pump (Nihon Seimitsu Kagaku NP-KX-105U high-pressure pump) and it flowed through all the columns. The effluent from the bottom of the final (second or fifth) column was collected into small fractions and analyzed for Ca contents and Ca isotopic compositions. The experimental temperature was kept constant at 10, 25, 40, or 50°C by circulating thermostatted water through jackets surrounding the columns.
Experimental conditions are summarized in .
Table 1. Experimental conditions and results other than isotopic data.
2.4. Analyses
The Ca2+ ion concentrations in effluent fractions and feed solutions were measured after appropriate dilution by inductively coupled plasma atomic emission spectrometry (ICP-AES; Seiko Instruments SPS 7700 ICP-atomic emission spectrometer). For the Ca isotope analysis, the chemical form of Ca in effluent fractions and feed solutions was converted from CaCl2 to calcium iodide (CaI2) through anion exchange (Muromac, 1 × 8, 200–400 mesh, OH form) followed by addition of hydroiodic acid (HI) [Citation4,Citation12]. Ca isotopic ratios (42Ca/40Ca, 43Ca/40Ca, 44Ca/40Ca and 48Ca/40Ca) of the selected fractions of the effluents in the frontal part of the Ca adsorption zones were determined by thermal ionization mass spectrometry (Finnigan MAT261 mass spectrometer) [Citation4,Citation12]. The standard deviations were typically 0.08 to 0.21%, 0.13 to 0.32%, 0.09 to 0.18% and 0.13 to 0.32% for the 42Ca/40Ca, 43Ca/40Ca, 44Ca/40Ca and 48Ca/40Ca isotopic pairs, respectively.
2.5. MO calculations
To elucidate the Ca isotope effects observed in the present chromatographic Ca isotope fractionation experiments in a qualitative fashion, MO calculations of the reduced partition function ratios (rpfrs) of Ca species expected to be involved in the experiments were carried out.
The Ca isotope exchange reaction that gives rise tothe present Ca isotope effects may be expressed, for the 42Ca/40Ca, 43Ca/40Ca, 44Ca/40Ca, and 48Ca/40Ca isotopic pairs, as
The general expression for the rpfr is, under Born–Oppenheimer and harmonic oscillator approximations, given as [Citation15],
As model Ca species in aqueous solution, we considered a Ca2+ ion with the solvation number of six or seven, surrounded by water molecules and additionally a chloride (Cl−) ion, [Ca2+(H2O)6], [Ca2+(H2O)6](H2O), [Ca2+(H2O)7], [Ca2+(H2O)5Cl−], and [Ca2+(H2O)6Cl−], in which water (H2O) molecules and a Cl− ion in the brackets denote that they are in the primary solvation sphere of the Ca2+ ion, directly interacting with the Ca2+ ion. As model Ca species inthe crown ether phase of the B18C6 resin system, weconsidered clusters consisting of a B18C6 molecule and a CaCl2 without or with up to two HCl molecules ([B18C6 + CaCl2], [B18C6 + CaCl2 + HCl], and [B18C6 + CaCl2 + 2HCl]). Similarly, [B15C5 + CaCl2], [B15C5 + CaCl2 + HCl], and [B15C5 + CaCl2 + 2HCl] were considered as model Ca species in the crown ether phase of the B15C5 resin system. Inclusion of HCl molecules in the crown ether phases was inferred from the results of strontium (Sr) isotope fractionation in a solvent extraction system reported by Shibahara et al. [Citation16]. For both the B18C6 and B15C5 resins, the existence of organic networks of the resins and H2O molecules was ignored.
Calculations were made at the B3LYP/6-31G(d) level of theory using the Gaussian 03 and 98 program packages (Gaussian Inc.) [Citation17]. In our previous paper onboron (B) isotope fractionations between the boric acid (B(OH)3) molecule and the monoborate anion (B(OH)4 −) [Citation18], we showed that a more advanced MO theory with a higher basis level basis set did not necessarily yield better rpfr results. The B3LYP theory is probably the most commonly used one in computational chemistry nowadays, and the 6-31G(d) basis set, although not a large one, may be one of the most standard basis sets. The Gauss View (Gaussian Inc.) and the Free Wheel programs (Butch Software Studio) were used for the graphics. No frequency correction was made since we were only concerned with relative magnitudes of the rpfrs of Ca species and of equilibrium constants of Ca isotope exchange reactions. For each species considered, the vibrational analysis was performed after the structure optimization, and using the calculated frequencies, we estimated the rpfrs and equilibrium constants for the Ca isotope exchange reactions.
