Abstract
The neutron neutron-capture cross cross-sections of 244Cm and 246Cm were measured by the time-of-flight method in the energy range of 1–300 300 eV with an array of large germanium detectors in the Accurate Neutron-Nucleus Reaction measurement InstrumentANNRI at Material and Life Science Experimental Facility (MLF) of the Japan Proton Accelerator Research ComplexJ-PARC/MLF. The 244Cm resonances at around 7.7 and 16.8 8 eV and the 246Cm resonances at around 4.3 and 15.3 3 eV were observed in the capture reactions for the first time. The uncertainties of the obtained cross cross-sections are 5.8% at the top of the first resonance of 244Cm and 6.6% at that of 246Cm. The rResonance analyses were performed for low-energy ones using the code SAMMY. The prompt γ-ray spectra of 244Cm and 246Cm were also obtained. Eight and five new prompt γ-ray emissions were observed in the 244Cm(n, γ) and 246Cm(n, γ) reactions, respectively.
1. Introduction
Accurate neutron-capture cross-section data for minor actinides (MAs) are required to estimate the production and transmutation rates of MAs in various reactors, including thermal and innovative reactor systems [1]. Accurate measurements of these cross-sections, however, are very difficult due to their high-specific radioactivity. Therefore, the data with high accuracy are not available [1].
244Cm (half-life: 18.1 years [2]) and 246Cm (half-life: 4730 years [2]) are among the most important MAs [1]. 244Cm shares nearly 50% of the total actinide decay heat in spent reactor fuels even after three years of cooling [3], making the acquisition of accurate data especially important. However, there is only one reported experimental data set on the measurement of the neutron-capture cross-sections of 244Cm and 246Cm in the resonance region [4], and the uncertainties of the data are much larger than the required accuracy of 4.1–25.7% [1].
To satisfy the requirement for accurate data on the neutron-capture cross-sections of MAs and long-lived fission products, Accurate Neutron-Nucleus Reaction measurement Instrument (ANNRI) has been developed in the Material and Life Science Experimental Facility (MLF) of the Japan Proton Accelerator Research Complex (J-PARC), which provides the most intense pulsed neutron beam in the world [5–7]. A series of neutron-capture cross-section measurements, including those of 244Cm and 246Cm, have been started using an array of large germanium (Ge) detectors [8] and a high-performance data acquisition system (DAQ) [9,10] in the ANNRI. Parts of preliminary results for 244Cm and 246Cm were reported [11,12]. This article presents the new results of neutron-capture cross-sections and resonance information of 244Cm and 246Cm in the neutron energy range from 1 to 300 eV obtained through complementary measurements and detailed analysis of the data.
This article is organized as follows: in section 2, an overview of our instruments and the experimental setup are presented. Section 3 describes the procedure used to obtain prompt γ-ray and time-of-flight (TOF) spectra, and their results. By taking advantage of the ANNRI, some prompt γ-rays from 244Cm and 246Cm were identified. Section 4 describes the data analysis used to obtain the neutron-capture cross-sections of 244Cm and 246Cm and the results. In section 5, we discuss the uncertainties in the obtained neutron-capture cross-sections, and conclude this article in section 6.
2. Experiments
2.1. Overview of the ANNRI
The ANNRI is an instrument for measuring neutron-capture cross-sections with high-intensity pulsed neutrons from the No. 04 beam port in the MLF, to which the proton pulses are supplied by the 3-GeV Rapid-Cycling Synchrotron of J-PARC. The proton beams are usually supplied at a repetition rate of 25 Hz and in the “double-bunch mode,” in which the pulsed protons consist of two bunches, each with a width of 100 ns, at intervals of 600 ns [13].
shows the structure of ANNRI. The ANNRI uses neutrons from a coupled moderator (with a 140-mm diameter and 120-mm height) that provides the most intense neutron beam among the moderators at the Japan Spallation Neutron Source (JSNS) [13]. The neutron beam goes through collimators and a beam duct to reach the sample positions. There are two sample positions in the ANNRI, one of which is for measurements of γ-rays using the array of large Ge detectors. This sample position is located 21.5 m from the center of the moderator. The neutron beams are collimated to diameters of 3, 7, 15, and 22 mm with the collimator closest to the sample position. The energy-integrated neutron intensities at the sample position are 5.2 × 1010 n/s/m2 in the neutron energy range of 1.5–25 meV and 7.2 × 109 n/s/m2 in 0.9–1.1 keV under a proton beam power of 120 kW [14].
Figure 1. A side view of NNRI. The neutron beam emitted from the moderator goes through the T0 chopper, neutron filter, disk choppers, collimators and the two detector systems, and then is dumped into the beam stopper. The sample positions for the array of large Ge detectors and NaI detectors are 21.5 and 27.9 m from the moderator, respectively.
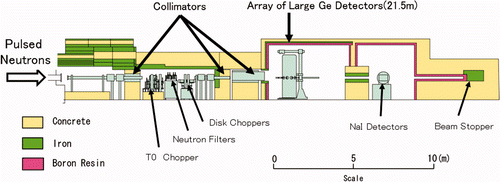
2.2. Array of Ge detectors
The array of Ge detectors is composed of two cluster-Ge detectors, eight coaxial-Ge detectors and anti-coincidence shields around each Ge detector as seen in [8]. Each cluster-Ge detector consists of seven Ge crystals. The positions of the cluster-Ge detectors were arranged so that the distance between the front surface of each detector and the center of the sample position was 125 mm. A lead plate with a thickness of 3 mm was placed in front of each cluster-Ge detector for the attenuation of the intense decay γrays (42.8, 98.9, and 152.6 keV) from 244Cm. A 10-mm-thick borated silicone rubber (polydimethylsiloxane) sheet and 6LiH powder with a total thickness of 40 mm (sealed in aluminum cases) were also placed in front of each Ge detector. The peak efficiency of the spectrometer is 2.28 ± 0.11% for 1.33 MeV γ rays [8]. Typical energy resolutions (full width at half maximum, FWHM) for 1.33-MeV γ-rays are 5.5 keV (beam-on) and 2.4 keV (beam-off). The spectrometer does not discriminate γ rays due to fission events from those due to capture events. Therefore, the influences of fission events wereevaluated and subtracted during the analysis (seesection 4.7).
Figure 2. Layout of the array of Ge detectors. The array is composed of two cluster-Ge detectors, eight coaxial-Ge detectors and anti-coincidence shields around each Ge detector.
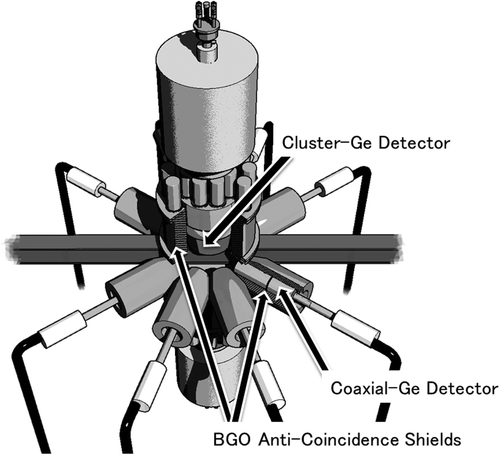
A high-count rate and high-resolution DAQ, based on a digital data processing technique, was developed [9,10]. In the data acquisition, γ-ray pulse heights, TOFs were recorded together with detector IDs by the DAQ (list mode).
