Abstract
The formation of volatile alkyl iodides other than methyl iodide during a serious nuclear reactor accident may have radiological significance. The hypothesis that radioactive alkyl iodides, other than methyl iodide, could form from paint solvents under the conditions of a serious nuclear accident in light water reactors (under boiling water reactor (BWR) and pressurised water reactor (PWR) conditions) was tested using stable elemental iodine, a gamma irradiator and gas chromatography equipment. It was found that methyl and isopropyl iodides were formed from the texanol ester, which is used in many modern water-based paints. Methyl, ethyl, propyl and butyl iodides were formed from a hydrocarbon solvent (white spirit) commonly used in paint products used in the past. These results suggest that further work on the formation and behaviour of the higher alkyl iodides (containing more than one carbon atom) under the conditions of a serious nuclear accident is justified.
1. Introduction
It is well known that iodine is one of the most critical fission products being released from UO2 fuel during a severe nuclear accident in light water reactors due to its mobility and radiotoxicity [Citation1]. However, the exact chemical forms of the iodine released during a serious accident is not known. While particles [Citation2] and elemental iodine can be trapped from gas phases, for example, using charcoal filters [Citation3], some volatile organic iodides, such as alkyl iodides, might not be removed from the gas phase by such means due to their different chemical behaviour. It is known that some alkyl halides are able to pass through a standard charcoal respirator filter, thus exposing the worker to compounds such as methyl bromide [Citation4]. The ability of charcoal to trap alkyl iodides may be improved by 1,4-diaza-bicyclo(2,2,2)octane (DABCO) [Citation5]. However, some alkyl iodides, such as 2-iodopropane, might fail to react with this additive as a steric effect retards the SN2 chemical reaction with DABCO [Citation6].
It has been shown that radioactive iodine in the form of organic methyl and ethyl iodide is converted into inorganic iodine in the human body, which is then accumulated in the thyroid gland [Citation7]. Thus, the form and formation of alkyl iodides during a serious nuclear accident is likely to have an effect on the radiological health of both the workers and the general public.
While it has been shown that alkyl iodides were formed during both the Chernobyl [Citation8] and the Three Mile Island [Citation9] accidents, it appears that many authors have assumed that the organic iodine is in the form of methyl iodide. For example, studies in which the reactions of organic iodine inside the containment of a damaged nuclear reactor [Citation10, Citation11] are investigated and studies of the organic iodine capture ability of emergency charcoal filters have used methyl iodide [Citation12, Citation13]. This assumption may not be completely correct and thus the methods to measure and prevent the release of alkyl iodides may be inappropriate.
In previous studies, commonly used paint solvents in different types of paint products used in different nuclear installations, have been identified at Chalmers Technical University of Technology [Citation14]. The main solvent in Teknopox Aqua VA epoxy paint (bisphenol-A based), which is used e.g. in the Swedish nuclear power plant Ringhals 2, is texanol ester (mono-2-methylpropionate esters of 2,2,4-trimethyl-1,3-pentanediol). While modern water-based paints typically use volatile oxygenated organics, the older paints often used hydrocarbon solvents such as white spirit (Stoddard solvent).
In this paper, common paint solvents, texanol ester and white spirit, were investigated towards their ability to form volatile organic iodides. Paint solvent solutions saturated with elemental iodine under anaerobic (BWR case) and aerobic (PWR case) conditions were exposed to intense gamma irradiation (Gammacell 220: 60Co, 14 kGy/h). The formed volatile organic iodides were identified using headspace gas chromatography (GC-MS). Texanol ester alcohol (isomer mixture; Aldrich, 99%) and two grades of white spirit were compared. One grade had a low content of aromatic hydrocarbons (DecoMax from Akzo Nobel), while the other one (Jotun) had a higher content of aromatic hydrocarbons (2–25%).
2. Experimental
2.1. Examination of Jotun and DecoMax white spirit
Preliminary gas chromatography measurements on the two studied commercially available white spirits (Jotun and DecoMax) were performed using a Varian 3350 gas chromatograph fitted with a flame ionisation detector and using hydrogen as carrier gas. The machine was fitted with a DB-23 column (15 m, 0.32 mm ID) with a 25-mm coating of a 50% cyanopropyl-methylpolysiloxane phase. Samples of the products were injected either directly in liquid form or 25 μl of its vapours which were released when heating for 5 minutes at 50 °C.