3. Results and discussion
3.1. Distribution coefficients of Ca
The measured K d value of Ca2+ ion was plotted against the HCl concentration in the feed solution in . As is seen in the figure, the B18C6 resin works as a Ca2+ ion adsorbent at pH values of about 4 and higher, and the B15C5 resin does so at pH values of about 6 and higher. For both resins, the measured K d value is an increasing function of the HCl concentration at both 25 and 40°C, which means the resins are better Ca ion adsorbents at a higher HCl concentration. This observation is reasonable since Ca2+ ions are considered to be adsorbed on the crown ethers as the neutral species, CaCl2. At a given pH value, the K d value is much larger for the B18C6 resin than for the B15C5 resin, meaning that the Ca adsorption capacity of the former is much larger than the latter and consequently, the former is a much better Ca2+ ion adsorbent than the latter. At a given pH value, the K d value is slightly larger at a lower temperature for the two resins, which indicates that the adsorption of Ca2+ ion from the aqueous feed solution onto the resins is slightly exothermic.
Figure 1. Plots of K d as a function of HCl concentration in the feed solution at Ca2+ ion concentration of 0.05 mol dm−3. □, B18C6 resin at 25°C; ▪, B18C6 resin at 40°C; ○, B15C5 resin at 25°C; •, B15C5 resin at 40°C.
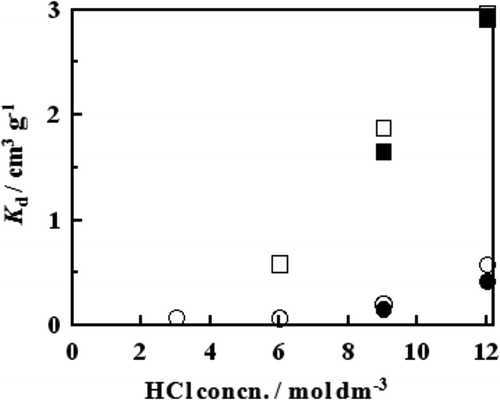
The K d value was plotted against the concentration of Ca2+ ion in the feed solution in . It is a decreasing function of the concentration of Ca2+ ion for both resins at the HCl concentrations of 9 and 12 mol dm−3. At a given Ca2+ ion concentration, the K d value is much larger for the B18C6 resin than for the B15C5 resin.
Figure 2. Plots of K d as a function of Ca2+ ion concentration in the feed solution at a given HCl concentration at 25°C. □, B18C6 resin at HCl concentration of 9 mol dm−3; ▪, B18C6 resin at HCl concentration of 12 mol dm−3; ○, B15C5 resin at HCl concentration of 9 mol dm−3; •, B15C5 resin at HCl concentration of 12 mol dm−3.
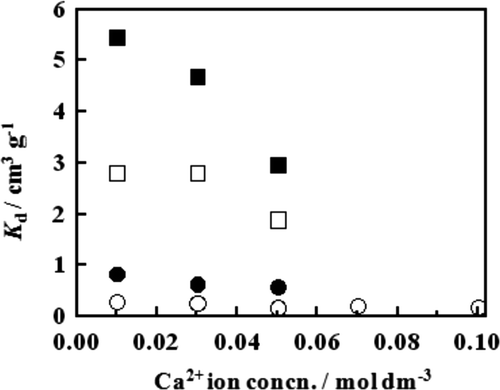
As a summary of the K d measurements, the B18C6 resin is a much better Ca2+ ion adsorbent than the B15C5 resin. This is most likely attributable to the difference in the hole size between the two crown ethers. While a B18C6 molecule with the hole (ring) size of 260 to 320 pm can accommodate the Ca2+ ion with the diameter of 228 pm in its hole, the hole size (170 to 220 pm) of a B15C5 molecule is slightly smaller than the diameter of the Ca2+ ion [Citation19]. A higher HCl concentration is preferable for a larger adsorption capacity for the Ca2+ ion, but temperature has only a slight effect.
3.2. Chromatograms and isotope accumulation curves
The results of the Ca isotope fractionation experiments, excluding the isotopic data, are summarized in . The total Ca2+ ion adsorption capacity, Q, of the resin column in each experiment was calculated by the equation
Breakthrough mode chromatograms for the experiments with the B18C6 resin are depicted in and 4. compares the chromatograms with varying HCl concentrations in the feed solution while the other experimental conditions were nearly kept constant (Runs Ca18-1, Ca18-2, Ca18-3, and Ca18-4). As expected from the results of K d measurements, Vp and V 1/2, and consequently, Q and Q/Vr , too, increase with increasing HCl concentration in the feed solution for a constant Ca2+ ion concentration in the feed solution and for a constant resin column height at a constant temperature. Figure 4 shows the temperature dependence of the chromatograms for a constant Ca2+ ion concentration in the feed solution and for a constant resin column height at a constant flow rate. The shapes of the chromatograms are seemingly nearly independent of temperature, but Vp and V 1/2, and consequently, Q and Q/Vr , too, slightly decrease with increasing temperature.
Figure 3. Chromatograms with the benzo-18-crown-6 ether (B18C6) resin with varying HCl concentration. —□—, Run Ca18-1; —•—, Run Ca18-2; —○—, Run Ca18-3; —▴—, Run Ca18-4.