2.3. Samples
The 244Cm sample for γ-ray measurements contained 0.6 mg of curium oxide. Its isotopic enrichment was 90.1 mole%, and its main activity was 244Cm 1.8 GBq. The 246Cm sample contained 2.1 mg of curium-oxide. Its isotopic enrichment was 59.4 mole% with a 27.5 mole% contamination of 244Cm, and its main activities were 246Cm 12.1 MBq and 244Cm 1.7 GBq. To fulfill the safety regulations at MLF, each sample was sealed in an aluminum case 9 mm in outer diameter, weighing 280 mg, and having 0.5-mm-thick walls. The samples were supplied by the Russia Research Institute of Atomic Research after sealing them in the aluminum cases. Each sample was put in a bag of fluorinated ethylene propylene (FEP) films and attached to a sample holder. The sample holders were made of polytetrafluoroethylene and shaped into a 50 mm × 70 mm rectangular frame, which was made much larger than the size of the collimated neutrons (7 mm) to avoid background production by neutron beam.
To make detailed isotopic and impurity analyses, a small amount of sample for each batch of curium oxide powder was also supplied for detailed isotopic and impurity analyses. Using these samples, the ratios of isotopic abundance of curium isotopes were determined by thermal ionization mass spectrometry (TIMS). The ratios of number density of 240Pu (a daughter nuclide of 244Cm) and 246Cm to 244Cm were determined by α -particle spectrometry at KURRI [15] and are listed in . In the data reduction, the isotopic ratios of 244–248Cm measured by TIMS and the ratios of 240Pu measured by α-particle spectrometry were used in the analysis.
Table 1. Isotopic and chemical impurities of curium oxide in the 244Cm and 246Cm samples [15].
The masses of 244Cm and 246Cm in the samples were not known with high accuracy, which made difficult thenormalization of cross-section scale. However, the 240Pu first resonance whose resonance parameters areestablished relatively well, was clearly observed in the TOF spectra for both samples. Therefore, in this article, the absolute neutron-capture cross-sections of 244Cm and 246Cm were obtained by normalizing the relative yields to the evaluated values of the first resonance of 240Pu in JENDL-4.0 [16]. The details of the normalization are described in section 4.5.
2.4. Measurement conditions
In the measurements, two cluster-Ge detectors were used, but the coaxial-Ge detectors were not used because they suffered from severe electrical noise. The total measuring time with the ANNRI was about 64 h (4.88 × 106 shots) for the 244Cm sample and about 94 h (6.85 × 106 shots) for the 246Cm sample. For the background estimation, γ-ray measurements with an empty aluminum case (dummy case) and neither a sample material nor the sample case (blank, i.e. only a sample holder and an FEP bag) were also carried out for 48 h (3.51 × 106 shots) and 44 h (3.88 × 106 shots), respectively.
During the experiment, the first 72 proton shots (i.e. 144 bunches) out of every 75 were provided to JSNS, and the last 3 proton shots (i.e. 6 bunches) were provided to other facilities. The proton intensity per shot was stable within 1% in FWHM. To reduce the scattering of neutrons by air, the air in the beam duct was replaced with helium gas. The neutron beam was collimated to a 7-mm diameter at the sample position.
For dead-time correction, pulses from a random-pulse generator (Berkeley Nucleonics, DB-2) were input to the pre-amplifier of every Ge crystal [17]. At the same time, the number of pulses was counted by a fast counter. The amplitude of the random pulses was adjusted to be above that of 9.5 MeV γ-rays, which is the highest energy among probable γ-rays in the measurement. 9.5 MeV is higher than the neutron separation energies of the isotopes of interest (245Cm (5520.3 ± 1.0 keV), 247Cm (5156 ± 4 keV), 249Cm (4713.5 ± 0.3 keV), and 241Pu (5241.52 ± 0.03 keV) [2]) and the energies of most of the intense background γ-rays from 54Fe (9.298 MeV) (described in the following section). The average repetition rate of the random-pulse generator was set to about 1 × 103 Hz.
3. 𝛄-Ray pulse-height and TOF spectra
3.1. 𝛄-Ray pulse-height spectra
3.1.1. Raw spectra
shows three γ-ray pulse-height spectra for (1) 244Cm sample with neutron beam on, (2) dummy case with neutron beam on, and (3) decay γ-ray measured with one of the 14 Ge crystals. shows those from the 246Cm sample and the dummy case. Almost all the intense photo peaks in the spectra for 244Cm and 246Cm with beam on are observed also in the dummy case and the decay γ-ray. For example, 478-keV γ-rays from the 10B(n, αγ) reaction, prompt γ-rays from 27Al(n, γ)28Al reactions, and decay γ-rays from 28Al are observed in both the γ-ray spectra of the samples and the dummy case. They are main components of background γ-rays. The peaks originating from 244Cm and 246Cm neutron-capture events were hidden under the intense background γ rays because the amounts of the curium oxide powders (0.6 and 2.1 mg) are much smaller than the amounts of aluminum (280 mg).
3.1.2. Resonance-gated spectra for the first resonances of 244Cm and 246Cm
To eliminate background γ-rays and obtain prompt γ-rays emitted in the 244Cm(n, γ), 246Cm(n, γ), and 240Pu(n, γ) reactions, resonance-gated net spectra for the first resonances of 244, 246Cm and 240Pu were constructed by the following procedures:
1. | A gate was set in the first resonance of the raw TOF spectrum to obtain a resonance-gated γ-ray pulse-height spectrum for each Ge crystal. | ||||
2. | Gates were set on both side of the resonance gate to obtain off-resonance-gated γ-ray pulse-height spectra for each crystal. The width of each off-resonance gate was half the width of the resonance gate. | ||||
3. | Dead-time correction was done for each spectrum [17]. | ||||
4. | All of the 14 resonance-gated γ-ray pulse-height spectra were added after the dead-time correction. | ||||
5. | All of the 28 off-resonance spectra were added after the dead-time correction. | ||||
6. | The off-resonance-gated sum spectrum was subtracted from the resonance-gated sum spectrum to obtain the resonance-gated net spectrum. |
To check that the backgrounds were subtracted appropriately, the resonance-gated net spectrum was compared to the sum of the resonance-gated spectra (obtained in step (4)). The resonance-gated sum spectrum and the background-subtracted net spectrum of the 240Pu first resonance in the 246Cm sample data are compared in . In the resonance-gated spectrum, peaks from the 478 keV background γ-rays, prompt γ-rays from 27Al(n, γ) reactions, and decay γ-rays are observed. On the other hand, in the resonance-gated net spectrum, these peaks disappear and peaks from many known prompt γ-rays from 240Pu [2] are observed. Consequently, it was confirmed that the backgrounds were subtracted appropriately and the spectrum contained only the prompt γ-rays from 240Pu.
Figure 5. The resonance-gated net spectrum (black solid line) and the sum of the resonance-gated γ-ray pulse-height spectra (gray solid line) in the first resonance of 240Pu in the 246Cm sample data.
