Abstract
The hydrogen isotope exchange reaction between HTO vapor and each amino acid has been observed in order to establish a method of estimation of the internal exposure of organically bound tritium in a heterogeneous system at 25∼70°C. Rate constants (k) for the amino acids have been obtained by applying the A″-McKay plot method. Using these k values, Arrhenius plots for both the COOH and NH2 groups are drawn, and linearity was obtained over the range of 25∼70°C. Comparing the rate constants, the following four statements can be made regarding the T-for-H exchange reaction. (1) The reactivity of the functional groups in amino acids increases with increasing temperature. (2) Applying Taft's equation, the ratio of polar effect to steric effect is 2:8 in the COOH group and 9:1 in the NH2 group at 25°C. (3) The A″-McKay plot method is useful for studying the reactivity of materials, not only with one (or the same kind of) functional group(s) but also with two different kinds of functional groups. (4) The method used in this work may be useful to investigate the behavior of organically bound tritium, quantitatively.
1. Introduction
The external exposure from tritium (T) can be neglected because the maximum energy of the emitted β −-ray is very low (18.6 keV). In the case of tritiated-water (HTO), T can be incorporated into the body and behaves as free water tritium (FWT) [Citation1]. T also causes a hydrogen isotope exchange reaction [Citation2] (T-for-H exchange reaction) that can be divided into exchangeable organically bound tritium (OBT) and non-exchangeable OBT [Citation3]. Exchangeable OBT behaves as FWT in the body, and the dose coefficient of T in FWT is unity. On the other hand, the dose coefficient of T in non-exchangeable OBT is 2.3 [Citation4]. This is a key distinction that makes the study on the internal exposure of T and is very important. The T concentration in the atmosphere will increase as more reprocessing plants for spent nuclear fuel come online and nuclear fusion research continues. Thus, the study on exchangeable OBT and non-exchangeable OBT is more important.
Previously [Citation5], we have studied the T-for-H exchange reaction over the range of 50∼70°C using the A″-McKay plot method, and the rate constants (k) of the materials were obtained. We also have estimated the reactivity of functional groups using k. Considering the evaluation of internal exposure, it is found that observing the T-for-H exchange reaction under the biological temperature (≈ 36°C) in a heterogeneous system is necessary [Citation6].
Taking into account the above background information, the T-for-H exchange reaction (gas–solid reaction) between HTO vapor and each amino acid having two different kinds of functional groups (i.e. COOH and NH2 groups) is observed at 36°C and 25°C. The observed data were applied to the A″-McKay plot method to obtain the k for the functional groups in each amino acid. Each Taft plot was also drawn by using these k values. Consequently, the ratio of the polar effect to the steric effect in the reaction can be estimated. Observing the reaction at various temperatures, the temperature dependence is clarified. From these experiments, the effect of T on the human body can be estimated.
2. Theoretical background
As mentioned in previous literature [Citation6–Citation11], the A″-McKay plot is described by the following equation.
This method has three characteristics: (1) The value of the slope equals k when the plot of −log10[1 − x/x ∞(F)] vs. t is drawn. (2) k can be also obtained in a heterogeneous system. (3) It is possible to study the reactivity of the materials not only having one (or the same kind of) functional group(s), but having three different kinds of functional groups.
3. Experiment
Using a vacuum line [Citation12], each T-for-H exchange reaction was observed between HTO vapor and several amino acids at 25, 36, 50, 60, or 70°C. Each solid sample was sufficiently powdered in an agate mortar, then dried and sieved to obtain a grain size between 53 and 75 μ m in diameter [Citation12]. For each reaction run, both 7.22 mg of HTO water as a gas sample (whose specific activity was 8 × 105 Bq g−1) and 15.0 mg of each solid material were used. The method of observation was the same as previously reported [Citation12]. After the reaction, the solid sample was dissolved in 3.00 ml of water, and the activity of 1.00 ml of the solution was measured with a liquid scintillation counter. Because the half-life of T is 12.3 years, the activity was corrected by a calculation every three months.
In order to apply the obtained data to the A″-McKay plot method in the gas–solid reaction, the excess experiment [Citation13,Citation14] was performed. From the experiment, it is found that the total number of 1H atoms and T atoms in this HTO vapor are much larger than the total number of H atoms at the solid sample surface contributing to the reaction. In other words, we changed the mass of the solid samples in order to use the analysis method of pseudo first order reaction.
Based on the two previous reports [Citation15,Citation16], 1H atoms directly bonded to the carbon atom cannot participate in the reaction. Accordingly, it is found that the exchange 1H atoms are the only 1H atoms in the COOH and NH2 groups under the conditions in this work.
