Abstract
We proposed a method to measure the radioactivity of Sr/Y-90 in the mixture of Cs-134 and Cs-137 without chemical pretreatment. It was realized by subtracting the electrons produced in Cs-134 and Cs-137 from entire electron emission rate that can be determined by the efficiency tracer technique in the 4πβ–γ coincidence counting method. The radioactivity of Cs-134 and Cs-137 can be determined by gamma ray spectrometry. The measurements were conducted using plastic and NaI(Tl) scintillation detector with a large solid angle. The validity of the measurement method was shown by agreement of the results with the known radioactivities.
1. Introduction
Radioactivity measurements of samples that are thought to be contaminated by some type of radionuclide are conducted to protect the public from exposure. In the accident at Fukushima Daiichi Nuclear Power Plant, a great amount of radioactive material was released [Citation1]. In this case, the radioactivities of Cs-134 (half-life T1/2 = 2.0664 y [Citation2]), Cs-137 (T1/2 = 30.05 y [Citation3]), and Sr/Y-90 (T1/2 = 28.80 y [Citation3]) are of great concern for long-term uptake.
Radioactivity is typically determined using counts from a detector that has a calibrated efficiency. Gamma ray spectrometry is often employed because samples are prepared by a simple treatment such as mixing and filling in a container. Gamma rays from the sample in the container are observed by a detector such as a high-purity germanium detector, which is often used because of its outstanding energy resolution. In gamma ray spectrometry, the radionuclide in the mixed sample is identified by observing the full-energy peak of the gamma rays. The radioactivity of the radionuclide is calculated using the counting efficiencies calibrated for each radionuclide. A pure beta emitter such as Sr/Y-90 cannot be measured by gamma ray spectrometers because no gamma ray is emitted from the pure beta emitter.
To measure the radioactivity of a pure beta emitter, a windowless gaseous detector and liquid scintillation counter are employed. If radionuclides are included in a sample, however, it is difficult to identify them because the measured beta ray energy spectrum shows a continuous distribution.
To determine the radioactivity of a pure beta emitter in a mixed sample including gamma and pure beta emitters, for example, a mixture of Cs-134, Cs-137, and Sr/Y-90, chemical pretreatment is currently required to separate the pure beta emitter from the other nuclides. The pretreatment is conducted in a chemical experimental room by skilled personnel using a complex procedure. Although a disk for selective capture of Sr-90 [Citation4] is used, chemical pretreatment using a strong acid solution is required before counting beta rays.
We have already proposed a method to measure the radioactivity of Cs-134, Cs-137, and Sr/Y-90 without chemical pretreatment [Citation5]. This method employs both gamma and beta detectors with a large solid angle. The combination of gamma and beta ray detectors provides (1) discrimination of Cs-134 from Cs-137 and (2) absolute determination of the entire beta ray emission rate. The signals from Cs-134 and Cs-137 in the gamma spectrum are distinguished by the coincidence of beta and gamma rays. Cs-134 emits beta and gamma rays simultaneously. In contrast, Cs-137 emits a gamma ray at an interval after beta ray emission because the gamma ray is emitted by a transition from the metastable state to the ground state of Ba-137, with a half-life of 2.5 min, after the beta transition from Cs-137.
The radioactivity of Sr/Y-90 is determined by counting the rate of beta rays, which is obtained by subtracting the electrons produced in Cs-134 and Cs-137 from the entire electron emission rate. The electron count from Cs-134 and Cs-137 can be determined using gamma ray spectrometry. For absolute measurement of the entire electron emission rate, the efficiency tracer technique in 4πβ−γ coincidence counting method [Citation6] is applied. The absolute measurement does not require calibration using a standard radioactive source.
There are some candidates for beta and gamma detectors for 4πβ−γ coincidence counting. They were designed to achieve high detection efficiencies of both beta and gamma rays. For example, a gaseous proportional counter with an internal radioactive source on a thin film was applied as a beta detector [Citation7]. In another case, advantages and problems of a liquid scintillation counter as a beta detector were discussed [Citation8].
