Abstract
In order to analyze and compare the absorbed radiation dose and dose rate of visual organs (eyes, corneas, and lenses) of the head with the surrounding areas of the head during CT scans, a mathematical head phantom was created. In addition, the amount of radiation exposure reduced when using an eye shield was also analyzed. According to the results, it was found that the absorbed dose was highest when using 120 kV (as compared to 80 kV and 100 kV) and the absorbed dose rate of the visual organs compared to the head was about 1.35% for the eyes, 0.9% for the corneas, and 0.095% for the lenses. Finally, it was shown that the dose reduction rate of each organ when using an eye shield was about 24% for the eyes, about 22% for the corneas, and more than 40% for the lenses.
Keywords:
1. Introduction
Types of X-rays that are used for diagnoses include radiography, fluoroscopy, computed tomography, interventional radiology, and bone densitometry. Computed tomography (CT) was invented in 1971 by G. Housnfied and in 1989 the helical (spiral) CT scanner was developed. Currently, dual source CT scanners are commonly used throughout the world. With developments in technology, the use of CT scans as a diagnostic tool has increased dramatically [Citation1,Citation2].
In Korea, the first total body CT was installed at KyungHee University Hospital in 1977. Since 1996, when CT was included among the benefits of medical insurance, its use has increased rapidly [Citation3].
In the US, the number of CT scans steadily rose from 2.8 million in 1981 to about 20 million by 1995, a more than seven-fold increase. As of 2006, it was reported that 2 persons per 100 had a CT examination [Citation4].
It is reported that 29 per 100 patients in Japan, and 11 per 100 patients in Germany are examined with a CT and their number continues to increase [Citation5]. However, the increase of CT testing is suspected to be a cause of the rising rate of carcinogenesis. It is already known that even low dose scans can occasionally cause leukemia, breast cancer, thyroid cancer, lung cancer, and other cancers [Citation6,Citation7].
Although a lot of X-ray radiation is needed to raise the diagnostic value for patients undergoing an X-ray test in clinical cases, doing so may have led to an increase of radiation exposure and even to have caused several disorders. For that reason, it is required to use minimal doses of radiation. As shown in the above, the diagnostic value and the radiation dose have an incompatible relationship and neither one of them should be overlooked [Citation2 Citation,8].
Head CT scans, which account for about 45% of all CT scans, are conducted to help diagnose head injuries, brain cancers, and other brain-related diseases [Citation9,Citation10]. At this time, the radiation dose loaded upon the head of patients is reported to be about 50 mGy of which about 1.5% ∼ 2% directly affects the eyes [Citation6,Citation11].
According to the International Commission on Radiological Protection (ICRP), it was reported that the radiation dose affecting the lenses was about 3 mGy during a head CT scan, 7 mGy from a paranasal sinus CT, and reaching up to 13 mGy from a CT for an ophthalmic injury [Citation9]. In addition, as an organ with high radiation susceptibility (radiosensitivity), radiation upon the lenses may cause lenticular opacity and cataracts, so protection from radiation is a critically important consideration during a head CT scan [Citation7,Citation12]. For this reason, assessing and analyzing the exposure dose quantitatively on the head and surrounding organs of a head CT scan should be a priority.
In existing studies on dose assessment of the head and surrounding organs, the dose was evaluated through direct measurement using detectors. However, because the resulting values of the detector are inconsistent due to detection inefficiencies, energy and direction dependency, it is difficult to secure reliability for the exposure dose.
Accordingly, this study intends to predict the radiation exposure expected to impact the head and eyes of a patient receiving a head CT scan by using a mathematical head phantom.
2. Materials and methods
2.1. Ophthalmic structure and radiation effects
The visual organ is an organ that senses light, is located in the orbit (a.k.a. eye socket) of the skull, and consists of an eyeball and ocular adnexa. The lenses are convex in shape and focus light onto the macula at the rear of the eyeball. The front surface of the lenses is covered with a sheath of epithelial cells. When the eyes are exposed to radiation, the conjunctive, corneas, and lenses are affected. Among them, the conjunctive showed the fastest reaction with a reaction identical to conjunctivitis. The corneas develop superficial corneitis and may develop a corneal abscess in severe cases. Finally, as for the lenses, the radiation may cause lenticular opacity which may transform into cataracts if neglected Cataracts caused by radiation have a long term latent stage [Citation13,Citation14].
Currently, the dose thresholds for radiation-induced lens opacities are 0.5–2 Gy for a single dose or 5 Gy for fractionated doses, while the dose thresholds for cataracts are 4–5 Gy for a single dose or 8 Gy for fractionated doses. However, some studies have reported that the dose thresholds for cataracts are actually 2 Gy for a single dose or 10–15 Gy for fractionated doses. The ICRP is now reviewing the possibility of low dose thresholds for radiation-induced cataracts [Citation15–Citation17].