4. Results and discussion
4.1. Applicability of the A″-McKay plot method
Based on the former section, the excess experiment was carried out. If the excess condition is satisfied, total activity of each solid sample material is proportional to the mass in the reaction. shows the excess experiment of the l-aminobutylic acid at 70°C. This figure shows a linear relationship between the activity and mass. That is, the number of reactions per unit is constant in the mass range of 5∼60 mg and the quantity of the HTO vapor is much in excess to the l-aminobutylic acid. The results obtained by using other sample materials were similar to that of the amino acid.
4.2. Reactivity of each material
The T-for-H exchange reaction between HTO vapor and each amino acid was observed. The relation between reaction time and the specific activity of l-aminobutylic acid at 25°C was drawn (). It is found that the reaction occurred because the specific activity of T increased with time. From the previous report [Citation17], it can be assumed that the reactivity of the COOH group is larger than that of the NH2 group in the same molecule. Therefore, it is found that the three following reactions occurred in this figure.
Figure 2. T specific activity vs. time for the reaction between l-aminobutyric acid and HTO vapor. Reaction temp: 25°C.
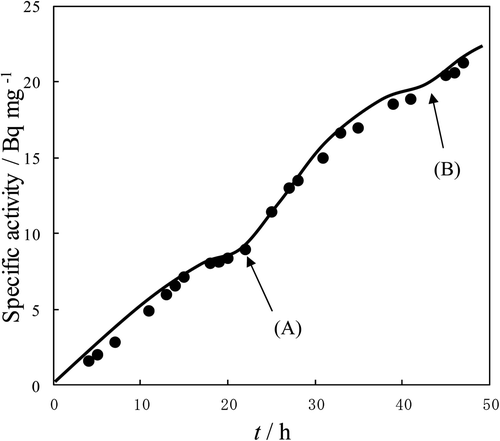
Part (1): From the origin to (A)
Both the COOH group and NH2 group at the surface of l-aminobutylic acid react with HTO vapor, and a reaction at the surface of the COOH group reaches equilibrium at (A).
Part (2): From (A) to (B)
Only the NH2 group at the surface of l-aminobutylic acid reacts with HTO vapor and a reaction at the surface of the NH2 group reaches equilibrium at (B).
Part (3): After (B)
T on the solid surface diffuses to the inside.
4.3. Reactivity of COOH and NH2 groups in each material
In , the data obtained from Part (1) was applied to the A″-McKay plot method to obtain the rate constant (k NH2) [Citation18]. shows the A″-McKay plot of the NH2 group, and the slope of the straight line of the NH2 group is equal to k NH2. In order to obtain the rate constant (k COOH), the reaction value of the NH2 group in Part (1) was calculated from the broken line. Then the reaction value of the NH2 group thus obtained was subtracted from the total reaction value. Each data thus obtained was applied to the A″-McKay plot method in order to obtain k COOH. The slope of the straight line of the COOH group in is equal to k COOH. For other materials, the values of k for the COOH and NH2 groups are shown in . The table shows that the reactivity of each group increases with increasing temperature. In this way, the A″-McKay plot method is useful to analyze two steps of a simultaneous reaction.
Figure 3. A″-McKay plots for l-aminobutyric acid in the reaction at 25°C. ▴: COOH group, •: NH2 group.
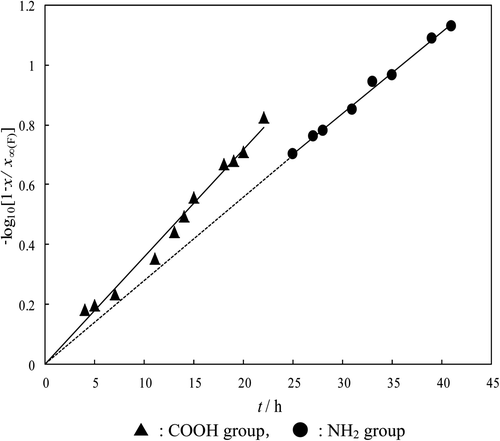
Table 1. Rate constants (k) for COOH and NH2 groups in each sample material and activation energy (E a).
Using these k values, Arrhenius plots for both the COOH and NH2 groups are shown in . The correlation coefficients of the straight lines in the figure satisfy the condition of significance at the 1% level. As previously reported [Citation18], linearity was seen in the range of 50∼70°C. However, such linearity was not obtained at low temperature. In this work, linearity was obtained over the range of 25∼70°C, that is, the reactivity at biological temperature can be clarified. In addition, activation energies (E a) for both COOH and NH2 groups were obtained from and shown in .