We intend to develop instruments that can be used to measure the radioactivity of a mixed sample by methods that do not require professional skill. For beta ray detection, the self-absorption in the sample should be as small as possible. Therefore, we chose a plastic scintillator for beta ray detection because it can be used as a disposable container by direct filling of the sample. The use of a plastic scintillator for 4πβ−γ coincidence counting was proposed by Kawada [Citation9] to improve the detection efficiency of gamma rays using a well-type NaI(Tl) scintillation detector. We develop an alternative experimental setup that is an assembly of beta and gamma ray detectors.
The experimental result in this paper demonstrates measurement of Sr/Y-90 radioactivity in a mixture of Cs-134 and Cs-137 in comparison with the known activities. The measurement uncertainty is estimated to determine the detectable level of the radioactivity ratio of Sr/Y-90 to Cs-134 and Cs-137 in the gamma ray spectrometry results. It is demonstrated that the Sr/Y-90 radioactivity in a mixture of Cs-134 and Cs-137 can be measured by employing the detectors and analyzing the obtained data without chemical separation.
2. Experimental setup
shows an outline view of our detector assembly. It is equipped with a light guide for photomultipliers (PMTs) to deliver scintillation light, which is caused mainly by beta rays in a plastic scintillator. The light guide allows us to make the solid angle of both beta and gamma ray detectors as large as possible. A sample was sealed in the plastic scintillator as shown in to achieve a 4π solid angle for detection of beta rays from the sample. A pair of PMTs was placed on opposite sides of the sample to reduce the noise of the PMT itself by coincidence counting. The gamma ray detectors were placed as close as possible to increase the solid angle. Shields are required around the gamma ray detectors for stable measurement. These elements of the assembly are described in detail in the following sections.
Figure 1. Outline view of detector assembly. Prepared sample sandwiched by a pair of plastic scintillators, which were inserted. Cross-sectional view of assembled detectors was drawn to scale.
Note: PLS, plastic scintillator.
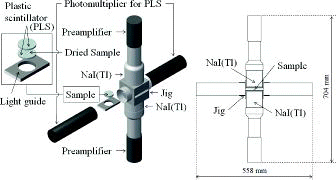
2.1. Sample preparation
A radioactive sample in a sealed plastic scintillator was prepared in an experimental room. A radioactive water solution was dropped and mixed on the center of the circular plate in a plastic scintillator (Eljen Technology, EJ-212, 40 mm in diameter × 4 mm in thickness). Three samples with different activities were prepared with (1) only Cs-134, (2) a mixture of Cs-134 and Cs-137, and (3) a mixture of Cs-134, Cs-137, and Sr/Y-90. The radioactivity of the drop for sample (1) was 77.1 Bq of Cs-134 in 36.4 mg of carrier solution. The activities of the drops for mixture sample (2) were 23.7 Bq of Cs-134 in 11.2 mg and 31.0 Bq of Cs-137 in 12.2 mg. Those of the drops for mixture sample (3) were 16.5 Bq of Cs-134 in 7.82 mg, 27.0 Bq of Cs-137 in 10.6 mg, and 52.0 Bq of Sr/Y-90 in 11.8 mg. The radioactivity ratio of Cs-134 and Cs-137 was similar to that in the contamination caused by the release from the accident at Fukushima Daiichi Nuclear Power Plant in June 2012. As a seeding agent, the same amount of diluted (1:8000) Ludox SM-30 was added to the drops [Citation10]. The drops were dried completely in a desiccator for half a day.
The weights were measured by a comparator (Mettler, AT21) using the difference in the weight of water solution in a picnometer before and after the drop was added. Their radioactivities were measured by a calibrated pressurized ionization chamber (Centronic, IG11N20) for Cs-134 and Cs-137 and a liquid scintillation counter (PerkinElmer, Tri-Carb liquid scintillation counter 3110TR) for Sr-90. These uncertainties for calibrated radioactivity of Cs-134, Cs-137 and Sr-90 were 0.9%, 1.2% and 1.4%, respectively, with coverage factor k = 2.