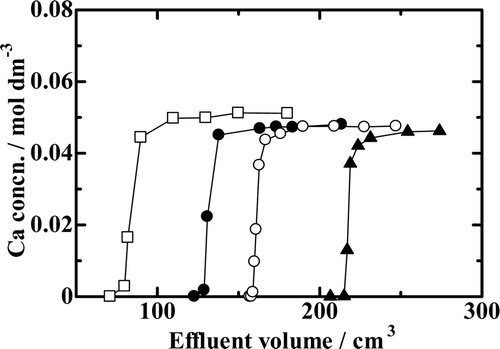
Figure 4. Chromatograms with the benzo-18-crown-6 ether (B18C6) resin at different temperatures and at different resin bed heights. —▵—, Run Ca18-2; —•—, Run Ca18-5; —□—, Run Ca18-6; —▾—, Run Ca18-7; —▴ — Run Ca18-8; —○—; Run Ca18-9; —▪—, Run Ca18-10.
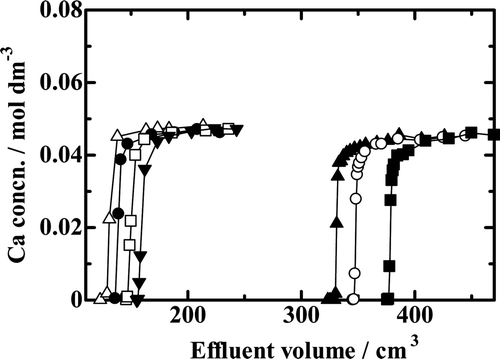
Breakthrough mode chromatograms for the experiments with the B15C5 resin are depicted in . The Vp and V 1/2 values are both larger at higher HCl concentration in the feed solution for a constant Ca2+ ion concentration in the feed and for a constant resin column height. The Vp and the V 1/2 values of Run Ca15-1 are equivalent to those of a chemical species like the Cl− ion that is not adsorbed onto the B15C5 resin at all, which means that no Ca2+ ions are adsorbed onto the B15C5 resin when HCl concentration is 4.5 mol dm−3 and lower.
Figure 5. Chromatograms with the benzo-15-crown-5 ether(B15C5) resin. —□—, Run Ca15-1; —○—, Run Ca15-2; —▴—, Run Ca15-3.
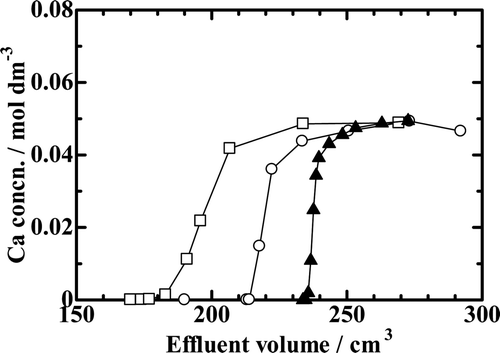
The results shown in to 5 are all consistent with the K d data shown in and .
Except for Runs Ca15-1 and Ca18-1 that showed practically no adsorption capacity for Ca2+ ions, the others were analyzed for their Ca isotopes. The results of the selected Runs in which five columns were used are shown in –. In each of those figures, the chromatogram is shown in the lower half of the figure and the isotopic data are given in the upper half. The isotopic data are plotted as local enrichment factor (LEF) of a fraction of the effluent defined as the x Ca/40Ca (x = 42, 43, 44 or 48) isotopic ratio of that fraction divided by the corresponding ratio of the feed solution:
Figure 6. Chromatogram and LEF profiles of Run Ca18-8. ▪, 42Ca/40Ca isotopic pair; ○, 43Ca/40Ca isotopic pair; □, 44Ca/40Ca isotopic pair; •, 48Ca/40Ca isotopic pair.
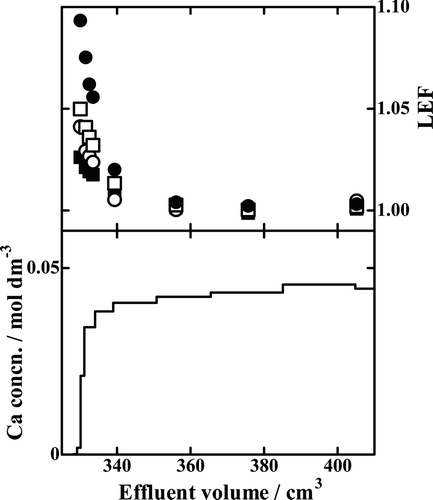
In to 8, the results with the B18C6 resin, Runs Ca18-8, Ca18-9, and Ca18-10, are drawn. The LEF value of an isotopic pair in general decreases monotonously with increasing effluent volume; it is generally the largest in the breakthrough-point fraction and asymptotically and promptly approaches unity with increasing effluent volume. The LEF values larger than unity mean the heavier isotopes are preferentially fractionated into the solution phase while the lighter ones remain in the crown ether phase. The degree of deviation of LEF from unity is larger for a larger value of x. That is, a larger mass difference between the isotopic pair results in larger Ca isotope effects.