To characterise the white spirit solvents, dilute solutions of elemental iodine were examined with a Perkin Elmer spectrophotometer Lambda 19 (300–800 nm). New (iodine-free) samples of the white spirit solvents were diluted with cyclohexane before examination (225–400 nm) with the ultraviolet/visible (UV/Vis) spectrophotometer.
The white spirits consist of n-alkanes, isoalkanes, cycloalkanes and low amounts of aromatics containing between 10 and 13 carbon atoms. A dilute solution of iodine in DecoMax white sprit shows an absorption peak at 520 nm, while a similar solution of iodine in cyclohexane has an absorption peak at 523 nm. The absorption peak for iodine (209 nm) in Jotun white spirit was shifted towards the ultraviolet suggesting that substances with a π-system (aromatics) are present at a higher concentration in the Jotun white spirit [Citation15].
The white sprits contain aromatic compounds that absorb at 266 nm. Based on the UV absorption measurements at this wavelength, the Jotun white spirit has an aromatic content 140 times higher than that found in the DecoMax product. Further examination of concentrated solutions (10% v/v) of both white spirits suggests that the Jotun grade may contain traces of naphthalene (absorption peaks 319 and 311 nm) [Citation15] or a similar polycyclic aromatic hydrocarbon. These peaks for naphthalene could be observed in the spectrum of the DecoMax white spirit.
2.2. Radiolysis experiments
Iodine (1 g; Scharlau, >99.8%) was dissolved in 15 ml of the paint solvents. The concentration of the dissolved iodine was measured using a UV/Vis measurement at 350 nm after diluting the samples in a 1 M KI solution in ethanol. The amount of iodine was quantified with a calibration series between 10−1 and 10−6 mol/l elemental iodine in 1 mol/l KI–ethanol solutions. The concentrations of the iodine in the texanol ester, the DecoMax white spirit and the Jotun white spirit were 154, 174 and 171 mmol/l, respectively.
To be deoxygenated, iodine solutions (2 ml) were placed inside home-made borosilicate ampoules and subjected to two freeze-pump-thaw cycles using a vacuum line before sealing [Citation16]. The liquid in the ampoule was frozen using liquid nitrogen before the glass was sealed with a butane torch.
Oxygenated and oxygen-free samples were exposed to between 1.17 and 3700 kGy of 60Co gamma irradiation inside a Gammacell 220 at a dose rate of 14 kGy/h and at a temperature of 50 °C.
After irradiation, the iodine concentration in the aerobic iodine solutions was measured by UV/Vis spectrophotometry using the same method as described before.
To protect the gas chromatograph equipped with a mass spectrometer (GC-MS) the unreacted elemental iodine was removed from the white spirit samples by shaking with aqueous potassium iodide (1 mol/l) and if required they were diluted with ethanol before examination.
The deoxygenated samples were shaken with a saturated sodium chloride solution (brine) and with a sodium thiosulphate solution to remove acid and elemental iodine, respectively, before being dried over anhydrous magnesium sulphate (MgSO4) to remove traces of water. After removal of the drying agent, the samples were placed inside headspace vials and if not immediately used for analysis they were stored with a spiral of copper wire to stabilise the alkyl iodides.
The deoxygenated samples were examined in an initial experiment without using internal standards to determine what organic iodine compounds could form as a result of irradiation.
To the aerated samples (0.5 ml), an internal standard was added before the headspace vials were sealed with a crimp-on-septum lid. For the texanol ester samples, butyl iodide (20 μl; Aldrich, 99%) was used, while for the white spirit samples, iodobenzene (20 μl; Aldrich, 98%) was used as an internal standard.
The samples were heated (50 oC, 15 minutes) in a thermostated aluminium block before an air sample (20 μl) was transferred into a gas chromatography mass spectrometer (HP GCD fitted with a 15 m, 0.25 mm ID Zebron column with a 25 μm coating of ZB-5 ms {95% dimethylpolysiloxane and 5% phenyl/arylene}) (see ). The ionisation mode of the mass spectrometer was electron impact (70 eV) and the spectrometer was set to scan between 45 and 400 atomic mass units (amu).