The dried sample was completely covered by another circular plate in a plastic scintillator. Optical grease was placed on the outer edge of the plate before coupling. The set of plates was inserted into a through-hole (40 + 0.2 mm in diameter) in the light guide (50 × 88 × 8 mm) that connected the plastic scintillators and the PMT optically using grease. The set consisting of the plastic scintillators and the light guide formed a cuboid after the insertion. A white reflector (EJ-510) was painted on the surface of the cuboid except for the faces in contact with the PMT.
2.2. Detector assembly
As shown in , this detector consisted of plastic and NaI(Tl) scintillation detectors that were placed orthogonally to each other. The entire instrument was fixed by an aluminum jig. The plastic scintillator, which acts as a sample capsule, was inserted into a through-hole in the aluminum jig with the light guide. Both side edges of the light guide were connected to the PMT (Hamamatsu, H3177-50) by optical grease. The pair of PMTs could pick up one scintillation event for each coincidence trigger. Outside light was blocked by black tape (3M, Scotch® Super 33+TM) around the insertion port for the PMT. The NaI(Tl) scintillation detectors (Saint-Gobain, 3M3/3) have a cylindrical crystal (3 in. in diameter and 3 in. in thickness). These detectors were fixed to stand perpendicular to the PMT for the plastic scintillator. Wells were dug into the aluminum jig as deep as possible to bring the NaI(Tl) scintillation detectors closer to the sample. The NaI(Tl) scintillation detector was coupled with a 14-pin socket-type preamplifier (Clear Pulse, 5626). The overall height of the assembly was 704 mm and the width was 558 mm, excluding the connector and cable for signal output. The shortest distance from the sample to the front face of the NaI(Tl) scintillation detector was 7 mm. The detector wall and reflector were between the front faces of the crystals in the detector.
The detector assembly was placed on the floor of the experimental room using supporting instruments. Lead shields 50 mm in thickness were placed at the lateral sides of the NaI(Tl) scintillation detectors.
2.3. Data acquisition
shows the electrical circuit for data acquisition, which consists of standard nuclear instrumentation module (NIM) and computer automated measurement and control (CAMAC) modules. The circuit was configured to acquire the pulse heights of the signal from the detectors in list mode. It was divided into two sections, one for pulse shaping and the other for trigger control. The pulse heights were analyzed by a peak-hold-type analog-to-digital converter (ADC) via a preamplifier and shaping amplifier. Detection signals of scintillation events were used to generate the trigger signal for data acquisition. The signals from the pair of PMTs for beta ray counting were fed to the coincidence circuit (“Coin103” in ) before the fan-in/fan-out circuit (“FI/FO102”). Gate signals to the ADC were generated at the gate generator (“G.G.1500 (a)”). To reject the trigger signal during ADC conversion and data transfer, veto signals were fed to “Disk 305.” When “CC/NET” received a trigger signal from “G.G.1500 (b),” ADC data were transferred to a personal computer (PC). The combination of signals from “G.G.1500(c)” and the busy out from “CC/NET” generated the veto signal. The real and live times of data acquisition time were recorded by “Scaler 425.”
Figure 2. Block diagram of electrical circuit for data acquisition.
Note: PA113, Preamp (ORTEC, Model No. 113); Amp572A, spectroscopy amplifier (ORTEC); TFA474, timing filter amplifier (ORTEC); CFD, constant fraction discriminator (ORTEC); TAC2043, time-to-amplitude converter (Canberra); Coin103, coincidence circuit (Technoland, N-TM103); FI/FO102, logic fan-in/fan-out (Technoland, N-TM102); Disc203, discriminator (Technoland, N-TM305); CG203, clock generator (Technoland, N-TM203); G.G.1500, gate generator (Kaizu Works, KN1500); ADC405, analog-to-digital converter (Technoland, C-TM405); Scaler 425, visual scaler (Technoland, N-OR 425); CC/NET, network crate controller (TOYO Corporation).
