Abstract
Denitration of a highly concentrated sodium nitrate (NaNO3) aqueous solution via a catalytic reduction method using a palladium–copper catalyst supported on carbon powder (Pd–Cu/C) and hydrazine (N2H4) was investigated. It was demonstrated that nitrate ion (NO3 −) in a 5 mol L−1 NaNO3 solution was completely reduced through an intermediate nitrite ion (NO2 −) to nitrogen compounds such as nitrogen, nitrous oxide, and ammonia. By comparing the reaction rates of NO3 − and NO2 − obtained using catalysts with various Pd–Cu compositions and different reductants (hydrogen (H2) or N2H4), it was determined that the catalyst with a molar ratio of Pd:Cu = 1:0.66 provides the maximum reaction rates for NO3 − and NO2 − using N2H4, and that not only the reactions of NO3 − and NO2 − but also that of N2H4 were affected by the Pd–Cu composition.
1. Introduction
Low-level radioactive liquid wastes containing highly concentrated nitrate salts are generated from the operation of nuclear-fuel reprocessing facilities [Citation1]. When the liquid waste is solidified with cement without denitration treatment and then disposed; first the solidified substance comes in contact with groundwater, and then the nitrates in the substance dissolve in the groundwater and diffuse into surrounding environment. Because nitrate ion (NO3 −) is an environmental pollutant [Citation2] and is included in the waste before solidification, its removal is effective for preventing its diffusion into the environment from the waste. Therefore, a nitrate removal method is required to efficiently remove NO3 − of high concentration from a large amount of waste and reduce the amount of radioactive waste produced. It has been reported that NO3 − is reduced via a catalytic reduction method using a bi-metal catalyst such as palladium–copper (Pd–Cu) and a reductant such as hydrogen gas (H2) [Citation3 Citation Citation Citation Citation Citation Citation Citation Citation Citation Citation Citation Citation Citation–Citation17], and this method has a possibility of meeting such requirements. However, because this method was mainly studied for the denitration of low-concentrated nitrate salt solutions, the applicability of the method to the denitration of highly concentrated nitrate salt solutions must be confirmed.
Since Vorlop and Tacke [Citation4] reported a reduction method for low concentrations of NO3 − using supported Pd–Cu as the catalyst and H2 as the reductant, many studies have been conducted [Citation3 Citation Citation Citation Citation Citation Citation Citation Citation Citation Citation Citation Citation Citation–Citation17]. The mechanism of NO3 − reduction with a Pd–Cu catalyst and H2 reductant has been studied, and it was deduced that NO3 − is reduced through the nitrite ion (NO2 −) to nitrous oxide (N2O), nitrogen (N2), or ammonia (NH3) via hydrogen adsorbed on the catalyst surface [Citation6,Citation11,Citation14,Citation16,Citation17]. Ilinitch et al. [Citation14] reported that the maximum reaction rate for NO3 − was achieved using a catalyst with a molar ratio of Pd:Cu = 1:0.8, and that the maximum reaction rate of NO2 − was observed with a Pd monometallic catalyst.
Nitrate ion at 200 ppm was completely reduced by a Pd–Cu catalyst and H2 reductant [Citation11]. However, when H2 is used in the denitration of highly concentrated nitrate salt solutions, for example, 5 mol L−1 sodium nitrate (NaNO3), it is guessed that the reaction rate will be slow because the concentration of H2 in the solution is low. To increase the concentration of the reductant, the use of a water-soluble reductant is effective. Formaldehyde (HCHO); formic acid (HCOOH); hydrazine (N2H4); and reductants including boron, sulfur, phosphorus, and other metal ions are available as water-soluble reductants. However, given that the liquid wastes will be solidified with cement and then disposed, the products of the denitration reaction must not be highly corrosive and should not include any environmentally harmful substances or metal salts. Therefore, reductants including boron, phosphorus, and other metals are not suitable. When HCHO or HCOOH is used, they are oxidized to carbonate and the carbonate salts are precipitated in the denitration of highly concentrated nitrate salt solutions. With a reductant including sulfur, there is a concern that the catalyst will be poisoned by sulfur compounds [Citation18]. Hydrazine, which produces N2, is thus a suitable reductant.