Figure 1 Schematic of the GC-MS machine, a headspace vial heated in a heating block and the injection syringe
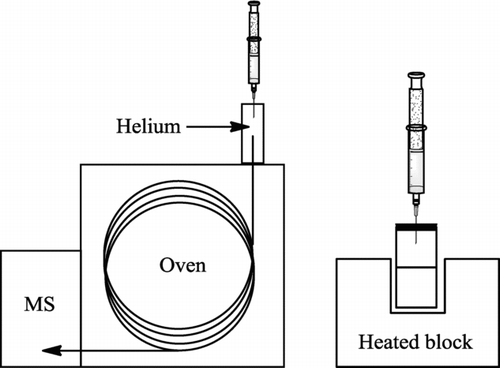
The GC-MS machine was calibrated for the expected alkyl iodides with headspace injections analogous to the sample measurements, using the paint solvents spiked with an internal standard. White spirit (Jotun) was calibrated with ethyl iodide (Fluka, 99%), methyl iodide (Aldrich, >99%), isopropyl iodide (Aldrich, 99%) and butyl iodide (Aldrich, 99%). Texanol ester (Aldrich, 99%) was calibrated for methyl and isopropyl iodide. The calibrated concentration range was between 10 and 100 mmol/l.
3. Results and discussion
While it was suggested that the use of aromatic paint solvents would minimise the formation of alkyl iodides during a serious reactor accident [Citation17], in recent years for health [Citation18], environmental and fire safety reasons, a movement from high volatile organic compound (VOC) petroleum-based paints to low VOC paints, which include water-based paints, has occurred. The water-based paints (e.g. Teknopox Aqua VA epoxy paint) typically contain oxygenated VOCs such as coalescence agents like texanol ester (see ), while the main VOCs in the petroleum-based paints were often aliphatic and aromatic hydrocarbons.
Figure 3 The two isomers of 2,2,4-trimethyl-1,3-pentanediol mono-2-methylpropinate ester (texanol ester) showing the proposed cleavage sites
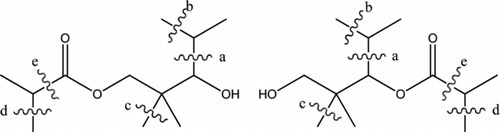
As the radiolysis of organic compounds can form free radicals, which can then react with elemental iodine to form organic iodine compounds, it is reasonable to assume that the structure of the original organic compound would have a great influence on the structure of the alkyl iodides formed during a nuclear reactor accident. The main alkyl iodides detected from the radiolysis of texanol ester and white spirits are methyl, ethyl, isopropyl and butyl iodide (see ).
3.1. Formation of organic iodides from texanol ester
Using the data presented by Blanksby and Ellison [Citation19], an estimate has been made of the energy required to form alkyl radicals from the texanol ester (see Figure ).
For both isomers of texanol ester (see Figure ), it is possible to break a carbon–carbon bond (a) to form an isopropyl radical and a heteroatom-stabilised radical, which forms two comparatively stable radicals. The formation of methyl radicals requires the breaking of one of the three bonds, (b), (c) or (d). The cleavage of bonds (b) and (c) forms the higher energy methyl radical and a secondary or tertiary alkyl radical, respectively. The cleavage of bond (d) would form a methyl radical and a radical stabilised by the ester group. The cleavage of bond (e) would form an isopropyl radical. As the radical formed by the loss of a hydrogen atom from formaldehyde is very stable, the cleavage of this bond might be a significant source of isopropyl radicals. If the data for C–H bonds in acetaldehyde and formaldehyde are used as surrogates to enable estimates of the energies of the oxygen-containing radicals formed by cleavage of bonds (d) and (e), then an estimate of the energy required to break bonds (a)–(e) can be made (see ).
Table 1 Energies required for homolysis of bonds (a)–(e)
(a) Formation of alkyl iodides from texanol ester under oxygen-free conditions (BWR case)
The ion chromatogram (see ) for 127 amu for the air in the headspace vial containing irradiated texanol ester exhibited a small peak due to methyl iodide (142 {M+} and 127 {I+} amu), which was observed at 32 seconds, and a large peak due to isopropyl iodide (170 {M+}, 155 {M+-CH3} and 127 {I+} amu). As no strong peaks at 156 amu (ethyl iodide) or 184 amu (butyl iodide) were observed, the alkyl iodides have been formed from methyl and isopropyl radicals, which were generated by the radiolysis of the texanol ester. While electron impact mass spectroscopy is unable to distinguish between the two different propyl iodides, as no credible fragmentation of the texanol ester would yield n-propyl radicals, it was assumed that isopropyl iodide was responsible for the ion at 170 amu.