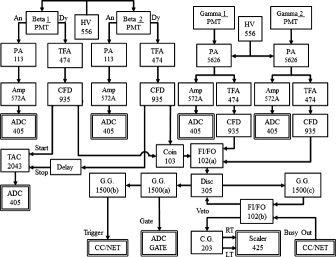
2.4. Data analysis
shows block diagram of data analysis for measurement of the prepared samples. To measure the radioactivity of Sr/Y-90 (ASr/Y-90) in mixed samples containing Cs-134 and Cs-137, the electron emission rates of Cs-134 (ACs-134) and Cs-137 (ACs-137) should be subtracted from the entire electron emission rate from a sample (AEntire) as follows:
(1)
2.4.1. Extrapolation technique in 4πβ–γ coincidence counting method
It is known that radioactivity is measured directly by 4πβ−γ coincidence counting without determining the detection efficiencies [Citation6]. The method is expressed in the following formula:
(2) where ρβ, ργ, and ρβγ are the experimentally observed count rates of beta rays and gamma rays and the coincidence between beta and gamma rays, respectively. Further, ϵβ and ϵγ are the detection efficiencies of beta and gamma rays, respectively. Formula (2) shows that the radioactivity A is independent of the efficiencies. It is modified to formula (3) because a beta ray detector is sensitive to only a fraction of the gamma rays and conversion electrons,
(3) where α is the total internal conversion coefficient, ϵce is the detection efficiency of beta ray detector for internal conversion electrons, and ϵβγ is the gamma ray detection efficiency of the beta ray detector. To obtain the radioactivityA, (1 − ϵβ)/ϵβ is extrapolated toward zero (i.e., ϵβ → 1) by varying the beta ray detection efficiency. Here, the detection efficiency was controlled by varying the threshold level of the pulse height for beta counting in offline analysis of the list data.
2.4.2. Efficiency tracer technique
When a prepared sample contains both a pure beta emitter and a beta–gamma ray emitter, the beta ray emission rate is measured by the 4πβ−γ coincidence counting method with the extrapolation technique. The radioactivity of the pure beta emitter is obtained by subtracting the emission rate of the beta and gamma ray emitters, which acts as a tracer. The efficiency tracer technique stands on an idea in which it is considered that the efficiency of the pure beta emitter is also extrapolated to one when the beta ray detection efficiency of the tracer radionuclide is extrapolated toward one. Efficiency tracing can be also applied to a radionuclide that has a long half-life excited state such as Cs-137. In fact, the efficiency tracer technique has been adopted for radioactivity standardization of Cs-137 by national metrology institutes in the world [Citation11].
3. Results
Gamma ray pulse height distributions obtained by measurement for the mixture sample of Cs-134 and Cs-137 with the NaI(Tl) scintillation detectors are shown in . Overlapping peaks at 605 keV from Cs-134 and 662 keV from Cs-137 were observed in the raw pulse height distribution. Peaks of gamma rays which have small emission rate such as 596 and 802 keV from Cs-134 were included in the primary peaks. Under the condition of coincidence with beta ray detection, the 662 keV peak disappeared from the gamma ray spectrum because the 662 keV gamma rays were emitted non-simultaneously with beta disintegration. This is because the 662 keV gamma rays are emitted in decays from the metastable excited level of Ba-137 to the ground level, which has a relatively long half-life (2.5 min). In , the sum peak was observed under the condition of coincidence with beta ray detection. The center of sum peak appeared at slightly lower channels than the peak in the raw pulse height distribution because counts of 1461 keV from K-40 which exist in the background were eliminated by the condition of coincidence with beta ray detection.
Figure 4. Obtained pulse height distributions of NaI(Tl) scintillation detector for mixture sample of Cs-134 and Cs-137. Hatched lines indicate pulse height distribution under condition of coincidence with beta ray detection.