The results of Run Ca15-2 using the B15C5 resin are depicted in . The shapes of the isotope accumulation profiles are roughly similar to those with the B18C6 resin. A close look at the plotted points, however, reveals that the LEF profiles, especially thoseof the 43Ca/40Ca and 48Ca/40Ca isotopic pairs, are significantly shifted from the expected smooth andmonotonously decreasing function of the effluent volume. We also note that the maximum degrees of isotope fractionation reached are much lower in than in to 8, which indicates that the B18C6 resin is a better Ca isotope separator than the B15C5 resin.
Figure 9. Chromatogram and LEF profiles of Run Ca15-2. ▪, 42Ca/40Ca isotopic pair; ○, 43Ca/40Ca isotopic pair; □, 44Ca/40Ca isotopic pair; •, 48Ca/40Ca isotopic pair.
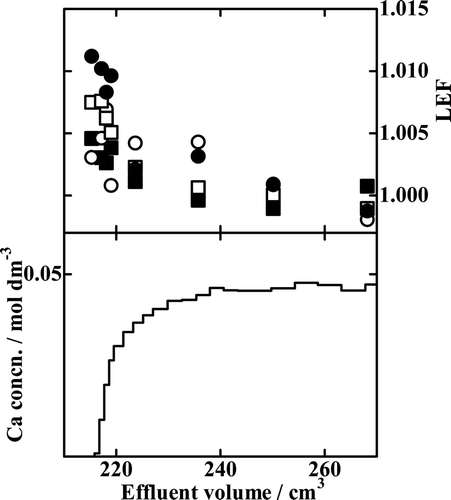
3.3. Evaluation of the magnitude of Ca isotope effects
To evaluate the magnitudes of the Ca isotope effects observed in the present study, the fractionation coefficient, ε = S − 1, was calculated. The single-stage separation factor, S, is defined as
In Equation (9), Ri and qi are the atomic fraction of the isotope of interest and the amount of Ca in the ith fraction of the effluent, respectively, R 0 is the atomic fraction of the isotope of interest in the feed solution, and the summation is taken over all the fractions where isotope fractionation is observed. The values of ε and ε per unit mass difference between the two isotopes, ε/ΔM, are listed in together with some other ε data obtained in chromatographic studies found in the literature [Citation5–Citation8,Citation10–Citation12]. The errors on the ε values are often large. The uncertainty in the value of ε originates mostly from Ri − R 0 in Equation (9) since this difference is usually very close to zero. We could not determine the ε values for the 43Ca/40Ca and 48Ca/40Ca isotopic pairs of Run Ca15-2 due to the too large experimental uncertainties.
Table 2. Fractionation coefficients obtained in this work and in some previous chromatographic works.
The findings in as well as in to 9 may be summarized as follows:
1. | In the present study, the heavier isotopes are preferentially fractionated into the solution phase, while the lighter ones are fractionated into the crown ether phase in both the B18C6 and B15C5 resin systems. The direction of this Ca isotope fractionation is common to all the systems listed. | ||||
2. | The values of ε on the order of 10−3 and those of ε/Δ M on the order of 10−4 were obtained with the B18C6 resin; they are on the same order as those of previous studies with the same resin [Citation11,Citation12] and for 18-crown-6 resin [Citation10], but larger than those of studies with ion exchange resins [Citation5,Citation6]. The cryptand systems [Citation7,Citation8] yielded larger ε values than crown ether systems. However, we note that, while the cryptand systems were basically operated in organic solvent media, our experiments were all carried out in aqueous solutions, which is a very large advantage obtained using the present silica-based resins. | ||||
3. | The ε and ε/ΔM values with the B15C5 resin, which have very large errors, are one order of magnitude smaller than the corresponding values obtained with the B18C6 resin. | ||||
4. | Admittedly roughly, we can say the ε value seems nearly proportional to the isotopic mass difference, ΔM, i.e. the ε/Δ M value is nearly constant in a given Run. This indicates that the present Ca isotope effects are solely mass dependent ones and are little affected by nuclear size and shape effects (nuclear field shift effects) [Citation21]. The data supporting the present mass dependent Ca isotope effects can be provided by three-isotope plots. An example is shown in where the LEF value minus one for the x Ca/40Ca isotopic pair (x = 43, 44 or 48) of Run Ca18-8 is plotted against that for the 42Ca/40Ca isotopic pair. The slopes of the curves were calculated to be 1.44, 1.90, and 3.44 for the x Ca/40Ca isotopic pair (x = 43, 44, or 48), respectively. The corresponding theoretical values, estimated on the basis that the observed isotope effects arise solely from molecular vibration, are 1.47, 1.91, and 3.50, respectively. The smallness of the deviation of the experimental slope from the theoretical one supports our conclusion that the isotope effects in the present systems are normal mass dependent ones. | ||||
5. | In general, a higher HCl concentration in the feed solution yields a smaller ε value both in the B18C6 resin system (Runs Ca18-2, Ca18-3, and Ca18-4) and in the B15C5 resin system (Runs Ca15-2 and Ca15-3) at a given temperature. The degree of HCl concentration dependence ofthe ε value is more substantial with the B15C5 resin than with the B18C6 resin. We previously observed a similar HCl concentration dependence of the ε value in chromatographic Sr isotope fractionation experiments with the B15C5 resin [Citation13]. | ||||
6. | Contrary to our expectation, the temperature dependence of the ε value is not clear (Runs Ca18-2, Ca18-5, Ca18-6, Ca18-7 and Runs Ca18-8, Ca18-9, Ca18-10). The temperature range may be too narrow for the temperature dependence of the ε value to show up clearly. |
3.4. MO calculations
Our major purpose for the present MO calculations was to elucidate the findings (1), (3), and (5) above. For all the Ca species considered, no imaginary frequency was obtained, which means that the optimized structures are all at the global or local minimum of the potential energy surface. Electronic energies corrected for the zero point energies are summarized in for some Ca species considered.