In the present study, NO3 − in a 5 mol L−1 NaNO3 aqueous solution was reduced using a Pd–Cu catalyst and hydrazine monohydrate (N2H4 · H2O) reductant at 333 K. The denitration performance of the N2H4 was compared with that of H2. The reaction rates of NO3 − and NO2 − were determined with catalysts having various Pd–Cu compositions, and the effect of the Pd–Cu composition on the reactivities of not only NO3 − and NO2 − but also N2H4 was discussed.
2. Experimental
2.1. Preparation and characterization of the catalysts
Pd–Cu catalysts supported on carbon powder (Pd–Cu/C) were prepared by an electroless method as follows. Palladium dichloride (PdCl2, Sigma-Aldrich Co., LLC, 99%), copper dichloride (CuCl2, Kojundo Chemical Laboratory Co., Ltd., 4N), and carbon powder (Kojundo Chemical Laboratory Co., Ltd., 2N7) were used. The carbon powder was suspended in a mixed solution of PdCl2 (0–0.016 mol L−1), CuCl2 (0–0.013 mol L−1), and 0.01 mol L−1 hydrochloric acid (Wako Pure Chemical Industries, Ltd.), and then disodium ethylenediaminetetraacetate (Nacalai Tesque, Inc., 99.5%) was added to achieve 0.1 mol L−1 in the suspension with stirring at 333 K. Next, the pH of the suspension was adjusted to 10 using a HORIBA F53 pH meter by dropwise adding a 5 mol L−1 sodium hydroxide (Koso Chemical Co., Ltd., 96%) solution. Then, a solution containing an equimolar amount (relative to the metals) of sodium borohydride (Wako Pure Chemical Industries, Ltd., 90%) was added to the suspension. The carbon powder in the suspension was recovered by suction filtration, washed, and then dried at 333 K. Catalysts with the composition 0.47 mmol g−1 Pd–x mmol g−1 Cu/C (x = 0–0.39) and y mmol g−1 Pd–0.39 mmol g−1 Cu/C (y = 0–0.47) were prepared. Distilled and deionized water was used in the preparation of the catalysts. The naming convention used in this paper for the catalysts with various Pd–Cu compositions is shown by the following example: 0.47 mmol g−1 Pd–0.39 mmol g−1 Cu/C is denoted as 0.47 Pd–0.39 Cu/C.
The specific surface area of the carbon powder was measured using a SHIMADZU Gemini V surface area analyzer. The total metal contents of the catalysts were evaluated in following way: Pd and Cu of the 0.47 Pd–0.39 Cu/C were dissolved in an aqua regia, and then the Pd and Cu concentrations in the solution were determined using a SHIMADZU ICPS-8000E inductively coupled plasma atomic emission spectrometer (ICP-AES). The X-ray diffraction (XRD) patterns of the prepared Pd–Cu/C were also measured using a Rigaku MiniFlexII X-ray diffractometer.
2.2. Procedures
2.2.1. Denitration
Aqueous solutions containing NO3 − or NO2 − were prepared using NaNO3 (Wako Pure Chemical Industries, Ltd., 99.0%) or sodium nitrite (NaNO2, Wako Pure Chemical Industries, Ltd., 98.5%), respectively. An apparatus for denitration is shown in . The apparatus consisted of a four necked Pyrex glass 500 mL separable flask in a water bath, a magnetic stirrer, a measuring cylinder, a peristaltic pump, a tygon tube, a condenser tube, and a recirculating cooler. Two grams of 0.47 Pd–0.31 Cu/C and 100 mL of 5 mol L−1 NaNO3 solution were placed in the flask. The suspension was stirred and maintained at 333 K by heating with the water bath. Forty milliliters of N2H4 · H2O solution (Sigma-Aldrich Co., LLC, 98%) was then added by drops into the suspension for 0.5 h.
Figure 1. An apparatus for denitration experiment. (a) A four necked separable flask, (b) a water bath, (c) a underwater magnetic stirrer, (d) a measuring cylinder, (e) a peristaltic pump, (f) a tygon tube, (g) a condenser tube, and (h) a recirculating cooler.