Figure 4 The ion chromatograms for ions at 127 (I+), 142 (CH3I+) and 170 (CH3CHICH3 +) atomic mass units (amu) obtained from a gas chromatography measurement of the texanol ester irradiated with iodine
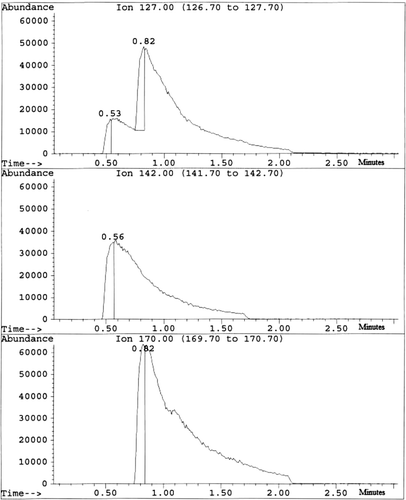
A series of small peaks was observed at 7.7, 8.1, 9.0, 10.8, 11.1 and 13.7 minutes. On examination of the mass spectra recorded at these times, the peak due to positively charged iodine atoms (127 amu) was not visible. This absence of iodine atoms strongly suggests that these compounds do not contain iodine. The chromatogram for the total ion current did not contain any major additional peaks. The compound at 8.1 minutes had a mass spectrum, which matched that of 2,2,4-trimethyl-1,3-pentanediol (145, 129, 103, 85, 73 and 56 amu) suggesting that, during the experiment, some cleavage of the ester groups had occurred. The peaks at 10.8 minutes (173, 143, 98, 83, 71 and 56 amu) and 11.1 minutes (173, 143, 89, 71 and 56 amu) were due to the two isomers of the texanol ester. It has so far been impossible to assign the mass spectra for the remaining peaks at 7.7, 9.0 and 13.7 minutes. The gas chromatogram obtained in a control experiment using unirradiated texanol ester only contained peaks at 10.8 and 11.1 minutes due to the texanol esters.
(b) Formation of alkyl iodides from texanol ester under aerobic conditions (PWR case)
Again, both methyl and isopropyl iodides were observed suggesting that the oxygen of the air is not able to inhibit the formation of both alkyl iodides. The concentration of isopropyl iodide was measured by GC-MS, but the reliable measurement of the concentration of methyl iodide was not possible. About 200 mmol/l of isopropyl iodide was formed from the iodine and the texanol ester after obtaining a dose of 220 kGy (see ).
Figure 5 Formation of isopropyl iodide from a texanol–elemental iodine solution under gamma irradiation (14 kGy/h)
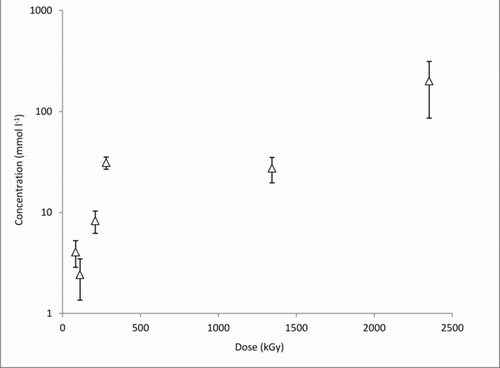
The spectrophotometric measurements suggest that after a dose of 100 kGy about 140 mmol/l of the elemental iodine had been consumed (see ). This suggests that about 70% of the iodine atoms have been incorporated into isopropyl iodide molecules. The production of alkyl iodides already at low doses (1.17 kGy) suggests that the production of both of the alkyl iodides from texanol ester under the conditions of a serious reactor accident is very likely. Our failure to observe ethyl iodide and butyl iodide by headspace gas chromatography strongly suggests that the fragmentation of this paint additive will occur in a well-defined way, which is dictated by the stability of the reactive species formed when the bonds are broken.