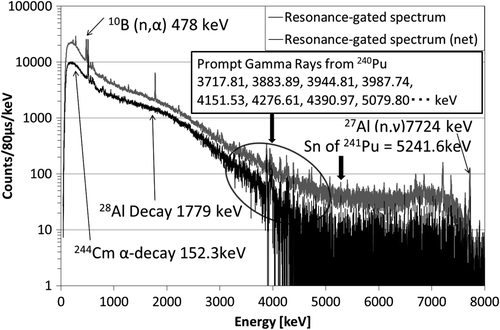
In the same way, the resonance-gated net spectra of 244Cm and 246Cm were obtained. The resonance-gated net spectra in the first resonance of 244Cm determined from the 244Cm and 246Cm sample data are shown in . The energies of the observed prompt γ-rays are listed in . The 252.4- and 380.8-keV γ-rays have already been studied in α decay of 249Cf [18], electron capture decay of 245Bk [19], and β−-decay of 245Am [20]. The other γ-rays were not known until now. Transition assignments are also listed in . The 4524- and 4885-keV γ-rays are assigned to primary transitions to the 252.84-keV [19] and 633.64-keV [21] states. The 252.4- and 380.8-keV γ-rays have already been assigned in Ardisson et al. [18] and Ahmad et al. [19]. In this study, the 3250-, 3260-, 3865-, and 4122-keV γ-rays could not be assigned to transitions between the known energy levels.
Figure 6. The resonance-gated net spectra in the first resonance of 244Cm from the 244Cm sample (black dashed line) and the 246Cm sample (gray solid line). Asterisks (*) indicate previously unknown γ-rays.
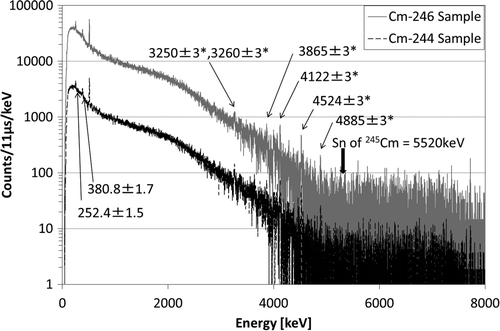
Table 2. Observed γ-ray energies and transition assignments.
The resonance-gated net spectrum in the first resonance of 246Cm from the 246Cm sample data is shown in . The energies of the observed prompt γ-rays and their transition assignments are also listed in . All the prompt γ-rays are previously unknown. The 438.3- and 892.4-keV γ-rays were assigned to ground-state transitions from the 439- and 897-keV states, and the 4206- and 4493-keV γ-rays were assigned to primary transitions to the 947- and 668-keV states [21]. The 3634-keV γ-ray could not be assigned.
3.2. TOF spectra
Typical TOF spectra of the 244Cm and 246Cm samples measured with one of the 14 crystals are shown in . In the spectra, the events due to the random timing pulses were eliminated by applying a gate below 9.5 MeV on the γ-ray pulse-height spectra. In the spectrum of the 244Cm sample, not only the resonances of 244Cm but also those of impurities 246Cm, 240Pu, and 243Am are observed. In the spectrum of the 246Cm sample, the resonances of impurities 244,245,247, and 248Cm, 240Pu, and 243Am are also clearly observed. Therefore, to obtain neutron-capture cross-sections of 244Cm and 246Cm, the contributions from these impurities must be evaluated and subtracted.
4. TOF data analysis
To obtain neutron-capture cross-sections of 244Cm and 246Cm, the influences of impurities and fission events must be evaluated and subtracted. Furthermore, the masses of the curium oxide are not known. Therefore, normalizations were needed to obtain absolute values of the cross-sections. The data of each sample were analyzed with the procedure shown in . Details of each step are described in the following sections.
4.1. Dead-time correction
In the first step of the analysis, the data were corrected for the dead-time (effect). In the experiments, the events of both the random timing pulses and the pulses that originated from γ-rays were recorded with the DAQ. At the same time, the number of random timing pulses was counted using a scaler circuit. TOF spectra S Cor (t) were corrected using the following equation [17]:
4.2. Background estimation and subtraction
After the dead-time correction, the background was also estimated for each sample and crystal. In this step, the contributions from overlapping neutrons and decay γ-rays and those of scattering or capturing by materials other than the curium samples (the aluminum case or helium gas, for example) to the background were estimated separately.
4.2.1. Estimation and subtraction of background due to overlapping neutrons and decay γ-rays
During the experiment, the first 72 of every 75 shots were provided to JSNS and the other shots to the other facilities. Therefore, every 3 seconds, a TOF measurement over a wide time range was done. The backgrounds due to the overlapping neutrons and the decay γ-rays were deduced from this TOF spectrum using the TOF range after 40 ms (= 1/25 s).
shows a typical TOF from the 246Cm sample spectrum measured with one of the crystals and the corresponding TOF dependence of the area count due to the random timing pulses in an 80-ms TOF range. The number of events from both the random timing pulses and those that originated from γ-rays suddenly decreased at 40 ms. This is due to the pattern of the proton injection, as explained in the previous paragraph; the TOF events after 40 ms were recorded once in every 72 proton injections. To correct for the decrease, the number of TOF events after 40 ms was multiplied by 72. After correction for the decrease, the dead-time correction was done. The corrected TOF spectrum is shown along with the corresponding raw spectrum in .
Figure 10. A typical time dependence of the live-to-real time ratio (red dotted line) and the corresponding TOF spectra with (blue dashed line) and without (black solid line) the dead-time correction from the 246Cm sample measured with one of the Ge crystals.
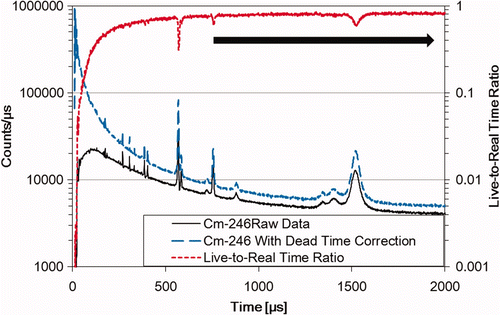
Figure 11. A typical TOF spectrum measured with one of the crystals (blue dotted line), the corresponding TOF dependence of the area count due to the random timing pulses (red dashed line), the TOF spectra with the dead-time correction (light-blue solid line), the fitted curve (black dashed-dotted line), and the deduced background (black solid line) in the 0–80 ms TOF range from the 246Cm sample.
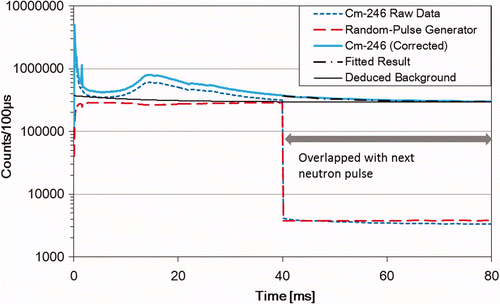
The corrected spectrum in indicates that the influence of low-energy neutrons remained after a TOF of 40 ms. In every 40 ms interval, neutrons were produced by a proton shot. Some of the low-energy neutrons arrive at the sample at the same time as high-energy neutrons from the next proton pulse. Therefore, the TOF events before 40 ms included the influence of the low-energy neutrons that were generated by previous proton shots, i.e. overlapping neutrons. Thus, the background due to the overlapping neutrons should be estimated and subtracted. In this analysis, the background was estimated from the data after 40 ms. However, the data after 40 ms include not only the influence of overlapping neutrons but also that of decay γ-rays from 244Cm, 246Cm, 28Al, and the other activated materials. These influences contributed differently to the data before 40 ms, and these components were deduced separately by fitting the data after 40 ms to the following function:
This calculation was done for each sample and crystal. An example of the calculated background is also shown in . In this step, propagations of fitted errors were taken into account.