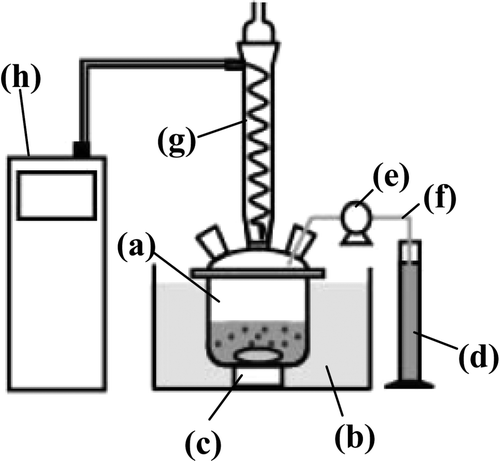
In a second experiment, 0.2 g of the 0.47 Pd–0.39 Cu/C and 100 mL of 5 × 10−3 mol L−1 or 0.5 mol L−1 NaNO3 solution were placed in the flask, and then the suspension was stirred and maintained at 333 K. The flow of H2 (TOMOE SHOKAI Co., Ltd., 99.99%) into the suspension was maintained at a constant rate of 30 mL min−1. Additional experiments using 0.2 g of 0.47 Pd/C, 0.47 Pd–0.39 Cu/C, or 0.39 Cu/C and 5 × 10−3 mol L−1 of NaNO2 solution with H2 were also conducted using the abovementioned procedure.
In a third experiment, 0.2 g of a Pd–Cu/C catalyst and 100 mL of 0.5 mol L−1 NaNO3 or NaNO2 solution were placed in the flask. The suspension was stirred and maintained at 333 K. Four milliliters of N2H4 · H2O was then rapidly added. The catalysts used in these experiments included 0.47 Pd–x Cu/C (x = 0−0.39) and y Pd–0.39 Cu/C (y = 0−0.47).
2.2.2. Analysis
To analyze the reactions, a portion of the suspension was sampled, followed by filtration and dilution. The concentrations of NO3 −, NO2 −, and N2H4 in the diluted solutions were determined using a DIONEX (now THERMO SCIENTIFIC) ICS-1000 ion chromatographic apparatus. The pH value of the suspension was measured using a pH meter. The generated gas was collected and the component of the gas was analyzed using a HITACHI263-50 gas chromatography. The concentrations of the Pd and Cu ions in the suspension after denitration were determined by ICP-AES.
3. Results and discussion
3.1. Characterization of the catalysts
The Pd–Cu/C was prepared using an electroless method. The specific surface area of the carbon powder support was determined to be 13.57 m2 g−1. The metals supported on the carbon powder were dissolved in an aqua regia, and the concentrations of the Pd and Cu in the solution were determined. For this determination, the 0.47 Pd–0.39 Cu/C catalyst with the highest supported ratio of the prepared catalysts was used. The results are summarized in together with the calculated concentrations of the Pd–Cu/C based on the preparation conditions. The found ratios of Pd and Cu were 0.46 and 0.37 mmol g−1, respectively, which are in good agreement with the calculated values. This result suggests that nearly all the metal ions used for the catalyst preparation were deposited on the carbon powder.
Table 1. Calculated and found ratios for the Pd and Cu supported on carbon powder.
shows the XRD patterns of the carbon powder and the 0.47 Pd–x Cu/C (x = 0−0.39) and y Pd–0.39 Cu/C (y = 0–0.47) catalysts. Three peaks were observed for the Pd/C in (b). The peak at 2θ = 40.02° was attributed to Pd(111) and those at 42.43° and 44.55° were attributed to graphite [Citation19,Citation20]. The Pd(111) peak shifted with an increase in the Cu fraction of the catalyst. The Pd(111) peak appeared at 2θ = 40.35°, 40.57°, 40.66°, 40.84°, and 40.88° for the catalysts with Cu fractions of 0.14, 0.25, 0.40, 0.46, and 0.51, respectively. These shifts of the Pd(111) peak positions indicate the formation of an alloy of the Pd with the Cu having different lattice parameters [Citation21]. In addition, the Pd(111) peak intensity decreased with an increase in the Cu fraction in the catalyst when the Pd concentration remained constant. This decrease in the peak intensity is likely caused by a decrease in the crystallinity of the Pd–Cu alloy ((b)–(e)). Other peaks were observed for the catalysts with a Cu fraction greater than 0.68 ((h)–(j)). These peaks were attributed to Cu oxide [Citation22,Citation23]. No peaks for Cu metal were observed in the catalysts having a high-Cu fraction, because the Cu(111) peak appears at approximately 2θ = 43.3°, which overlaps with the C peaks [Citation24].