3.2. A comparison of Jotun and DecoMax white spirits
It has been shown that the presence of benzene can protect cyclohexane from gamma irradiation [Citation20]. It was postulated that the aromatics might alter the outcome of the experiment. Examination with gas chromatography direct liquid injection suggests that the profiles of the more volatile aliphatic hydrocarbons in the two grades of white spirits were very similar. However, the Jotun white spirit did contain some less volatile components, which were suspected to be aromatic hydrocarbons.
The examination of the white spirits with headspace GC-MS revealed that they were complex mixtures of mainly saturated hydrocarbons. The strongest peaks in the chromatogram were due to undecane (C11H24 7.19 minutes) and dodecane (C12H26 8.71 minutes). Many other alkanes and cycloalkanes were observed in the white spirit samples.
It was found that the DecoMax white spirit contained decahydronaphthalenes, while the Jotun white spirit contained small amounts of tetrahydronaphthalenes, alkyl benzenes and other unsaturated hydrocarbons.
3.3. Formation of alkyl iodides from white spirits (Stoddard solvent)
(a) Formation of alkyl iodides from white spirits under oxygen-free conditions (BWR case)
When both grades of white spirit were irradiated with elemental iodine present, methyl iodide (142 amu), ethyl iodide (156 amu), at least one isomer of propyl iodide (170 amu) and butyl iodide (184 amu) could be detected. These results suggest that a greater range of alkyl iodides will be formed from this paint solvent than from the texanol ester.
(b) Formation of alkyl iodides from white spirits under aerobic conditions (PWR case)
The irradiation of both grades of white spirit formed the same alkyl iodides as were observed when deoxygenated samples were irradiated. While it was possible to detect the presence of the alkyl iodides, their concentration was too low for a reliable quantification. Spectrophotometry does reveal that the vast majority of the iodine was consumed during the irradiation (see Figure ).
The radiation was more able to cause the DecoMax white spirit to react with the iodine than it was to make the Jotun white spirit react with the iodine. The reduced consumption of iodine by the Jotun white spirit could be explained by the fact that small amounts of aromatic compounds are able to protect alkanes from radiolysis [Citation21].
4. Conclusions
The irradiation of mixtures of paint solvents and elemental iodine forms alkyl iodides other than methyl iodide under both aerobic conditions (PWR case) and oxygen-free conditions (BWR case). When iodine solutions in all three paint solvents are subjected to 100 kGy the vast majority of the elemental iodine is converted into other chemical forms of iodine. White spirits formed mainly methyl iodide with smaller amounts of ethyl, propyl and butyl iodides. The reaction of the white spirits with iodine formed too low concentrations of these organic iodides for their concentrations to be measured reliably. The reaction of the texanol ester and iodine predominately formed isopropyl iodide and a small amount of methyl iodide. The large amount of isopropyl iodide formed from the texanol ester was quantified with GC-MS.
Because some higher alkyl iodides (ethyl and isopropyl iodide) react more slowly than methyl iodide with the DABCO added to the charcoal used in Maypack samplers, these compounds might pass through the sampler without being captured. This could lead to an underestimation of the amount of mobile radioactive iodine species.
The detailed studies of the paints present inside the reactor buildings of nuclear power plants would enable an estimation of the influence of different paint solvents on the volatile (organic) iodine source term during severe accidents such as Fukushima Daiichi in 2011.
Acknowledgements
Dr Aldo Jesorka (Chalmers University of Technology) is acknowledged for his kind help with the maintenance of the gas chromatography equipment.
References
- Hala , J and Navrail , J D . 2003 . Radioactivity, ionizing radiation and nuclear energy. 1st ed , 378 – 387 . Brno : Konvoj .
- Smith , A D , Jones , S R , Gray , J and Mitchell , K A . 2007 . A review of irradiated fuel particle releases from the Windscale piles, 1950–1957 . J Radiol Prot. , 27 : 115 – 145 . doi: 10.1088/0952-4746/27/2/001
- Hillary , J J and Evans , M G . 1986 . Compsn. for removing fission products from reactor coolant - comprises quat. salt of diazabi-cyclooctane on coconut based charcoal Patent UK GB2171399-A
- Clarke , C A , Roworth , C G and Holling , H E . 1945 . Methyl bromide poisoning. An account of four recent cases met with in one of H.M. ships . Br J Ind Med. , 2 : 17 – 23 . doi: 10.1136/bmj.2.4409.17
- Tanaka , S , Abuku , S-I , Imaizumi , K , Ishizuka , H , Seki , Y and Imamiya , S-I . 1989 . Efficiency of respirator canisters with methyl bromide . Ind Health. , 27 : 111 – 120 . doi: 10.2486/indhealth.27.111
- Morrison , B . 1987 . Organic chemistry , 5th ed. , 182 – 183 . Newton, MA : Allyn and Bacon .