Table 3. Comparison of energies of Ca species.
To determine the hydration number of the aqueous Ca2+ ion, we first optimized the structures of [Ca2+(H2O)6](H2O) (i.e. six H2O molecules in the primary hydration sphere and one in the secondary hydration sphere) and [Ca2+(H2O)7] (i.e. seven H2O molecules in the primary hydration sphere), and compared the energies at the optimized structures. Asa result, [Ca2+(H2O)6](H2O) was found to be more stable by 17.9 kJ mol−1 than [Ca2+(H2O)7], as shown in . We thus regarded [Ca2+(H2O)6] as the simply hydrated Ca species in aqueous HCl solution. The hydration number of 6 is within the range of 5 to 13 reported as hydration number of aqueous Ca2+ ion [Citation22]. The optimized structure of [Ca2+(H2O)6] is shown in .
In Ca2+ ion-bearing aqueous HCl solution, part of the Ca2+ ions form complex species with Cl− ions,
The stability constant of Equation (10) is 1.2 at theionic strength of 0.7 mol dm−3 and 25°C [Citation23]. Applying this value for the present systems with HCl concentrations of 9 mol dm−3 and Ca2+ ion concentration of 0.05 mol dm−3, the percentage of the complex species is calculated to be 91.6%. Since the ionic strength in our chromatographic experiments was 9 to 12 mol dm−3, quite different from 0.7 mol dm−3, this value is not directly applicable to the present systems. It must be true, however, that the majority of Ca2+ ions in our chromatographic experiments are in the complex form with Cl− ions, CaCl+(aq), and the proportion of the complex form is higher at a higher HCl concentration.
The optimized structures of [B18C6 + CaCl2], [B18C6 + CaCl2 + HCl] and [B18C6 + CaCl2 + 2HCl], the model species expected for the crown ether phase ofthe B18C6 system, are shown in –c, respectively. In each of these structures, the six oxygen atoms of the B18C6 molecule and the Ca2+ ion are nearly coplanar, since the size of the Ca2+ ion fits that of the hole (ring) formed by the six oxygen atoms [Citation19]. For [B18C6 + CaCl2], we considered two conformers, Conformer I in which the two Cl− ions of CaCl2 are bonded to the Ca2+ ion from one side of the plane, and the other (Conformer II) in which one of the two Cl− ions is bonded to the Ca2+ ion from one side of the plane and another Cl− ion from the other side of the plane. Calculations showed that Conformer II is more stable by 54.8 kJ mol−1 than Conformer I, and so, we regarded Conformer II as [B18C6 + CaCl2], which is depicted in a. In [B18C6 + CaCl2 + HCl], the HCl molecule interacts with a Cl− ion of CaCl2 through its H atom. In [B18C6 + CaCl2 + 2HCl], each of the two HCl molecules interacts with each of the two Cl− ions of CaCl2. Although no experimental data exist, we expect the proportion of [B18C6 + CaCl2 + HCl] and [B18C6 + CaCl2 + 2HCl] increases with increasing HCl concentration.
Figure 12. The optimized structures of (a) [B18C6 + CaCl2], (b) [B18C6 + CaCl2 + HCl] and (c) [B18C6 + CaCl2 + 2HCl].
![Figure 12. The optimized structures of (a) [B18C6 + CaCl2], (b) [B18C6 + CaCl2 + HCl] and (c) [B18C6 + CaCl2 + 2HCl].](/cms/asset/90de32e2-ade3-4f66-b732-390bc4664cfb/tnst_a_669245_o_f0012g.jpg)
The optimized structures of [B15C5 + CaCl2], [B15C5 + CaCl2 + HCl], and [B15C5 + CaCl2 + 2HCl], the model species expected for the crown ether phase ofthe B15C5 system, are shown in a–c, respectively. In each of these structures, the five oxygen atoms of the B15C5 molecule are nearly coplanar and the Ca2+ ion is located slightly above the plane. This is because the size of a Ca2+ ion is larger than that of the hole (ring) formed by the five oxygen atoms of the B15C5 molecule [Citation19]. For [B15C5 + CaCl2], we considered two conformers, Conformer I in which the two Cl− ions of CaCl2 are bonded to the Ca2+ ion from the same side of the plane as the Ca2+ ion is located and Conformer II in which one of the two Cl− ions is bonded to the Ca2+ ion from one side of the plane and another Cl− ion from the other side of the plane. Contrastively to the case of [B18C6 + CaCl2], Conformer I is more stable by 8.6 kJ mol−1 than Conformer II, and so, we decided to regard Conformer I as [B15C5 + CaCl2], which is depicted in a. As in the case of [B18C6 + CaCl2 + HCl] and [B18C6 + CaCl2 + 2HCl], the HCl molecule interacts with a Cl− ion of CaCl2 through its H atom in [B15C5 + CaCl2 + HCl], and each of the two HCl molecules interacts with each of the two Cl− ions of CaCl2 in [B15C5 + CaCl2 + 2HCl]. Although no experimental data exist, we expectthe proportion of [B15C5 + CaCl2 + HCl] and [B15C5 + CaCl2 + 2HCl] increases with increasing HCl concentration.