3.2. Denitration
3.2.1. Denitration of a highly concentrated NaNO3 solution using the Pd–Cu catalysts and N2H4
Nitrate ion in a 5 mol L−1 NaNO3 solution (100 mL) was reduced using the 0.47 Pd–0.31 Cu/C catalyst (2 g) suspended in the NaNO3 solution, and 40 mL of N2H4 · H2O was added by drops for 0.5 h. The variation in the concentration of NO3 −, NO2 −, and N2H4 in the suspension as a function of time was determined, and the results are plotted in . The concentration of NO3 − decreased from 5 mol L−1 to 0.33 mol L−1 after 0.5 h and fell below 1 × 10−3 mol L−1 after 4 h. Nitrite ion was newly detected in the suspension, suggesting that the denitration proceeds through NO2 − as an intermediate. The concentration of NO2 − increased to 1.8 mol L−1 after 0.25 h and decreased to 6.6 × 10−3 mol L−1 after 7 h. The concentration of N2H4 was below the detection limit until 0.25 h, reached 3.7 mol L−1 at 0.5 h, and then decreased to 0.45 mol L−1 after 7 h. From these changes in the concentration of NO3 −, NO2 −, and N2H4 with time, it was assumed that the reaction of NO3 − with N2H4 proceeds more rapidly than the addition rate of N2H4 · H2O, but that the reaction of NO2 − with N2H4 was slower than that. The pH of the suspension rose to 14 for 7.5 min and 14.6 after 7 h. About 99.9% of NO3 − was removed with this method, and thus it was determined that the use of a Pd–Cu catalyst and N2H4 is applicable for the denitration of highly concentrated nitrate salt solutions.
Figure 3. Concentrations of NO3 −, NO2 −, and N2H4 in suspension as a function of time. Reaction solution: 100 mL of 5 mol L−1 NaNO3, catalyst: 2 g of 0.47 Pd–0.31 Cu/C, reductant: 40 mL of N2H4 · H2O, drop rate of N2H4 · H2O: 80 mL h−1, temperature: 333 K. •: NO3 −, ○: NO2 −, □: N2H4.
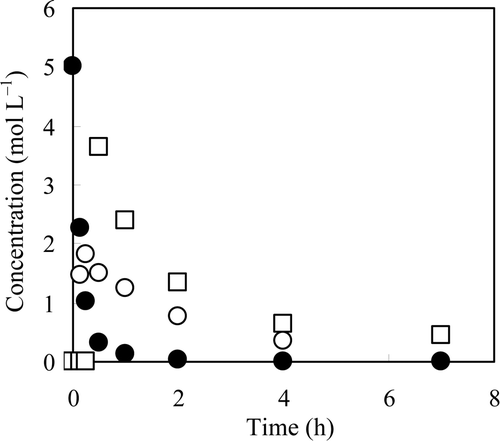
However, NO3 − was not reduced by mixing the NaNO3 solution and N2H4 · H2O in the absence of the catalyst at 333 K. Therefore, it was confirmed that the denitration reaction proceeds via a catalytic reaction. In addition, after completion of the denitration reaction, no dissolved Pd or Cu ions from the catalyst were found in the suspension. This result indicated that the catalyst was not corroded during the reaction with N2H4 in a highly concentrated NO3 − solution.
The mechanism of NO3 − reduction with Pd–Cu catalysts and H2 has been widely studied, and it has been deduced that NO3 − is reduced through NO2 − to N2, N2O, and NH3 via reaction with hydrogen adsorbed on the catalyst surface [Citation6,Citation11,Citation14,Citation16,Citation17]. In the present study, a Pd–Cu catalyst was also used, and N2, N2O, and NH3 were produced. In addition, it has been reported that the adsorbed hydrogen is generated by decomposition of N2H4 on a Pd or Cu surface [Citation25 Citation–Citation27]. Therefore, in the reduction using N2H4, NO3 − is likely reduced through the same mechanism as that used for the reduction using H2.