- Morgan , D J and Black , A . 1968 . Mitchell G. Retention of inhaled ethyl iodide labelled with iodine-132 . Nature. , 218 : 1177 – 1178 . doi: 10.1038/2181177a0
- Noguchi , H and Murata , M . 1988 . Physicochemical speciation of airborne I-131 in Japan from Chernobyl . J Environ Radioact. , 7 : 65 – 74 . doi: 10.1016/0265-931X(88)90042-2
- Cline , J E , Roy , P A , Hollcroft , J W , Houbaugh , J Jr , McVey , T L , Thomas , C D Jr , Pelletiar , C A and Voilleque , P G . 1981 . Measurements of 129I and radioactive particulate concentrations in the TMI-2 containment atmosphere during and after the venting GEND009 , Washington, DC : Department of Energy .
- Glänneskog , H . 2004 . Interactions of I2 and CH3I with reactive metals under BWR severe-accident conditions . Nucl Eng Des. , 227 : 323 – 329 . doi: 10.1016/j.nucengdes.2003.11.008
- Morfin , F , Sabroux , J C and Renouprez , A . 2004 . Catalytic combustion of hydrogen for mitigating hydrogen risk in case of a severe accident in a nuclear power plant: study of catalysts poisoning in a representative atmosphere . Appl Catal B-Environ. , 47 : 47 – 58 . doi: 10.1016/j.apcatb.2003.07.001
- Nacapricha , D and Taylor , C G . 1996 . Quality control of nuclear charcoals: particle size effect and trapping mechanism . Carbon. , 34 : 155 – 163 . doi: 10.1016/0008-6223(96)00163-7
- Wren , J C , Moore , C J , Rasmussen , M T and Weaver , K R . 1999 . Methyl iodide trapping efficiency of aged charcoal samples from Bruce-A emergency filtered air discharge systems . Nucl Technol. , 125 : 28 – 39 .
- Tietze , S , Foreman , M RStJ , Ekberg , C and van Dongen , B . 2013 . Identification of the chemical inventory of different paint types applied in nuclear facilities . J Radioanal Nucl Ch. , 3 : 1981 – 1999 . doi: 10.1007/s10967-012-2190-3
- Kemp , W . 1987 . Organic spectroscopy , 2nd ed. , Vol. 4 , 188 – 222 . Basingstoke : Macmillan . Ultraviolet and visible spectroscopy
- Komiya , S . 1997 . Synthesis of organometallic compounds , 35 – 55 . Chichester : John Wiley & Sons . Chapter 4, Manipulation of air-sensitive compounds
- Taghipour , F and Evans , G J . 2000 . Radiolytic organic iodide formation under nuclear reactor accident conditions . Environ Sci Technol. , 12 : 3012 – 3017 . doi: 10.1021/es990507d
- Wieslander , G and Norbäck , D . 2010 . Ocular symptoms, tear film stability, nasal patency, and biomarkers in nasal lavage in indoor painters in relation to emissions from water-based paint . Int Arch Occ Environ Hea. , 83 : 733 – 741 . doi: 10.1007/s00420-010-0552-0
- Blanksby , S J and Ellison , G B . 2003 . Bond dissociation energies of organic molecules . AccChem Res. , 36 : 255 – 263 . doi: 10.1021/ar020230d
- Lamborn , J and Swallow , A J . 1961 . The protection effect in gamma-radiolysis of benzene-cyclohexane mixtures and its explanation in terms of selectivity of primary radiation act . J Phys Chem. , 65 : 920 doi: 10.1021/j100824a006
- Manion , J P and Burton , M . 1952 . Radiolysis of hydrocarbon mixtures . J Phys Chem. , 65 : 560 – 569 . doi: 10.1021/j150497a005