Figure 13. The optimized structures of (a) [B15C5 + CaCl2], (b) [B15C5 + CaCl2 + HCl] and (c) [B15C5 + CaCl2 + 2HCl].
![Figure 13. The optimized structures of (a) [B15C5 + CaCl2], (b) [B15C5 + CaCl2 + HCl] and (c) [B15C5 + CaCl2 + 2HCl].](/cms/asset/5c2f0996-dbb4-4e65-8bdb-f2b8a8488981/tnst_a_669245_o_f0013g.jpg)
The rpfrs for the 48Ca/40Ca isotopic pair of the considered Ca species at 50°C are summarized in . First of all, we notice that the rpfrs for the species in the solution phase ([Ca2+(H2O)6] and [Ca2+(H2O)6Cl−]) are larger than those for the species in the crown ether phases ([B18C6 + CaCl2], [B18C6 + CaCl2 + HCl] and [B18C6 + CaCl2 + 2HCl] in theB18C6 system and [B15C5 + CaCl2], [B15C5 + CaCl2 + HCl], and [B15C5 + CaCl2 + 2HCl] in the B15C5 system). This means that the sum of the forces acting on the Ca2+ ion in the solution phase is larger than the sum in the crown ether phases, and as a consequence, the heavier Ca isotopes are preferentially fractionated into the aqueous solution phase and the lighter ones into the crown ether phases in both the B18C6 and the B15C5 systems if the aqueous and crown ether phases are in equilibrium with each other concerning Ca isotopes [Citation15]. The MO results are thus consistent with the results of the chromatographic Ca isotope fractionation experiments (finding (1) in Section 3.3). In, we also listed the rpfr value of Ca2+(H2O)7, a higher energy model of the aqueous Ca2+ ion; its value is larger than those of the species inthe crown ether phases. Thus, even if we regard Ca2+(H2O)7 as the aqueous Ca2+ ion in the solution phase, the qualitative discussion above does not change at all.
Table 4. The values of the reduced partition function ratios for the 48Ca/40Ca isotopic pair at 50°C.
We also notice in that the rpfrs of the Ca species in the crown ether phase of the B15C5 system are larger than those of the B18C6 system, from which we can expect that, under similar experimental conditions, the Ca isotopic fractionation coefficient is larger for the B18C6 system than for the B15C5 system. This also qualitatively agrees with the present experimental results (finding (3) in Section 3.3.).
The rpfr of [Ca2+(H2O)6] is larger than that of [Ca2+(H2O)6Cl−]; the former minus the latter is 1.02522 − 1.02325 = 0.00197. This indicates that the rpfr value of the solution phase decreases with increasing HCl concentration since the proportion of [Ca2+(H2O)6Cl−] is expected to be higher at a higher HCl concentration. The decreasing order of the rpfrs of the species in the crown ether phase of the C18B6 system is: [B18C6 + CaCl2] > [B18C6 + CaCl2 + HCl] > [B18C6 + CaCl2 + 2HCl]; the differences between the neighboring values are 0.00038 and 0.00022, respectively. This indicates that, like the rpfr of the resin phase of the B18C6 system, the rpfr of the solution phase also decreases with increasing HCl concentration. However, comparing the value 0.00197 with 0.00038 and 0.00022, the dependence of the rpfr value on the HCl concentration is expected to be much more significant in the solution phase than in the crown ether phase. Thus, the present MO calculations indicate that the ε value decreases with increasing HCl concentration, which is in qualitative agreement with the experiments (finding (5) in Section3.3.).
The decreasing order of the rpfrs of the species in the crown ether phase of the C15B5 system is: [B15C5 + CaCl2 + 2HCl] > [B15C5 + CaCl2 + HCl] > B15C5 + CaCl2]. This means that, contrastively to the B18C6 system, the existence of HCl in the crown ether phase enhances the rpfr of that phase in the B15C5 system; the rpfr of the crown ether phase is an increasing function of HCl concentration. Coupled with its effect on the rpfr of the solution phase, the increase in HCl concentration decreases the ε value in the B15C5 system, probably in a more significant way than in the B18C6 system. This is again consistent with the experiments (finding (5) in Section 3.3.).