2.2. Monte Carlo calculation
The mathematical head phantom of this study is as shown in .
The skull and brain were modified from the ORNL phantom and the composition of the visual organs simulated eyes, corneas, lenses, and the orbit surrounding the eyeballs, referencing an article by Jonathan R. in 2010 [Citation18,Citation19].
In addition, composition of materials of the organs is shown in . The density of the brain, skull bone, and eyes was 1.04 g/cm3, 1.40 g/cm3, and 1.03 g/cm3, respectively.
Table 1. Composition of the tissues for the phantoms.
As there was a previous example by Jonathan Bristol [Citation19] in 2010 and P. Nogueira et al. [Citation20] in 2011 assessing the radiation dose on the eyes using MCNP (Monte Carlo Particle Transport) code, we also chose to use MCNPX for calculating the amount of photon transport and energy deposition.
In order to calculate the exposure dose upon the eyes, corneas, lenses, and brain, CT tests were made at three different energy levels, which were 80 kVp, 100 kVp, and 120 kVp. These energy levels are typically used in actual clinical practice. It is hypothesized that the beam is generated from a total of eight sites by dividing the beam angle generated in the CT by 45°. At this time, the slice thickness was set to total 11 slices with 10 mm intervals.
An F6 tally was used to calculate the absorbed dose of photons and electrons in tissue exposed to radiation. In this study, the cutoff for electron energy was set at 1 keV because thermal noise was created by the motion of low-energy electrons, and the number of histories was 107. Relative error was below 3%.
In addition, it is necessary to apply a proper masking agent to reduce the dose accompanying the CT. In general, a bismuth shield is used for blocking the dose on the thymus and mammary glands in the chest and the crystalline lens of the eyes.
Additionally, in this study, in order to evaluate the effect of radiation protection on the visual organs, a bismuth shield of 0.5 mm, 1.0 mm, and 1.5 mm thickness was set laid over the eyes to analyze the radiation dose reduction effect on the visual organ according to the thickness of the shield.
2.3. Composition of the mathematical phantom
The mathematical phantom used in this study was generated based on the existing MIRD type human body phantom. Human body organs were composed of ovals, cones, planes, and cylinders and the absorbed dose was estimated by expressing each organ in three dimensions using a quadratic equation.
At this time, the eyes, corneas, orbit, and lenses were each composed as shown in the equation below.
(1) Eye
The location is:
(2) Cornea
(3) Bone surrounding eyes (orbit)
(4) Lenses
(5) Brain
(6) Skull
For preparing a mathematical phantom, it was intended first to predict the dose on the head of the patient including the eyes, then, second, evaluate the dose rate on the eyes compared with the head, and, third, to assess the reduction effect of the dose on the eyes depending on the use of an eye shield.
It is considered that the mathematical model used in traditional articles by P. Nogueira et al. (2011) [Citation20] and R. Behrens (2009) [Citation21] was better than the mathematical model developed in this study in terms of geometrical elements and material composition. However, whereas the mathematical model for eyes was restricted in one and the traditional studies were performed by preparing a model of the orbit (the bone structure surrounding the eyes), this study intended to perform a more practical study with a model more properly suited to evaluate the radiation exposure of the eyes accompanying a brain CT by preparing two eyes along with the cranium (brain part).
3. Results and discussions
3.1. Estimation of the absorbed dose of the head, eyes, corneas, and lenses during a CT
When setting the CT as the source area and the head, eyes, corneas, and lenses as the target areas, analysis results of the absorbed dose of the visual organs and radiation exposure rate affecting the eyes, corneas and lenses relative to the head are shown in .
Table 2. Estimation of the absorbed dose of the head, eyes, corneas, and lenses according to energy level.
3.1.1. Further details
It was found that at 80 kV, the brain, eyes, corneas, and lenses each received 31.38 mGy, 0.425 mGy, 0.285 mGy, and 0.03 mGy, respectively, and it was evaluated that using the brain as the standard, the eyes receive about 1.35%, the corneas receive about 0.9%, and the lenses receive about 0.09% of radiation exposure.
Then, it was found that at 100 kV and 120 kV, namely when the energy increased, the dose received by each organ increased as well. And notably, it was analyzed that the radiation exposure rate on the eyes, corneas, and lenses in relation to the amount absorbed by the brain was maintained to be consistent.
3.2. Estimation of the absorbed dose of the head, eyes, and corneas when using an eye shield
Results obtained by analyzing the absorbed dose of each organ and effect on the eyes, corneas and lenses compared to the head are shown in .