4.2.2. Estimation and subtraction of background due to scattering and capturing by materials other than the curium samples
After subtracting the contributions from overlapping neutrons and decay γ-rays, those of scattering and capturing by materials other than the curium samples were deduced from the measurement of the dummy case. The slight difference in aluminum masses between the curium samples and the dummy case was corrected using the measurements of the blank by normalizing with the areas of the 7724-keV γ-rays from 27Al(n, γ) reactions.
The background estimation and subtraction were done for each TOF spectrum collected at each crystal. shows a typical estimated background for the 246Cm sample measured with one of the crystals. The corresponding TOF spectrum before the background subtraction described in the previous section is also shown in . In this step, statistical errors in the number of 7724-keV prompt γ-rays were taken into account.
Figure 12. A typical estimated background of the 246Cm sample measured with one of the crystals (red dashed line) and the corresponding TOF spectrum minus the background due to overlapping neutrons and decay γ-rays (black solid line).
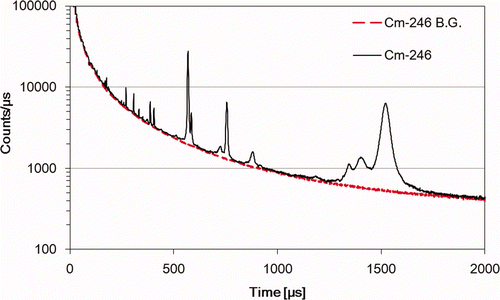
The corrections in the previous steps were done separately for each crystal, because the correction factors depend on the positions of the crystals. On the other hand, corrections done in the following steps are independent of the positions. Therefore, from here on, the sum spectrum of all 14 TOF spectra was analyzed for each sample. shows the sum spectra of the 244Cm and 246Cm samples and the uncertainties. The spectra show the number of events per proton shot per eV of neutron energy. The TOF parameters, such as a flight length for the neutron energy calibration, were taken from Kin et al. [22], in which they had been deduced from a TOF spectrum of a gold sample and the resonance energies of gold.
4.3. Self-shielding and multiple-scattering correction
Correction factors for neutron self-shielding and multiple scattering were calculated with the Monte Carlo simulation code MCNP [23]. In the calculation, the sample size, shape, mass, isotope abundances, and intensity distribution of neutrons were taken into consideration. The calculated correction factors for the 244Cm and 246Cm samples are shown in . The sharp positive peaks in the factors are due to self-shielding, and the broad dips are due to multiple-scattering neutrons. Uncertainties in the factors included those from uncertainties in the nuclear data in JENDL-4.0, those in isotopic and chemical impurities, and 10% of the systematic errors in the sample mass, shape, and mounted position.
4.4. Calculation of relative yield
In the present analysis, the energy dependence of the incident neutron flux reported in Ohta et al. [24] was used to calculate relative cross-sections. In Ohta et al. [24], the flux was determined by measuring 478-keV γ-rays emitted in 10B(n, αγ) reactions. The 10B(n, αγ) cross-section in JENDL-4.0 was used. shows the averaged neutron flux and neutron-energy-dependent errors. To reduce the statistical uncertainty, the flux was averaged by integrating over 40 points in the neutron energy range under 2 eV, 20 points in 2–60 eV, 10 points in 60–250 eV, and 5 points in 250–300 eV. Because the cross-sections will be normalized in the following section, the relative errors (statistical errors and error in the self-shielding and multiple-scattering corrections) discussed in Ohta et al. [24] were taken into account and the absolute errors (for example, error in the amount of 10B and error in the peak efficiency for 478-keV γ-rays) were ignored.
Figure 15. The averaged neutron flux at the 21.5-m sample position (black solid line) and neutron-energy-dependent errors (gray dashed line).
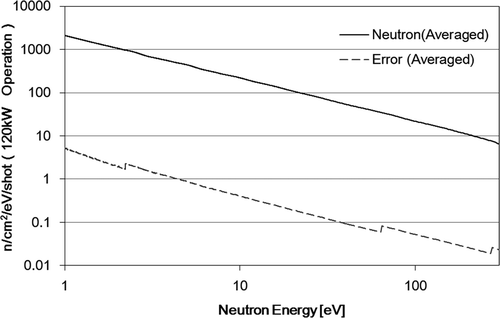
The relative yields of the 244Cm and 246Cm samples were calculated from the sum spectra shown in , the neutron self-shielding and multiple-scattering correction factors shown in , and the averaged neutron flux shown in .
4.5. Cross-section normalization
As mentioned above, the accurate masses of the curium oxide powders are unknown and it is difficult to determine by simple measurements of decay γ-rays from the curium isotopes in the samples because the accuracy of γ-ray emission probability of curium isotopes is only 10% even in the best case [2]. Furthermore, the relative yields of 244Cm and 246Cm at thermal energy could not be calculated because the fission cross-section of 245Cm at thermal energy is large (2411 b in JENDL-4.0) and the 244Cm and 246Cm samples were contaminated with 245Cm (2.71 and 1.06%, respectively). Therefore, it is also difficult to normalize the relative yields to nuclear data at thermal energy. On the other hand, the first resonance of 240Pu, which is a daughter nuclide of 244Cm, was clearly observed in the TOF spectrum of each sample as shown in , and the cross-sections of 240Pu at the first resonance are relatively well established. Therefore, the cross-section was derived by normalizing the relative yields to the evaluated values in JENDL-4.0 using the resonance area of the first resonance of 240Pu. For 240Pu, contaminations of the fission events are small and negligible because the fission cross-section of 240Pu at the first resonance is less than 0.1% of the neutron-capture cross-section in JENDL-4.0.
In the normalization, it was assumed that the sensitivities of the spectrometer to the neutron-capture events of 244Cm and 246Cm were the same in the all-neutron energy ranges and that the value of the sensitivities used was that of 240Pu at the first resonance, i.e.
The relative yields were normalized at the first resonance of 240Pu to the weighted evaluated values in JENDL-4.0 by the abundance of 240Pu listed in . The normalized yield from the 244Cm sample is shown in and that from the 246Cm sample is shown in . The normalized yields were weighted by the abundance listed in .
Figure 16. The normalized yields weighted by the abundance listed in Table 1 (red solid line); the contributions from neutron-capture events of 240Pu, 243Am, and 244, 246Cm (black dashed line); and uncertainties in the contributions (blue dotted line) from the 244Cm sample in the neutron energy region from 1 to 100 eV.
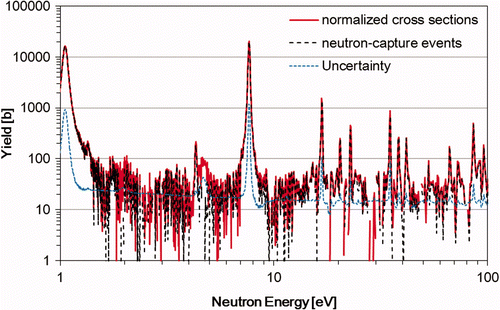
Figure 17. The normalized yields weighted by the abundance listed in Table 1 (red solid line); the contributions from neutron-capture events of 240Pu, 243Am, and 244, 246, 248Cm (black dashed line); and uncertainties in the contributions (blue dotted line) from the 246Cm sample in the neutron energy region from 1 to 100 eV.