4.4. Applicability of Taft's equation
In order to quantitatively estimate the reactivity, we applied the k values to Taft's equation [19]. Taft's equation is generally expressed as follows:
In this work, k 0 is the rate constant for glycine; k is a rate constant for each amino acid; ρ* equals the reaction constant for amino acids; σ* equals the polar substituent constant; E s equals the steric substituent constant; δ is a measure of the steric effect. The following two operations were carried out: (1) the value of ρ* was changed from 0.0 to 1.0 at 0.1 intervals; (2) inversely, δ was changed from 1.0 to 0.0 at 0.1 intervals. As a result, 11 figures for the COOH group (or the NH2 group) can be obtained at each temperature. One line was obtained in each figure, and the line having the largest correlation coefficient out of the 11 lines was selected as the ratio of ρ* to δ for the functional group at each temperature. These ratios for the COOH and NH2 groups at 25°C, 36°C, and 50°C are shown in , and the plots having the largest correlation coefficient for COOH and NH2 groups at 25°C are shown in and . It is fairly certain that (1) the reactivity of amino acids follows Taft's equation in gas–solid reaction, (2) the COOH group is strongly influenced by the steric effect, and (3) the NH2 group is strongly influenced by the polar effect. Consequently, the effect of “the ratio of the polar effect to the steric effect” on the reactivity of the materials has been clarified at each temperature.
Figure 5. A plot (based on Taft's equation) of COOH group for each amino acid. Reaction temp: 25°C. ×: l-aminobutyric acid (obtained in this work), *: l-valine (obtained in this work), □: l-2-phenylglycine, ⋄: l-phenylalanine, ▵: l-α-alanine, ○: l-norvaline.
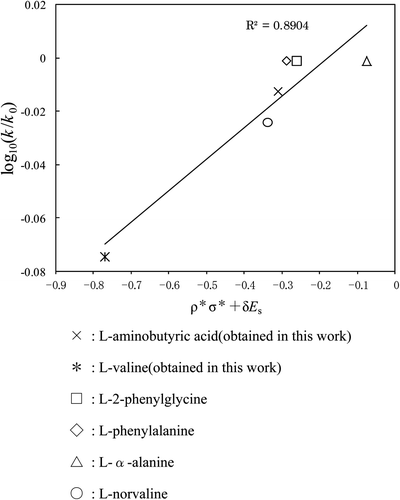
Figure 6. A plot (based on Taft's equation) of NH2 group for each amino acid. Reaction temp: 25°C. ×: l-aminobutyric acid, *: l-valine, ▪: l-2-phenylglycine, ♦: l-phenylalanine, ▴: l-α-alanine, •: l-norvaline.
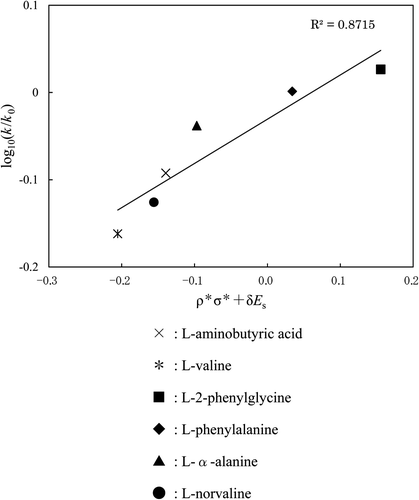
Table 2. The ratio of the reaction constant (ρ*) to the steric effect (δ).
4.5. Comparison of the reactivity of NH2 group in each amino acid
Comparing the structures of the various amino acids having two functional groups (COOH and NH2), the number of carbon or benzene rings is different in l-glycine as shown . Based on Taft's equation mentioned in the former section, the NH2 group can be considered as having some polar effect. The CH3 group is an electron-donative substituent (−I effect [Citation18]) and the effect increases with increasing carbon chain. If electron density of the functional group becomes large, the reactivity generally diminishes. Therefore, the reactivity of the NH2 group of l-norvaline diminishes because it has the longest carbon chains in the sample materials. In addition, by comparison between l-norvaline and l-valine, it is found that the reactivity tends to diminish by existing carbon near the functional group. However, the C6H5 group is an electron attractive substituent (+I effect). So, electron density of the functional group diminishes, and the reactivity of the NH2 group of l-phenylalanine or l-phenylglycine is larger than that of l-glycine.
On the other hand, the COOH group is also influenced by the steric effect. Based on the influence of steric effect of the substituent, the reactivity of the COOH group is diminished. Because the +I effect of the benzene ring is larger than the −I effect of CH3 group, the reactivity of l-phenylalanine or l-phenylglycine is similar to that of l-glycine (in spite of having the polar effect). That is, the reactivity of the COOH group diminishes because the volume of the substituent becomes large.
5. Conclusions
The T-for-H exchange reaction (gas–solid reaction) between HTO vapor and each amino acid was observed and the reactivity of the amino acid was quantitatively analyzed. The Arrhenius plots showed good linear behavior over the range of 25∼70°C. Based on these results we can make the following statements. (1) The reactivity at biological temperature was clarified. (2) Applying Taft's equation, the ratio of polar effect to steric effect was clarified at each temperature (i.e. 25°C and 36°C). (3) The method used in this work can quantitatively clarify the reactivity of the T-for-H exchange reaction, and can be useful to estimate the internal exposure of exchangeable OBT.