Table 3. Estimation of the absorbed dose of the head, eyes, corneas, and lenses when using an eye shield (thickness of shield: 0.5 mm).
First, using a bismuth shield of 0.5 mm thickness with the energy beam set at 80 kV, 100 kV, and 120 kV, the absorbed dose on the head, eyes, corneas, and lenses was assessed. It was found that the absorbed dose was 30.51 mGy ∼ 44.68 mGy for the head, 0.332 mGy ∼ 0.489 mGy for the eyes, 0.229 mGy ∼ 0.343 mGy for the corneas, and 0.019 mGy ∼ 0.029 mGy for the lenses. In addition, it was shown that the radiation exposure rate upon the eyes, corneas and lenses against that of the head was 1.067% ∼ 1.094%, 0.749% ∼ 0.766%, and 0.059% ∼ 0.065%, respectively.
When using a bismuth shield of 1.0 mm thickness, it was found that the absorbed dose was 30.32% ∼ 44.46 mGy for the head, 0.313 mGy ∼ 0.468 mGy for the eyes, 0.217 mGy ∼ 0.328 mGy for the corneas, and 0.017 mGy ∼ 0.026 mGy for the lenses. In addition, it was shown that the radiation exposure rate of the eyes, corneas, and lenses against that of the head was 1.033% ∼ 1.053%, 0.714% ∼ 0.738%, and 0.055% ∼ 0.059%, respectively ().
Table 4. Estimation of the absorbed dose of the head, eyes, corneas, and lenses when using an eye shield (thickness of shield: 1.0 mm).
When using a bismuth shield of 1.5 mm thickness, it was found that the absorbed dose was 30.25 mGy ∼ 44.40 mGy for the head, 0.213 mGy ∼ 0.32 mGy for the corneas, and 0.324 mGy ∼ 0.489 mGy for the eyes. In addition, it was shown that the radiation exposure rate of the eyes, corneas, and lenses against that of the head was 1.02% ∼ 1.046%, 0.706% ∼ 0.733%, and 0.053% ∼ 0.058%, respectively ().
Table 5. Estimation of the absorbed dose of the head, eyes, corneas, and lenses when using an eye shield (thickness of shield: 1.5mm).
3.3. Assessment of the dose reduction effect on the head, eyes, and corneas when using an eye shield
The dose reduction on the head, eyes, corneas, and lenses when using an eye shield was evaluated with the thickness of the Bismuth shielding to be 0.5 mm, 1 mm, and 1.5 mm. The results are shown in .
Figure 2. Absorbed dose reduction rate before and after use of an eye shield. (a) 80 kV. (b) 100 kV. (c) 120 kV.
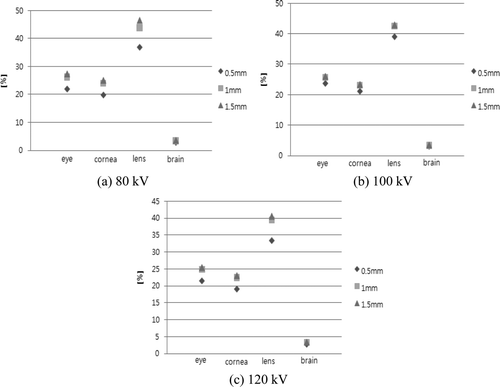
Looking at these results in further detail, it was found that the organ having the highest dose reduction effect was the lenses followed by the eyes, corneas, and head in descending order. The brain showed an insignificant reduction rate.
In addition, it was found that the total radiation reduction rate was 80 kV and the highest reduction rate was shown using a shield of 1 mm thickness. It was suggested that when the energy increased, the shielding effect decreased.
It was also found that when using a shield of 0.5 mm of thickness the radiation shield rate of each organ was highest at 100 kV of energy and when using shields of 1.0 mm and 1.5 mm of thickness, the radiation shield rate of each organ was highest at 80 kV.
4. Conclusions
This study assessed the absorbed dose clinically loaded on the visual organs during a head CT scan by configuring a mathematical head phantom. In addition, the absorbed dose reduction effect when using an eye shield was also analyzed.
Among the visual organs, the lenses are the most highly susceptible to radiation, so great care must be exercised when exposing them to a head CT scan. In terms of justification, exposure of the lenses to radiation is unavoidable when using a head CT scan to diagnose a disease. However, according to the results of this study, it was shown that the radiation exposure reduction rate of the lenses was reduced by more than 40% if an eye shield was used. On the contrary, it was found that for the brain the radiation exposure reduction rate when using an eye shield was insignificant, being about 3%. It can be deduced that an eye shield has no effect when diagnosing a brain disease, the main purpose of a head CT scan.