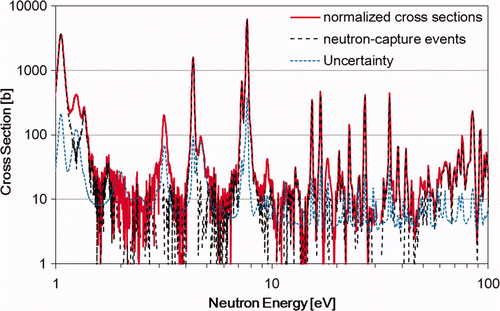
4.6. Evaluation of the abundance of 243Am
Although the isotope 243Am is not included in the isotope and impurity analyses given in , the resonances of 243Am are clearly observed in the TOF spectra from the 244Cm and 246Cm samples shown in . Therefore, to evaluate the abundances of 243Am, the normalized yields at the first resonance of 243Am were fitted with SAMMY-7, the multilevel R-matrix Bayesian code [26]. In the fits, Multi-Level Breit–Wigner formalism was used. The proton beam pulse width of 600 ns, the TOF channel width of 1 μs, the neutron production target size of 10 cm, and the flight length of 21.5 m were used as input parameters. The free gas model with a target temperature of 293 K was applied to the Doppler broadening. The resonance parameters in JENDL-4.0 were used. The abundance of 243Am was varied in each fitting. The influence of fission events was small and negligible, because the fission cross-section of 243Am at the first resonance is less than 1% of the neutron-capture cross-section in JENDL-4.0. The obtained abundance was 1.06 ± 0.10% for the 246Cm sample and 0.63 ± 0.04% for the 244Cm sample.
4.7. Evaluation and subtraction of influence of fission events
To obtain accurate neutron-capture cross-sections for 244Cm and 246Cm, the sensitivity of the spectrometer to the fission events must be evaluated, and the influence of the fission events must be subtracted.
The normalized yields are described in the following equation:
The first resonance of 247Cm is clearly observed in the TOF spectrum from the 246Cm sample as shown in . The fission cross-section of 247Cm at the first resonance is large in JENDL-4.0. Therefore, the ratio of the sensitivity of the fission events to that of the capture events was evaluated using the first resonance of 247Cm. Using the abundance listed in , the abundance of 243Am evaluated in section 4.6, and the values of JENDL-4.0, the ratio was deduced to be 2.3 ± 1.0 for the spectrometer. This ratio was named the fission correction factor. The error in the fission correction factor includes the uncertainty of the isotopic impurities, as shown in , the normalization error, and the uncertainty of the evaluated nuclear data of 247Cm.
Using the fission correction factor and the resonance parameters in JENDL-4.0, contributions from the fission events of all nuclides were calculated with SAMMY to consider the broadenings. These contributions were subtracted from the normalized yields. In this article, the capture cross-sections of 245Cm and 247Cm could not be discussed, because the fission cross-sections of these nuclides are larger than the capture cross-sections and the fission correction factor has a large error. Therefore, the contributions from the neutron-capture events of 245Cm and 247Cm were also calculated and subtracted in this step. As a result, capture yields of 240Pu, 243Am, and 244, 246, 248Cm (but not 245Cm and 247Cm) from the 244Cm and 246Cm samples were obtained. These are shown in and . The uncertainties shown in and include the errors discussed in previous sections, the error in the fission correction factor, the errors of theevaluated nuclear data of fission cross-sections, and the uncertainties of the impurities listed in .
4.8. Resonance analysis with SAMMY
Resonance parameters in the neutron energy range of 1.0–30 eV were extracted from the capture yields shown in and using SAMMY. The SAMMY fitting conditions were the same as those given in section 4.6. The ratios of the isotopic and chemical impurities in the samples given in and that of 243Am obtained in section 4.6 were used. In the analysis, only the resonance parameters E, Γ n , and Γγ were allowed to vary.
The final parameters from the SAMMY fits of both samples are listed in , along with the evaluated parameters in JENDL-4.0. The values of Γ n and Γγ for the second resonance of 240Pu and the resonances of 248Cm are consistent within error with the values in JENDL-4.0. The energies for all resonances are also consistent with the energy values in JENDL-4.0.
Table 3. Obtained resonance parameters along with the evaluations of JENDL-4.0 [16].
shows the obtained capture yields from the 244Cm sample, along with the corresponding uncertainties, the SAMMY-fitted results, and the contribution from each nuclide as obtained by the SAMMY fit. The capture yields were weighted by the abundances listed in . shows the results for the 246Cm sample. In , the weighted capture yields and the SAMMY-fitted results are compared to JENDL-4.0 (broadened and weighted with SAMMY) around the first resonance of 244Cm. The comparisons around the first resonance of 246Cm are shown in . As shown in the , there are residuals between the capture yields and the fitted results in the neutron energy range between 7.3 and 7.8 eV at the first resonance of 244Cm. This behavior of the residuals is also observed at the first resonance of 246Cm as shown in . These may be caused by small differences between the real shape of the pulsed neutrons and the shape that was used in the SAMMY fits. The spread in neutron energy for a measured flight time (resolution function) must also be known for detailed discussions.
Figure 18. The obtained capture yields weighted by the abundance in Table 1 (gray circles), with the corresponding uncertainties (black dashed line), the SAMMY [26]-fitted results for all nuclides (purple solid line), 244Cm (red dashed line), 246Cm (blue dashed line), 243Am (orange dashed line), and 240Pu (green dashed line) using the parameters shown in Table 4 (below 30 eV) and the JENDL-4.0 [16] (above 30 eV) in the neutron energy region from 1 to 100 eV for the 244Cm sample.
![Figure 18. The obtained capture yields weighted by the abundance in Table 1 (gray circles), with the corresponding uncertainties (black dashed line), the SAMMY [26]-fitted results for all nuclides (purple solid line), 244Cm (red dashed line), 246Cm (blue dashed line), 243Am (orange dashed line), and 240Pu (green dashed line) using the parameters shown in Table 4 (below 30 eV) and the JENDL-4.0 [16] (above 30 eV) in the neutron energy region from 1 to 100 eV for the 244Cm sample.](/cms/asset/fbcd7108-004a-4903-9900-0ade63ad357b/tnst_a_693887_o_f0018g.jpg)
Figure 19. The obtained capture yields weighted by the abundance in Table 1 (gray circles), with the corresponding uncertainties (black dashed line), the SAMMY [26]-fittedresults for all nuclides (purple solid line), 246Cm (red dashed line), 244Cm (blue dashed line), 248Cm (light green dashed line), 243Am (orange dashed line), and 240Pu (green dashed line) using the parameters shown in (below 30 eV) and the JENDL-4.0 [16] (above 30 eV) in the neutron energy region from 1 to 100 eV for the 246Cm sample.
![Figure 19. The obtained capture yields weighted by the abundance in Table 1 (gray circles), with the corresponding uncertainties (black dashed line), the SAMMY [26]-fittedresults for all nuclides (purple solid line), 246Cm (red dashed line), 244Cm (blue dashed line), 248Cm (light green dashed line), 243Am (orange dashed line), and 240Pu (green dashed line) using the parameters shown in Table 4 (below 30 eV) and the JENDL-4.0 [16] (above 30 eV) in the neutron energy region from 1 to 100 eV for the 246Cm sample.](/cms/asset/d297e2a9-9565-4e2d-bf2e-0197474b4028/tnst_a_693887_o_f0019g.jpg)
Figure 21. The capture yields weighted by the abundance in Table 1 from the 246Cm sample with error bars (blue circles), the SAMMY [26] fits using the parameters shown in Table 4 (blue solid line), and comparison to JENDL-4.0 [16] (broadened with SAMMY, black dashed line) around the first resonance of 246Cm.