As a summary of the MO calculations above, if weassume the existence of such Ca species as [Ca2+(H2O)6], [Ca2+(H2O)6Cl−], [B18C6 + CaCl2], [B18C6 + CaCl2 + HCl], [B18C6 + CaCl2 + 2HCl], [B15C5 + CaCl2], [B15C5 + CaCl2 + HCl] and [B15C5 + CaCl2 + 2HCl], most of the trends in the Ca isotope effects observed in the present chromatographic experiments are, albeit in a qualitative fashion, explainable.
In additional MO calculations, we examined effects of the existence of H2O molecules in the crown ether phases. The results are also included in . Twoclusters, [B18C6 + CaCl2 + H2O] and [B15C5 + CaCl2 + 2HCl + H2O] were considered as examples. In the optimized structure of [B18C6 + CaCl2 + H2O], the H2O molecule was H-bonded to a Cl− ion of CaCl2, and it was H-bonded to the Cl− ion of an HCl molecule in [B15C5 + CaCl2 + 2HCl + H2O]. In both the cases, the rpfr value of the cluster with a H2O molecule is slightly smaller than that of corresponding cluster without it, which indicates that the presence of H2O molecules slightly decreases the rpfr values in the crown ether phases.
4. Conclusion
The following statements summarize our present study.
1. | Heavier isotopes of calcium were enriched in the frontal part of the chromatograms obtained in the chromatographic experiments operated in the breakthrough mode in which the benzo-18-crown-6 ether resin synthesized in the inner pores of highly porous silica beads functioned as the absorbent of calcium ions. A similar tendency in calcium isotope fractionation was observed in the chromatographic experiments with the benzo-15-crown-5 ether resin. The fractionation coefficient for the given isotopic pair, x Ca/40Ca (x = 42, 43, 44, or 48), was larger with the benzo-18-crown-6 ether resin than with benzo-15-crown-5 ether resin, which was consistent with the molecular orbital calculations on the calcium isotopic reduced partition function ratios of the calcium species assumed to be present in the solution and crown ether phases of the chromatographic experiments. The calcium species assumed for the calculations were a simply hydrated calcium ion and an aqueous calcium ion directly interacting with a chloride ion in the solution phase, and those in the crown ether phase were calcium chloride interacting with a crown ether molecule (benzo-18-crown-6 ether or benzo-15-crown-5 ether) accompanied by zero, one or two hydrochloric acid molecules. | ||||
2. | The observed calcium isotope effects were most probably mass dependent; the values of the fractionation coefficients per unit mass difference between two calcium isotopes were nearly constant, albeit sometimes with large experimental uncertainties, and the slopes of the three-isotope plot curves were very close to the theoretical values that were inferred from the isotope effectsbased solely on the molecular vibration. | ||||
3. | A higher hydrochloric acid concentration in the feed solution resulted in a smaller value of the fractionation coefficient in both the benzo-18-crown-6 ether and the benzo-15-crown-5 ether systems. Support for this was provided by the results of the molecular orbital calculations in aqualitative fashion. |
References
- Kishimoto , T. , Ogawa , I. , Umehara , S. and Hirano , Y. 2007 . Double beta decay and CANDLES test [in Japanese] . Genshikaku Kenkyu , 51 : 36 – 48 .
- Reeve , J. , Arlot , M. , Bernat , M. , Charhon , S. , Edouard , C. , Slovik , D. , Vismans , F.J.F.E. and Meunier , P.J. 1981 . Calcium-47 kinetic measurements of bone turnover compared to bone histomorphometry in osteoporosis: The influence of human parathyroid fragment (hPTH 1-34) therapy . Metab. Bone Dis. Rel. Res , 3 : 23 – 30 .
- Zucker , D. and Drury , J.S. 1964 . Separation of calcium isotopes in an amalgam system . J. Chem. Phys , 41 : 1678 – 1681 .
- Saito , S. , Yanase , S. and Oi , T. 2011 . Calcium isotope effects accompanying electrochemical insertion of calcium intographite from electrolyte solution . Radioisotopes , 60 : 265 – 274 .
- Oi , T. , Morioka , N. , Ogino , H. , Kakihana , H. and Hosoe , M. 1993 . Fractionation of calcium isotopes in cation-exchange chromatography . Sep. Sci. Technol , 28 : 1971 – 1983 .
- Jepson , B.E. and Shochey , G.C. 1984 . Calcium hydroxide isotope effects in calcium isotope enrichment by ion exchange . Sep. Sci. Technol , 19 : 173 – 181 .
- Heumann , K.G. and Schiefer , H. 1980 . Calcium isotope separation on an exchange resin having cryptand anchor groups . Angew. Chem. Int. Ed. Engl , 19 : 406 – 407 .