References
- Choi , Y.H. , Lim , K.M. , Lee , W.Y. , Diabaté , S. and Strack , S. 2002 . Tissue free water tritium and organically bound tritium in the rice plant acutely exposed to atmospheric HTO vapor under semi-outdoor conditions . J. Environ. Radioact , 58 : 67 – 85 .
- Hisamitsu , S. and Takizawa , Y. 1995 . Tritium transfer from diet to human . J. Radioanal. Nucl. Chem , 197 : 271 – 280 .
- Diabate , S. and Strack , S. 1993 . Organically Bound Tritium . Health. Phys , 65 : 698 – 712 .
- Okada , S. and Momoshima , N. 1993 . Overview of tritium: characteristics, sources, and problems . Health. Phys , 65 : 595 – 609 .
- Imaizumi , H. , Muramatsu , K. and Endo , T. 1993 . Effect of degree of polymerization of PVA on THE Reactivity of aliphatic (or aromatic) compounds in hydrogen-isotope exchange reaction . Radiochim. Acta , 61 : 53 – 56 .
- Okada , M. , Imaizumi , H. , Satoh , H. and Kobayashi , K. 1985 . Comparison of poly(vinyl alcohol)with dicarboxylic acids for acidity applying isotope exchange reaction using tritiated water vapor . Radiochim. Acta , 38 : 49 – 52 .
- Okada , M. , Imaizumi , H. , Kobayashi , K. and H. 1986 . Satoh, Effect of the degree of polymerization of poly (vinyl alcohol) (PVA) on the reactivity in the hydrogen-isotope-exchange reaction between PVA and HTO . Radioisotopes , 35 : 9 – 14 .
- Okada , M. , Imaizumi , H. and Sugawara , J. 1991 . Measurement method of “Acidity Based on Kinetic Logic” by applying hydrogen-isotope exchange reaction in a heterogeneous system . Anal. Sci , 7 : 677 – 678 .
- Imaizumi , H. and Watanabe , E. 1994 . Comparison of toluenethiols in reactivity using hydrogen isotope exchange reaction in a liquid–solid system . J. Radioanal. Nucl. Chem , 188 : 33 – 41 .
- Imaizumi , H. and Funayama , K. 1994 . Measurement of the acidities of several cation-exchange resins using hydrogen isotope exchange reaction . Bunsekikagaku , 43 : 409 – 413 .
- Okada , M. and Imaizumi , H. 1984 . Comparison of the acidity of solid phosphates by applying isotope exchange reaction using tritiated water vapor . Radiochim. Acta , 37 : 161 – 164 .
- Imaizumi , H. , Kano , N. and Hiramatsu , J. 1998 . Tritium-incorporation into ethers in T-for-H exchange reaction . Radiochim. Acta , 47 : 897 – 903 .
- Imaizumi , H. , Uchida , K. and Okada , M. 1988 . Effect of degree of polymerization and of temperature on the reactivity of poly(vinyl alcohol) by applying T-for-H exchange reaction . Nippon Kagaku Kaishi , 6 : 853 – 857 .
- Imaizumi , H. , Kobayasi , K. and Okada , M. 1987 . Effect of isomerism and of substituents on surface isotope exchange of monosubstituted benzoic acids . Radiochim. Acta , 42 : 151 – 154 .
- Imaizumi , H. and Yumoto , Y. 1995 . Comparison of the reactivity of the materials having two different kinds of functional groups by applying hydrogen-isotope exchange reaction . Radiochim. Acta , 68 : 141 – 144 .
- Okada , M. , Imaizumi , H. , Satoh , H. and Kobayashi , K. 1986 . Study on the effect of the number of carbon atoms in alkyl group on the reactivity of carboxyl or hydroxyl group in a liquid compound using hydrogen isotope exchange reaction . Radiochim. Acta , 39 : 211 – 213 .
- Kataoka , N. , Imaizumi , H. , Saito , H. , Sato , T. and Kano , N. 2010 . Two steps simultaneous analysis of reactivity of l-norvaline in the T-for-H exchange reaction – Application of Taft equation to influence of polar effect and Steric effect . Radioisotopes , 59 : 379 – 386 .
- Yoshida , A. , Imaizumi , H. , Sato , T. and Kano , N. 2009 . Kinetic analysis of the reactivity of aromatic amino acids in the T-for-H exchange reaction . Radioisotopes , 58 : 121 – 128 .
- Taft , R.W. 1952 . Polar and steric substituent constant for aliphatic and o-benzoate groups from rates of esterification and hydrolysis of ester . J. Am. Chem. Soc , 74 : 3120 – 3128 .