3.2.2. Comparison of H2 with N2H4
Nitrate ion in a 5 × 10–3 mol L−1 NaNO3 solution (100 mL) was reduced using a 30 mL min−1 H2 flow in the presence of 0.47 Pd–0.39 Cu/C catalyst (0.2 g). Eighty percent of NO3 − in the suspension was reduced in 2 h. However, in the denitration of a 0.5 mol L−1 NaNO3 solution, the concentration of NO3 − did not decrease. Based on these results, it was concluded that H2 is unsuitable for the reduction of highly concentrated of NO3 − solutions, although H2 is applicable for the reduction of low-concentrated NO3 − solutions. On the other hand, NO3 − in a 0.5 mol L−1 NaNO3 solution (100 mL) was effectively reduced using N2H4 · H2O (4 mL) in the presence of 0.47 Pd–0.39 Cu/C (0.2 g). In this denitration reaction, 80% of the NO3 − was reduced within 1 h. This result demonstrated that N2H4 is more effective than H2 for the denitration of highly concentrated NaNO3 solutions.
Elliot et al. reported that the solubility of H2 at 343 K was 7.7 × 10−4 mol L−1 in water and 4.7 × 10−4 mol L−1 in a 2.0 mol L−1 lithium nitrate solution [Citation28]. Thus, the solubility of H2 was of the same order of magnitude regardless of whether the solution contained any salt. It is expected that the concentration of H2 in the present study would also be similar to the reported values. It has also been reported that NO3 − strongly adsorbs onto the Pd–Cu catalyst surface [Citation16], and H2 adsorption onto the catalyst surface may be blocked by the high concentration of adsorbed NO3 –. Therefore, it is presumed that the concentration of adsorbed H2 on the catalyst surface is too low owing to the combination of the low-solubilized H2 concentration and the defeat of H2 in adsorption competition with NO3 –. These factors may also be responsible for the reduced reaction rate of NO3 − in the reaction with H2 and the lack of a decrease in the NO3 − concentration in the above experiment. On the other hand, for the reaction with N2H4, the initial concentration of the reductant was 0.8 mol L−1, which is about 103 times higher than the concentration of H2, and was sufficient for the denitration of the 0.5 mol L−1 NaNO3 solution.
3.3. Reaction rate as a function of the Pd–Cu composition
The NO3 − present in a 0.5 mol L−1 NaNO3 solution (100 mL) was reduced using the 0.47 Pd–x Cu/C (x = 0−0.39) and y Pd–0.39 Cu/C (y = 0−0.47) catalysts (0.2 g) with N2H4 · H2O (4 mL). Similarly, the NO2 − in a 0.5 mol L−1 NaNO2 solution (100 mL) was also reduced. Then, in order to decrease the temperature change caused by the heat of reaction, 1/10th of the concentrations of both the NaNO3 and N2H4 reactants and the amount of catalyst were used for the denitration of a 5 mol L−1 NaNO3 solution, and also, the catalyst metal of the same quantity was used in all examinations without depending on difference in composition of the catalyst. In these reactions, the concentration of NO3 − and NO2 − decreased with time, and the rate of the decrease in the NO3 − concentration depended on the Pd–Cu composition of the catalyst. For example, the concentration of NO3 − decreased most rapidly using the 0.47 Pd–0.31 Cu/C catalyst, with 87% of NO3 − reduced in 1 h, while only 55% of NO3 − reduced in 1 h using the 0.47 Pd–0.16 Cu/C catalyst. Here, the reaction rate of NO3 − reduction was defined as the amount of NO3 − reduced per unit time and unit amount of Pd–Cu, and was calculated using the following procedure. First, an average slope for the initial 0.25 h of the reaction was determined from a graph of the amount of reduced NO3 − versus time, and the average slope was divided by the amount of Pd–Cu in the catalyst used for the reaction. The reaction rate of NO2 − reduction was also calculated using a similar procedure.