![Figure 21. The capture yields weighted by the abundance in Table 1 from the 246Cm sample with error bars (blue circles), the SAMMY [26] fits using the parameters shown in Table 4 (blue solid line), and comparison to JENDL-4.0 [16] (broadened with SAMMY, black dashed line) around the first resonance of 246Cm.](/cms/asset/4990a2e0-e572-43f1-8521-5b8c06cb5948/tnst_a_693887_o_f0021g.jpg)
4.9. Neutron-capture cross-sections of 244Cm and 246Cm
In the case of the 244Cm sample, contributions from impurities of 240Pu, 243Am, and 246Cm (without 244Cm) were calculated with SAMMY using the parameters shown in and JENDL-4.0. The contributions from 244Cm were calculated by subtracting the contributions from the impurities from the capture yields shown in . Neutron-capture cross-sections of 244Cm were obtained by dividing the 244Cm contributions by the isotopic ratio of 244Cm listed in . The errors in the obtained cross-sections were deduced from the uncertainties of the abundances (shown in ) and either the fitting error of the SAMMY fits (below 30 eV) or the uncertainty of the evaluated nuclear data (above 30 eV). Neutron-capture cross-sections of 246Cm were calculated analogously.
shows a comparison of the obtained neutron-capture cross-section of 244Cm with the one given by Moore [4] and with JENDL-4.0 (broadened with SAMMY). shows this comparison for 246Cm. The resonances at around 7.7 and 16.8 eV for 244Cm and 4.3 and 15.3 eV for 246Cm were observed in the capture reactions for the first time. The obtained cross-section of 246Cm shown in has a small peak around 7.5 eV. This is not a resonance peak of 246Cm but due to the fitting residuals, as shown in .
Figure 20. The capture yields weighted by the abundance in Table 1 from the 244Cm sample (red circles) and the 246Cm sample (blue triangles) with error bars, the results of the SAMMY [26] fits using the parameters shown in Table 4 (244Cm sample: red solid line, 246Cm sample: blue solid line), and comparison to JENDL-4.0 [16] (broadened with SAMMY, 244Cm sample: black dotted line, 246Cm sample: black dashed line) around the first resonance of 244Cm.
![Figure 20. The capture yields weighted by the abundance in Table 1 from the 244Cm sample (red circles) and the 246Cm sample (blue triangles) with error bars, the results of the SAMMY [26] fits using the parameters shown in Table 4 (244Cm sample: red solid line, 246Cm sample: blue solid line), and comparison to JENDL-4.0 [16] (broadened with SAMMY, 244Cm sample: black dotted line, 246Cm sample: black dashed line) around the first resonance of 244Cm.](/cms/asset/40037ac1-f6ac-4c52-a7c3-fa6af721c2d5/tnst_a_693887_o_f0020g.jpg)
5. Discussion
5.1. Uncertainties
5.1.1. Additional systematic errors
All the statistical uncertainties and some systematic uncertainties were discussed in section 4. In this section, systematic errors in the normalization and in the influence of scattered neutrons were discussed.
5.1.1.1. Systematic errors in the normalization
In this paper, the cross-sections were obtained by normalizing the relative cross-sections to the values in JENDL-4.0 at the first resonance of 240Pu. The sensitivities of the spectrometer to 240Pu and 244,246Cm were assumed to be the same across the entire neutron energy range. To estimate the systematic error in this assumption, the ratios of the sensitivities of the capture events at the resonances of 244, 246Cm to that at the first resonance of 240Pu were calculated with the PHWT and assuming a simplified weighting function of the spectrometer [27]. In the spectrometer, the efficiency of each Ge crystal is low enough to use the PHWT (about 0.5% for 662-keV γ-rays [22]).
In the PHWT, the neutron-capture cross-section of 246Cm is calculated by the following equation:
5.1.1.2. Systematic errors in influences of the scattering
In the present experiments, there were four main origins of γ-rays due to scattered neutrons:
• | Neutrons scattered by materials in the neutron beam except for the curium oxide (e.g. the Al case and the He gas in the beam duct) that were captured by the curium oxide (not the Al case, but the 244Cm, 246Cm, 240Pu, and so on). | ||||
• | Neutrons scattered by materials in the neutron beam that were captured by materials other than the curium oxide (e.g. the Al case, the beam duct, and the detectors). | ||||
• | Neutrons scattered by the curium oxide that were captured by the curium oxide. | ||||
• | Neutrons scattered by the curium oxide that were captured by the materials other than the curium oxide. |
To deduce the magnitude of this influence, the ratio of the influence to neutron-capture events at the first resonances of 244Cm and 246Cm were calculated with the following assumptions:
• | In the net spectra of the first resonances of 244Cm and 246Cm, events in γ-ray energy regions of 6–8 MeV are only due to the fourth origin; these regions are above the neutron-separation energies of 245Cm and 247Cm. (The events originating from fission reactions were ignored. This assumption gives the upper limits of the influence.) | ||||
• | The γ-ray pulse-height spectrum of the fourth origin is the same as that of the blank measurement, because events measured in the blank measurement are mainly due to neutrons that are scattered by the helium gas and captured by materials other than the samples. In the spectrum of the blank measurement, 478-keV γ-rays and prompt γ-rays from 27Al(n, γ) 28Al reactions are clearly observed. |
Using these assumptions and the net spectra of the first resonances of 244Cm and 246Cm, the ratio was calculated by the following equation:
5.1.2. Uncertainties at the first resonances of 244Cm and 246Cm
Contributions from uncertainties that were not dependent on the neutron energy are listed in . shows the total uncertainties and main contributions from the error sources to the uncertainties for 244Cm around its first resonance, and shows those for 246Cm. Because the ratio of the number of 240Pu nuclei to the sum of the curium isotopes in the 246Cm sample (listed in ) was normalized to the values of 244Cm, uncertainty in the isotopic ratio of 246Cm to 244Cm was also taken into account in the analysis of the 246Cm sample. The uncertainties in the impurities of 240Pu are the largest at the first resonances of 244Cm and 246Cm. The total uncertainties in the obtained cross-sections are 5.8% at the energy of the first resonance of 244Cm and 6.6% at that of 246Cm.
Figure 25. The deduced neutron-capture cross-sections of 244Cm (black circles; contribution from error sources to the uncertainties of the cross-sections, total error: red solid line; the abundances of 240Pu: black solid line; the evaluated value of JENDL-4.0 [16] of the first resonance of 240Pu: black dashed line; the contribution of fission events: blue dashed line; statistic: green solid line; self-shielding and multiple scattering: green dashed line; and systematic one in the normalization: purple solid line) around the first resonance of 244Cm.
![Figure 25. The deduced neutron-capture cross-sections of 244Cm (black circles; contribution from error sources to the uncertainties of the cross-sections, total error: red solid line; the abundances of 240Pu: black solid line; the evaluated value of JENDL-4.0 [16] of the first resonance of 240Pu: black dashed line; the contribution of fission events: blue dashed line; statistic: green solid line; self-shielding and multiple scattering: green dashed line; and systematic one in the normalization: purple solid line) around the first resonance of 244Cm.](/cms/asset/0ddb0d99-e384-4b11-80d1-b26f2e82d459/tnst_a_693887_o_f0025g.jpg)
Figure 26. The deduced neutron-capture cross-sections of 246Cm (black circles; contribution from error sources to the uncertainties of the cross-sections, total error: red solid line; the abundances of 240Pu: black solid line; the evaluated value of JENDL-4.0 [16] of the first resonance of 240Pu: black dashed line; isotopic ratio of 244Cm to 246Cm: blue solid line; the contribution of fission events: blue dashed line; statistic: green solid line; self-shielding and multiple scattering: green dashed line; and systematic one in the normalization: purple solid line) around the first resonance of 246Cm.