When considering optimization of a patient's radiation dose, protection of the corneal lenses during a head CT scan seems to be essential. Accordingly, it is considered that using an eye shield more actively within a range so as not to degrade image quality in a head CT scan, the radiation exposure of the visual organs, including the lenses, will be reduced remarkably.
References
- UNSCEAR 2000 . 2000 . Source and Effects of Ionizing Radiation United Nations Publication, New York
- Han , D.K. , Ko , S.G. , Yang , H.J. and Kim , M.C. (2007) . The evaluation of image quality and radiation dose in multi detector CT . Korean Soc Radiol. Sci , 30 : 129 – 138 .
- Lim , M.C. , Lim , J.S. , Park , H.G. and Kim , Y.H. (2004) . A study on the exposure dose for the computed tomography . Korean Soc. Radiol. Sci , 27 : 21 – 27 .
- Brenner , J. , Elliston , C.D. , Hall , E.J. and Berdon , W.E. 2001 . Estimated risks of radiation induced fatal cancer from pediatric CT . Am. J. Roentgenol , 179 : 289 – 296 .
- Grobe , H. , Sommer , M. , Koch , A. , Hietschold , V. , Henniger , J. and Abolmaali , N. 2009 . Dose reduction in computed tomography: The effect of eye and testicle shielding on radiation dose measured in patients with beryllium oxide-based optically stimulated luminescence dosimetry . Eur. Radiol , 19 : 1156 – 1160 .
- Tan , J.S.P. , Tan , K.L. , Lee , J.C.L. , Wan , C.M. , Leong , J.L. and Chan , L.L. 2009 . Comparison of eye lens dose on neuroimaging protocols between 16- and 64-section multidetector CT: Achieving the lowest possible dose . Am. Soc. Neuroradiol , 30 : 373 – 377 .
- Chiewvit , P. , Ananwattanasuk , J. , Mongkolsuk , M. , Boonma , C. and Suthipongchai , S. 2009 . Evaluation of image quality and lens's radiation dose of a low-dose cranial CT scan . J. Med. Assoc. Thai , 92 : 831 – 835 .
- Lim , C.H. , Cho , J.K. and Lee , M.K. 2007), pp . A study on the radiation dose in computed tomographic examinations . Korean Soc. Radiol. Sci , 30 : 381 – 389 .
- 2000 . International Commission on Radiological Protection, Managing patient dose in computed tomography, (Pergamon Oxford, 2000), ICRP Publication 87 . Ann ICRP , 30 : 20 – 24 .
- 2002 . National Radiological Protection Board, Radiation Exposure of the UK Population from Medical and Dental X-Ray Examinations, NRPB, Chilton, Didcot, Oxon NRPB_W4
- Jung , M.Y. , Kweon , D.C. and Kwon , S.L. 2009 . Effectiveness of Bismuth shield to reduce eye lens radiation dose using the photoluminescence dosimetry in computed tomography . Korean Soc. Radiol. Sci , 32 : 307 – 312 .
- McLaughlin , D.J. and Mooney , R.B. 2004 . Dose reduction to radiosensitive tissues in CT. Do commercially available shields meet the users' needs? . Clin Radiol , 59 : 446 – 450 .
- Un , K.K. 2009 . Human Anatomy Hyunmoon , Seoul
- Jin , K.S. 2011 . Radiobiology Jungmunkag , Seoul
- Cember , H. 1996 . Introduction to Health Physics , 3rd ed., , New York : McGraw-Hill .
- Faw , R.E. and Shultis , J.K. 1999 . Radiological Assessment Sources and Doses , Illinois, USA : American Nuclear Society .
- International Commission on Radiological Protection . 2007 . The 2007 Recommendations of the International Commission on Radiological Protection , Oxford : Pergamon Press . Publication 103,
- Cristy , M. and Eckerman , K.F. 1987 . Specific Absorbed Fractions of Energy at Various Ages from Internal Photon Sources , Oak Ridge , TN : Oak Ridge National Laboratory . ORNL/TM-8381,
- Bristol , J.R. 2010 . Comparison of Eye Plaque Dosimetry using Deterministic and Monte Carlo Methods , Degree of Master of Science : Oregon State University .
- Nogueira , P. , Zankl , M. , Schlattl , H. and Vaz , P. 2011 . Dose conversion coefficients for monoenergetic electrons incident on a realistic human eye model with different lens cell populations . Phys. Med. Biol , 56 : 6919 – 6934 .
- Behrens , R. , Dietze , G. and Zankl , M. 2009 . Dose conversion coefficients for electron exposure of the human eye lens . Phys. Med. Biol , 54 : 4069 – 4087 .