The reaction rates of NO3 − and NO2 − are plotted in as a function of the mole fraction of Cu in the catalyst. This figure shows the results obtained for the catalysts with a Cu fraction ranging from 0 to 1, i.e., the catalysts with 0.47 Pd–x Cu/C (x = 0−0.39) and y Pd–0.39 Cu/C (y = 0−0.47). Nitrate ion was not reduced by the Pd monometallic catalyst, but NO3 − was reduced by the Pd–Cu catalysts (). The reaction rate of NO3 − increased with the Cu fraction and reached a maximum at a Cu fraction of approximately 0.4, and then decreased with an increase in the Cu fraction in the range of 0.4–1. With the Cu monometallic catalyst, NO3 − was reduced. These results clarified that Cu is indispensable for the reduction of NO3 −. It has been reported that the reduction of NO3 − to NO2 − is initiated by the Cu sites, which are then converted to Cu2O during the deoxidation of NO3 − [Citation14]. However, the formed Cu2O must then be reduced back to Cu by the spillover hydrogen generated mainly on the Pd sites, thus regenerating the Pd–Cu alloy. Therefore, the reaction rate of NO3 − increases with an increase in the Cu content in the low-Cu fraction range, but decreases with a decrease in the number of Pd sites in the high-Cu fraction range. In this denitration system, the catalyst with 0.47 Pd–0.31 Cu/C, which has a Cu fraction of 0.40, gives the best results for the deoxidation of NO3 − and the regeneration of the Cu, and thus showed the highest rate for the reduction of NO3 −. This Cu fraction is similar to the results obtained in some previous reports [Citation14,Citation17].
Figure 4. Reaction rates of NO3 − and NO2 − using several catalysts with different Cu fractions. Reaction solution: both 100 mL of 0.5 mol L−1 NaNO3 and NaNO2, catalyst: 0.2 g of 0.47 Pd–x Cu/C (x = 0−0.39) or y Pd–0.39 Cu/C (y = 0−0.47), reductant: 4 mL of N2H4 · H2O, temperature: 333 K. •: NO3 −, ○: NO2 −.
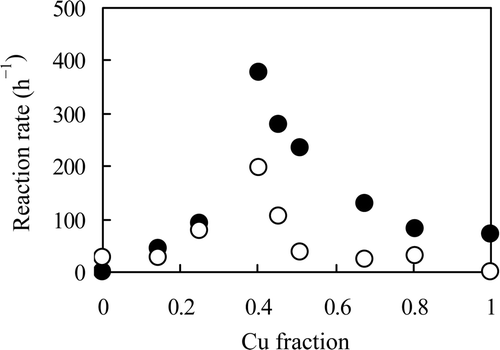
Reduction of NO2 − as the intermediate in the NO3 − reduction was also studied. It was confirmed that NO2 − is not reduced when a NaNO2 solution is mixed with N2H4 · H2O in the absence of the catalyst, as well seen with the NO3 − solution mentioned above. As shown in , NO2 − was reduced by the Pd monometallic catalyst and the Pd–Cu bi-metal catalyst, but was not reduced by the Cu monometallic catalyst. Therefore, it was confirmed that the NO2 − reduction was promoted by a catalytic reaction, and that Pd is indispensable for the reduction of NO2 −. The reaction rate of NO2 − reached a maximum when the catalyst with a Cu fraction of approximately 0.4 was used. This result is different from those described in earlier reports, in which the reaction rate of the NO2 − reduction by H2 reached a maximum when a catalyst with a low-Cu fraction was used [Citation10,Citation14,Citation17]. The decrease in the reaction rate of NO2 − with an increase in the Cu fraction () can be explained by the decrease in the Pd content if Pd serves as the NO2 − active site. The increase in the reaction rate of NO2 − () is discussed below.
The reaction rate of NO2 − was determined using H2 instead of N2H4. Here, 100 mL of 5 × 10−3 mol L−1 NaNO2 solution and 0.2 g of 0.47 Pd/C, 0.47 Pd–0.39 Cu/C, and 0.39 Cu/C were used. The relationship between the reaction rate of NO2 − and the Pd–Cu composition using H2 and N2H4 is summarized in . When Pd/C and Pd–Cu/C were used, the reaction rate of NO2 − was nearly the same as in the reduction with H2. Because NO2 − is mainly reduced on Pd surface, same reaction rates were observed for the catalysts with same Pd content. On the other hand, the rate with Pd–Cu/C was higher than that with Pd/C in the reduction with N2H4. This result indicates that the alloy of Pd with Cu strongly contributes to the increase in the N2H4 reactivity. Thus, the behavior of the NO2 − reaction rate () can be explained by the change in reactivity of the N2H4 with the change in the Cu fraction.