- Heumann , K.G. , Schiefer , H.P. and Spiess , W. 1982 . “ Enrichment of heavy calcium isotopes by ion exchanger resins with cyclopolyethers as anchor groups, in ” . In Stable Isotopes Edited by: Schmidt , H.-L. , Förstel , H. and Heinzinger , K. 711 – 718 . Elsevier , , Amsterdam
- Jepson , B.E. and Evans , W.F. 1990 . Chromatographic separation of calcium isotopes with polymer-bound ligands . Sep. Sci. Technol , 25 : 1893 – 1908 .
- Jepson , B.E. 1992 . Calcium isotope separation by chemical exchange with polymer-bound crown compound . Bull. Res. Lab. Nucl. Reactors Special Issue , 1 : 307 – 312 .
- Hayasaka , K. , Kaneshiki , T. , Nomura , M. , Suzuki , T. and Fujii , Y. 2008 . Calcium ion selectivity and isotope effects studied by using bwnzo–18-crown-6 resins . Prog. Nucl. Energy , 50 : 510 – 513 .
- Fujii , Y. , Nomura , M. , Kaneshiki , T. , Sakuma , Y. , Suzuki , T. , Umehara , S. and Kishimoto , T. 2010 . Mass dependence of calcium isotope fractionations in crown-ether resin chromatography . Isotopes Environ. Health Stud , 46 : 233 – 241 .
- Fukuda , Y. , Zhang , Y.-H , Nomura , M. , Suzuki , T. , Fujii , Y. and Oi , T. 2010 . Strontium isotope effects observed in liquid chromatography with crown ether resins . J.Nucl. Sci. Technol , 47 : 176 – 183 .
- Otake , K. , Suzuki , T. , Kim , H.-J. , Nomura , M. and Fujii , Y. 2006 . Novel synthesis method of phenol type benzo-15-crown-5 ether resin and its application for lithium isotope separation . J. Nucl. Sci. Technol , 43 : 419 – 422 .
- Bigeleisen , J. and Mayer , M.G. 1947 . Calculation of equilibrium constants for isotopic exchange reactions . J.Chem. Phys , 15 : 261 – 267 .
- Shibahara , Y. , Takaishi , H. , Nishizawa , K. and Fujii , T. 2002 . Strontium isotope effects in liquid exchange reaction . J.Nucl. Sci. Technol , 39 : 451 – 456 .
- Frisch , M.J. , Trucks , G.W. and Schlegel, G.E. Scuseria, M.A. Robb, J.R. Cheeseman, J.A. Montgomery, Jr., T. Vreven, K.N. Kudin, J.C. Burant, J.M. Millam, S.S. Iyengar, J. Tomasi, V. Barone, B. Mennucci, M. Cossi, G. Scalmani, N. Rega, G.A. Petersson, H. Nakatsuji, M. Hada, M. Ehara, K. Toyota, R. Fukuda, J. Hasegawa, M. Ishida, T. Nakajima, Y. Honda, O. Kitao, H. Nakai, M. Klene, X. Li, J. E. Knox, H.P. Hratchian, J.B. Cross, V. Bakken, C. Adamo, J. Jaramillo, R. Gomperts, R.E. Stratmann, O. Yazyev, A.J. Austin, R. Cammi, C. Pomelli, J.W. Ochterski, P.Y. Ayala, K. Morokuma, G.A. Voth, P. Salvador, J.J. Dannenberg, V.G. Zakrzewski, S. Dapprich, A.D. Daniels, M.C. Strain, O. Farkas, D.K. Malick, A.D. Rabuck, K. Raghavachari, J.B. Foresman, J.V. Ortiz, Q. Cui, A.G. Baboul, S. Clifford, J. Cioslowski, B.B. Stefanov, G. Liu, A. Liashenko, P. Piskorz, I. Komaromi, R.L. Martin, D.J. Fox, T. Keith, M.A. Al-Laham, C.Y. Peng, A. Nanayakkara, M. Challacombe, P.M.W. Gill, B. Johnson, W. Chen, M.W. Wong, C. Gonzalez, and J.A. Pople, Gaussian 03, Revision D.02, Gaussian, Inc., Wallingford CT , H.B. 2004 .
- Oi , T. 2000 . Calculations of reduced partition function ratios of monomeric and dimeric boric acids and borates by the ab initio molecular orbital theory . J. Nucl. Sci. Technol , 37 : 166 – 172 .
- Greenwood , N.N. and Earnshaw , A. 1984 . Chemistry of the Elements , 107 Oxford : Pergamon Press .
- Kakihana , H. and Kanzaki , T. 1969 . A simplified and generalized method for analyzing chromatographic isotope separation data . Bull. Tokyo Inst. Technol , 90 : 77 – 89 .
- Bigeleisen , J. 1996 . Nuclear size and shape effects in chemical reactions. Isotope chemistry of the heavy elements . J.Am. Chem. Soc , 118 : 3676 – 3680 .
- Zavitsas , A.A. 2005 . Aqueous solutions of calcium ions: Hydration numbers and the effect of temperature . J.Phys. Chem. B , 109 : 20636 – 20640 .
- Martell , A. and Sillen , L.G. 1984 . Stability Constants of Metal-ion Complexes , 418 London : The Chemical Society .