![Figure 26. The deduced neutron-capture cross-sections of 246Cm (black circles; contribution from error sources to the uncertainties of the cross-sections, total error: red solid line; the abundances of 240Pu: black solid line; the evaluated value of JENDL-4.0 [16] of the first resonance of 240Pu: black dashed line; isotopic ratio of 244Cm to 246Cm: blue solid line; the contribution of fission events: blue dashed line; statistic: green solid line; self-shielding and multiple scattering: green dashed line; and systematic one in the normalization: purple solid line) around the first resonance of 246Cm.](/cms/asset/de1a422d-fafc-4600-8141-94c8416bd824/tnst_a_693887_o_f0026g.jpg)
Table 4. Contributions to the non-neutron-energy-dependent errors in this work.
5.2. Comparison to the data given by Moore and JENDL-4.0
The capture cross-sections around the 7.7- and 16.8-eV resonances of 244Cm and the 4.3- and 15.3-eV resonances of 246Cm were obtained for the first time. The obtained cross-sections at the other resonances below 300 eV are consistent within 30% with the data given by Moore [4], as shown in and .
Of the obtained 244Cm cross-sections, the areas at the first, second, and fourth resonances are about 8, 13, and 9% smaller than the areas calculated with JENDL-4.0, respectively, whereas the area at the third resonance is about 9% larger. Of the obtained 246Cm cross-sections, the areas at the first and second resonances are about 7 and 10% smaller than the areas calculated with JENDL-4.0, respectively. It should be noted that the resonance at 131.0 eV listed in JENDL 4.0 was not observed, although the resonances at around 91.8, 158.4, and 193.5 eV were clearly visible.
6. Conclusion
The neutron-capture cross-sections of 244Cm and 246Cm were measured in the neutron energy range of 1–300 eV with the array of large Ge detectors in the ANNRI at J-PARC/MLF. The cross-sections were obtained by normalizing the relative yields to the values in JENDL-4.0 at the first resonance of 240Pu. The resonances of 244Cm at around 7.7 and 16.8 eV and of 246Cm at around 4.3 and 15.3 eV were observed in the capture reactions for the first time. The uncertainties of the obtained cross-sections are 5.8% at the energy of thefirst resonance of 244Cm and 6.6% at that of 246Cm. The resonance parameters of several low-energy ones were extracted from the cross-sections using the SAMMY code. The pulse-height spectra of the neutron-capture prompt γ-rays, which are emitted following the capture reactions by the 7.661-eV resonance of 244Cm and the4.314-eV resonance of 246Cm, were also obtained by gating the respective TOF regions. Eight new prompt γ-rays emitted in the 244Cm(n, γ) reaction were observed, as were five in the 246Cm(n, γ) reaction.
The present results gave accurate data for the neutron-capture cross-sections of 244Cm and 246Cm in the resonance region. This achievement also shows the effectiveness of the ANNRI for the measurement of neutron-capture cross-sections of highly radioactive samples.
Acknowledgements
This study includes the result of “Study on nuclear data by using a high intensity pulsed neutron source for advanced nuclear system” entrusted to Hokkaido University by the Ministry of Education, Culture, Sports, Science and Technology of Japan (MEXT). This work is supported by JSPS KAKENHI (22226016 and 22760675).
Additional information
Notes on contributors
Shinji Goko
†Present address: Japan Nuclear Energy Safety Organization, Toranomon 4-1-28, Minato-ku, Tokyo, 105-0001, Japan ‡Present address: Kyushu University, 6-1 Kasuga-kouen Kasuga, Fukuoka, 816-8580, JapanReferences
- Aliberti , G. , Palmiotti , G. , Salvatores , M. , Kim , T.K. , Taiwo , T.A. , Anitescu , M. , Kodeli , I. , Sartori , E. , Bosq , J.C. and Tommasi , J. 2006 . Nuclear data sensitivity, uncertainty and target accuracy assessment for future nuclear systems . Ann. Nucl. Energy , 33 : 700 – 733 .
- Firestone , R. 1999 . Table of Isotopes (1999 Update with CD-ROM), , 8th ed. , New York : John Wiley & Sons . ISBN 0471356336.
- Hagura , N. and Yoshida , T. 2010 . Analysis of prediction accuracy for actinide decay heat of spent MOX fuel from light water reactors [in Japanese] . Nihon- Genshiryoku-Gakkai Shi (J. At. Energy Soc. Jpn.) , 9 : 29 – 39 .
- Moore , M.S. and Keyworth , G.A. 1971 . Analysis of the fission and capture cross sections of the curium isotopes . Phys. Rev. C , 3 : 1656 – 1667 .
- Oshima , M. , Hori , J. , Harada , H. , Furutaka , K. , Koizumi , M. , Kitatani , F. , Toh , Y. , Kimura , A. , Nakamura , S. , Igashira , M. , Mizumoto , M. , Ohsaki , T. , Katabuchi , T. , Nishiyama , J. and Goto , J. Performance of a 4 π Ge Spectrometer for Neutron Capture Cross Section Measurements . Proceedings of the International Conference on ND 2007, Nice . April 22–27 2007 . pp. 603 – 606 .
- Kiyanagi , Y. , Kino , K. , Furusaka , M. , Hiraga , F. , Kamiyama , T. , Kato , K. , Igashira , M. , Katabuchi , T. , Mizumoto , M. , Oshima , M. , Harada , H. , Katakura , J. , Furutaka , K. , Goko , S. , Kimura , A. , Kin , T. , Kitatani , F. , Koizumi , M. , Nakamura , S. , Ohta , M. , Toh , Y. , Ohtsuki , T. , Hirose , K. , Fujii , T. , Hori , J. , Takamiya , K. , Fukutani , S. , Shibata , M. , Yamada , K. and Utsunomiya , H. 2011 . The ‘study on nuclear data by using a high intensity pulsed neutron source for advanced nuclear system’ nuclear data project and the characteristics of the neutron beam line for the capture cross section experiments at J-PARC . J. Korean Phys. Soc , 59 : 1781 – 1784 .
- Igashira , M. , Kiyanagi , Y. and Oshima , M. 2009 . Nuclear data study at J-PARC BL04 . Nucl. Instr. Meth. Phys. Res. A , 600 : 332 – 334 .
- Kin , T. , Furutaka , K. , Goko , S. , Harada , H. , Igashira , M. , Kamiyama , T. , Katabuchi , T. , Kimura , A. , Kino , K. , Kitatani , F. , Kiyanagi , Y. , Koizumi , M. , Mizumoto , M. , Nakamura , S. , Ohta , M. , Oshima , M. and Toh , Y. Development of a 4 π Germanium Spectrometer for Nuclear Data Measurements at J-PARC . The 2009 NSS-MIC Conference Record, Orland, USA . October 25–31 2009 . pp. 1194 – 1197 .
- Kimura , A. , Toh , Y. , Koizumi , M. , Osa , A. , Oshima , M. , Goto , J. and Igashira , M. Development of a Data Acquisition System for a Multiple Gamma-Ray Detection Method . International Conference on ND 2004, Santa Fe, USA, 27 September–1 October 2004, AIP Conference Proceedings Vol. 769 . pp. 792 – 795 .