Table 2. Comparison of the reaction rate of NO2 − using H2 and N2H4.
We concluded the mechanism of the denitration reaction using the Pd–Cu catalyst and N2H4 as follows. At first, NO3 − was deoxidized to NO2 − on Cu and next NO2 − was reduced to N2O, N2, or NH3 on Pd. The adsorbed hydrogen needed for the NO3 − and NO2 − reduction was generated by decomposition of N2H4 on Pd and Cu surface. The alloying of Pd and Cu increased reactivity of not only NO3 − and NO2 − but also N2H4, and the reaction rates of NO3 − and NO2 − showed the maximum when the composition of Pd:Cu was 1:0.66, as a result. By using a water-soluble reductant like N2H4, enough amount of the reductant could adsorb on the catalyst surface in competitive adsorption with NO3 − in the suspension of highly concentrated NaNO3 solution and the Pd–Cu catalyst, and then the denitration proceeded rapidly. It was confirmed that the chemical reduction method using the Pd–Cu catalyst and the N2H4 reductant had a high applicability for the denitration of the highly concentrated NaNO3 solution.
4. Conclusion
A catalytic reduction method that is applicable for highly concentrated NaNO3 solutions was demonstrated using the combination of a Pd–Cu catalyst and N2H4. Highest reaction rates for NO3 − and NO2 − reduction were achieved using a catalyst with a metal composition of approximately Pd:Cu = 1:0.66, and not only the reaction rates of NO3 − and NO2 − but also the reactivity of N2H4 were affected by the formation of a Pd–Cu alloy. To apply this method for practical use, the durability of the catalyst should be further studied in order to determine the optimized conditions for enhancing the catalyst lifetime and thus decreasing the operating costs of the catalyst. In addition, the selectivity for N2O, N2, and NH3 should be studied to determine how to suppress the production of N2O and NH3 as a means for reducing the required off-gas treatment processes.
Additional information
Notes on contributors
Haruhiko Kadowaki
†Present address: Japan Atomic Energy Agency, 3 Myojin-cho, Tsuruga-shi, Fukui 914-8510, Japan.References
- Japan Atomic Energy Agency . 2007 . “ The Federation of Electric Power Companies of Japan ” . In Second progress report on research and development for TRU waste disposal in Japan , Japan Atomic Energy Agency and the Federation of Electric Power Companies of Japan . JAEA-Review 2007-010, FEPC TRU-TR2-2007-01
- Japan Environmental Management Association for Industry . 2009 . Shin kogaiboshinogijutsutohoki suishitsuhen I II , I-51 – I-56 . Tokyo : Maruzen Co. Ltd . (in Japanese)
- Hörold , S. , Vorlop , K.-D. , Tacke , T. and Sell , M. 1993 . Development of catalysts for a selective nitrate and nitrite removal from drinking water . Catal. Today , 17 : 21 – 30 .
- Vorlop , K.-D. and Tacke , T. 1989 . Erste Schritte auf dem Weg zur edelmetall-katalysierten Nitrat- und Nitrit-Entfernung aus Trinkwasser . Chem.-Ing.-Tech , 61 : 836 – 837 .
- Pintar , A. and Batista , J. 2007 . Catalytic stepwise nitrate hydrogenation in batch-recycle fixed-bed reactors . J. Hazard. Mater , 149 : 387 – 398 .
- Pintar , A. , Batista , J. , Levec , J. and Kajiuchi , T. 1996 . Kinetics of the catalytic liquid-phase hydrogenation of aqueous nitrate solutions . Appl. Catal. B , 11 : 81 – 98 .
- Gauthard , F. , Epron , F. and Barbier , J. 2003 . Palladium and platinum-based catalysts in the catalytic reduction of nitrate in water: effect of copper, silver or gold addition . J. Catal , 220 : 182 – 191 .
- Mikami , I. , Sakamoto , Y. , Yoshinaga , Y. and Okuhara , T. 2003 . Kinetic and adsorption studies on the hydrogenation of nitrate and nitrite in water using Pd–Cu on active carbon support . Appl. Catal. B , 44 : 79 – 86 .
- Fanning , J.C. 2000 . The chemical reduction of nitrate in aqueous solution . Coord. Chem. Rev , 199 : 159 – 179 .
- Sa , J. and Vinek , H. 2005 . Catalytic hydrogenation of nitrates in water over a bimetallic catalyst . Appl. Catal. B , 57 : 247 – 256 .
- Nakamura , K. , Yoshida , Y. , Mikami , I. and Okuhara , T. 2006 . Selective hydrogenation of nitrate in water over Cu–Pd/mordenite . Appl. Catal. B , 65 : 31 – 36 .
- Lemaignen , L. , Tong , C. , Begon , V. , Burch , R. and Chadwick , D. 2002 . Catalytic denitrification of water with palladium-based catalysts supported on activated carbons . Catal. Today , 75 : 43 – 48 .
- Maia , M.P. , Rodrigues , M.A. and Passos , F.B. 2007 . Nitrate catalytic reduction in water using niobia supported palladium–copper catalysts . Catal. Today , 123 : 171 – 176 .
- Ilinitch , O.M. , Nosowa , L.V. , Gorodetskii , V.V. , Ivanov , V.P. , Trukhan , S.N. , Gribov , E.N. , Bogdanov , S.V. and Cuperus , F.P. 2000 . Catalytic reduction of nitrate and nitrite ions by hydrogen: investigation of the reaction mechanism over Pd and Pd–Cu catalysts . J. Mol. Catal. A , 158 : 237 – 249 .
- Gao , W. , Guan , N. , Chen , J. , Guan , X. , Jin , R. , Zeng , H. , Liu , Z. and Zhang , F. 2003 . Titania supported Pd–Cu bimetallic catalyst for the reduction of nitrate in drinking water . Appl. Catal. B , 46 : 341 – 351 .
- Sakamoto , Y. , Kamiya , Y. and Okuhara , T. 2006 . Selective hydrogenation of nitrate to nitrite in water over Cu–Pd bimetallic clusters supported on active carbon . J. Mol. Catal , 250 : 80 – 86 .
- Yoshinaga , Y. , Akita , T. , Mikami , I. and Okuhara , T. 2002 . Hydrogenation of nitrate in water to nitrogen over Pd–Cu supported on active carbon . J. Catal , 207 : 37 – 45 .
- Yamanaka , T. 1965 . Shokubai kagaku zohoban , 126 – 132 . Tokyo : Nikkan Kogyo Shimbun Ltd . (in Japanese)
- JCPDS Alphabetical Index . Inorg. Phases, #46-1043
- JCPDS Alphabetical Index . Inorg. Phases, #56-0159
- Subramanian , P.R. and Laughlin , D.E. 1991 . Cu–Pd (Copper–Palladium) . J. Phase Equilibria , 12 : 231 – 243 .
- JCPDS Alphabetical Index . Inorg. Phases, #44-0706
- JCPDS Alphabetical Index . Inorg. Phases, #5-0667
- JCPDS Alphabetical Index . Inorg. Phases, #4-0836
- Vogt , K.W. , Naugher , L.A. and Kohl , P.A. 1995 . Low temperature nitridation of transition metals with hydrazine . Thin Solid Films , 256 : 106 – 115 .
- Kodera , T. , Honda , M. and Kita , H. 1985 . Electrochemical behaviour of hydrazine on platinum in alkaline solution . Electrochim. Acta , 30 : 669 – 675 .
- Fukumoto , Y. , Matsunaga , T. and Hayashi , T. 1981 . Electrocatalytic activities of metal electrodes in anodic oxidation of hydrazine in alkaline solution . Electrochim. Acta , 26 : 631 – 636 .
- Elliot , A.J. , Chenier , M.P. and Ouellette , D.C. 1990 . Solubilities of hydrogen and oxygen in concentrated lithium salt solutions . Fusion Eng. Des , 13 : 29 – 31 .