- Kimura , A. , Toh , Y. , Koizumi , M. , Furutaka , K. , Kin , T. and Oshima , M. Performance of a High Speed and High Density Data Acquisition System for Multiple Gamma-Ray Detection . NSS-MIC 2008, Dresden . October 19–25 2008 . pp. 2107 – 2111 .
- Kimura , A. , Furutaka , K. , Goko , S. , Harada , H. , Kin , T. , Kitatani , F. , Koizumi , M. , Nakamura , S. , Ohta , M. , Oshima , M. , Toh , Y. , Fujii , T. , Fukutani , S. , Hori , J. , Takamiya , K. , Igashira , M. , Katabuchi , T. , Mizumoto , M. , Kamiyama , T. , Kino , K. and Kuyanagi , Y. 2011 . Measurements of neutron-capture cross sections of 244Cm and 246Cm at J-PARC/MLF/ANNRI . J. Korean Phys. Soc , 59 : 1828 – 1831 .
- Goko , S. , Kimura , A. , Harada, M. Oshima , H. , Ohta , M. , Furutaka , K. , Kin , T. , Kitatani , F. , Koizumi , M. , Nakamura , S. , Toh , Y. , Igashira , M. , Katabuchi , T. , Mizumoto , M. , Kiyanagi , Y. , Kino , K. , Furusaka , M. , Hiraga , F. , Kamiyama , T. , Hori , J. , Fujii , T. , Fukutani , S. and Takamiya , K. 2010 . Measurement of neutron capture cross section ratios of 244Cm resonances using NNRI . J. Nucl. Sci. Technol , 47 : 1097 – 1100 .
- Maekawa , F. , Harada , M. , Oikawa , K. , Teshigawara , M. , Kai , T. , Meigo , S. , Ooi , M. , Sakamoto , S. , Takeda , H. , Futakawa , M. , Kato , T. , Ikeda , Y. , Watanabe , N. , Kamiyama , T. , Torii , S. , Kajimoto , R. and Nakamura , M. 2010 . First neutron production utilizing J-PARC pulsed spallation neutron source JSNS and neutronic performance demonstrated . Nucl. Instr. Meth. Phys. Res. A , 620 : 159 – 165 .
- Kino , K. , Furusaka , M. , Hiraga , F. , Kamiyama , T. , Kiyanagi , Y. , Furutaka , K. , Goko , S. , Harada , H. , Harada , M. , Kai , T. , Kimura , A. , Kin , T. , Kitatani , F. , Koizumi , M. , Maekawa , F. , Meigo , S. , Nakamura , S. , Ooi , M. , Ohta , M. , Oshima , M. , Toh , Y. , Igashira , M. , Katabuchi , T. and Mizumoto , M. 2011 . Measurement of energy spectra and spatial distributions of neutron beams provided by the ANNRI beamline for capture cross-section measurements at the J-PARC/MLF . Nucl. Instr. Meth. Phys. Res. A , 626 : 58 – 66 .
- Fujii , T. , Hori , J. and Fukutani , S. Study on Nuclear Data by using a High Intensity Pulsed Neutron Source for Advanced Nuclear System (4) Analysis of Components of the Samples for Nuclear Data [in Japanese] . Conference record of 2010 Annual Meeting of AESJ . March 26–28 2010 . pp. 95 [CD-ROM] .
- Shibata , K. , Iwamoto , O. , Nakagawa , T. , Iwamoto , N. , Ichihara , A. , Kunieda , S. , Chiba , S. , Furutaka , K. , Otuka , N. , Ohsawa , T. , Murata , T. , Matsunobu , H. , Zukeran , A. , Kamada , S. and Katakura , J. 2011 . JENDL-4.0: a new library for nuclear science and engineering . J. Nucl. Sci. Technol , 48 : 1 – 30 .
- Kimura , A. , Furutaka , K. , Goko , S. , Harada , H. , Igashira , M. , Kamiyama , T. , Katabuchi , T. , Kin , T. , Kino , K. , Kitatani , F. , Kiyanagi , Y. , Koizumi , M. , Mizumoto , M. , Nakamura , S. , Ohta , M. , Oshima , M. and Toh , Y. A Dead-Time Correction Method for Multiple Gamma-ray Detection . The 2009 NSS-MIC Conference Record . October 25–31 2009 . pp. 138 – 143 .
- Ardisson , G. , Koua , A.A. , Barci , V. , Marsol , C. , Barci-Funel , G. and David , F. 1997 . 245Cm states fed by the α-decay 249Cf . Radiochim. Acta , 78 : 15
- Ahmad , I. , Sharma , D.D. and Sjoblom , R.K. 1976 . Electron capture decay of 245Bk (4.90 d) and 246Bk (1.80 d) . Nucl. Phys. A , 258 : 221 – 236 .
- Bunker , M.E. , Hoffman , D.C. , Orth , C.J. and Starner , J.W. 1967 . Decay of 245Am . Nucl. Phys. A , 97 : 593 – 599 .
- Braid , T.H. , Chasman , R.R. , Erskine , J.R. and Friedman , A.M. 1971 . (d,p) and (d,t) Studies of the actinide elements. II. 243Cm, 245Cm, 247Cm, and 249Cm . Phys. Rev. C , 4 : 247 – 262 .
- Kin , T. , Furutaka , K. , Goko , S. , Harada , H. , Kimura , A. , Kitatani , F. , Nakamura , S. , Ohta , M. , Oshima , M. , Toh , Y. , Hori , J. , Igashira , M. , Katabuchi , T. , Koizumi , M. , Mizumoto , M. , Kamiyama , T. , Kino , K. and Kiyanagi , Y. 2011 . The “4 π Germanium spectrometer” for measurements of neutron capture cross sections by the time-of-flight method at the J-PARC/MLF/ANNRI . J. Korean Phys. Soc , 59 : 1769 – 1772 .
- J.F. Briesmeister (ed.), MCNP-A General Monte Carlo N-Particle Transport Code, Version 4C, LA-13709-M, Los Alamos National Laboratory, Los Alamos, 2000.
- Ohta , M. , Oshima , M. and Kitatani , F. Measurement of Neutron Spectrum at BL04 of J-PARC/MLF [in Japanese] . Conference Record of 2009 Fall Meeting of AESJ . September 16–18 2009 . pp. 465 [CD-ROM] .
- Macklin , R.L. and Gibbons , J.H. 1967 . Capture-cross-section studies for 30—220-keV neutrons using a new technique . Phys. Rev , 159 : 1007 – 1012 .
- Larson , N.M. 2006 . Updated Users' Guide for SAMMY: Multilevel R-Matrix Fits to Neutron Data Using Bayes ′ Equations , ORNL/TM-9179/R7 , Oak Ridge , TN : Oak Ridge National Laboratory .
- Harada , H. , Goko , S. , Kimura , A. , Ohta , M. , Oshima , M. , Kitatani , F. , Toh , Y. , Furutaka , K. , Kin , T. , Koizumi , M. , Nakamura , S. , Igashira , M. , Katabuchi , T. , Mzumoto , sM. , Ohsaki , T. , Hori , J. , Fujii , T. , Takamiya , K. , Goto , J. , Kiyanagi , Y. , Kino , K. , Furusaka , M. , Hiraga , F. and Kamiyama , T. 2011 . Study of neutron capture reactions using the 4 π Germanium spectrometer . J. Korean Phys. Soc , 59 : 1547 – 1552 .