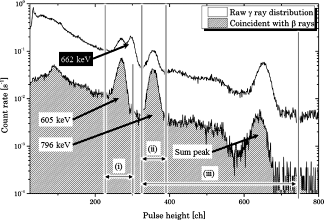
shows beta ray pulse height distribution obtained by measurement for the mixture sample of Cs-134, Cs-137, and Sr/Y-90 with the plastic scintillation detector. The peak for the internal conversion electrons from Cs-137 appeared clearly in continuous distribution. The distributions obtained by measurement for single-nuclide samples of Cs-134 and Sr/Y-90 and normalized to the estimated radioactivity are displayed. The distribution under the condition of anticoincidence with gamma rays was drawn to remove beta ray events from Cs-134. The flat region to the left of the peak was derived from the events from Y-90.
Figure 5. Obtained pulse height distributions of plastic scintillation detector for mixture sample of Cs-134, Cs-137, and Sr/Y-90 along with normalized distributions for single samples of Sr/Y-90 and Cs-134. Light gray area indicates pulse height distribution under condition of anticoincidence with gamma ray detection.
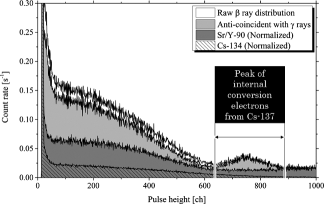
3.1. Single sample of Cs-134
The Cs-134 sample was measured for 3883 s in live time. shows the obtained plots of the apparent radioactivity as a function of beta ray detection efficiency. Second-order polynomial extrapolation curves were drawn to determine the radioactivity of Cs-134 by 4πβ−γ coincidence counting. The use of polynomial curves followed empirical procedures and theoretical consideration [Citation12,13]. The configured windows for gamma ray pulse height denoted “605 keV,” “796 keV,” and “796 keV and sum peak” are denoted in as (i), (ii), and (iii), respectively. The radioactivity of the sample was estimated to be 76.7 Bq by averaging the three extrapolations. The value agreed well with the known radioactivity (−0.5%) within the uncertainty of extrapolation (1.0%, k = 1). The radioactivity yielded a 796 keV counting efficiency of 11.6% for the NaI(Tl) scintillation detector.
Figure 6. Obtained extrapolation curve for Cs-134 with three gamma ray gate configurations. Closed triangles indicate data obtained with window for 605-keV full-energy peak, crosses indicate that obtained with window for 796-keV full-energy peaks, and open circles indicate that obtained with window for 796-keV peaks and their sum.
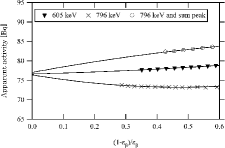
3.2. Mixture sample of Cs-134 and Cs-137
The mixture sample of Cs-134 and Cs-137 was measured for 3453 s in live time. shows the obtained plots of the apparent electron emission rate from the sample. Second-order polynomial extrapolation curves were drawn to determine the beta ray emission rate of this mixture sample by efficiency tracing. The entire electron emission rate was directly estimated to be 57.8 s−1 by averaging the two extrapolations. The internal conversion electrons of Cs-137 were included in the emission rate. The value of entire electron emission rate agreed well with the known radioactivity (+0.3%). The difference was within the uncertainties of the extrapolation (2.1%, k = 1).
The gross count rate of the 796 keV peak in the raw distribution was 2.71 s−1. The Cs-134 radioactivity in the mixture sample was estimated to be 23.3 Bq from the count rate. The value was 1.5% smaller than the known radioactivity. The electron emission rate from Cs-137, including the internal conversion electrons produced by decay from the metastable excited level of Ba-137, in the mixture sample was estimated to be 34.5 s−1 (31.5 Bq) by subtracting the contribution of Cs-134 from the entire electron emission rate. The value was 1.9% larger than the known emission rate. The Cs-137 radioactivity yields a 662 keV counting efficiency of 31.2% for the NaI(Tl) scintillation detector.
3.3. Mixture sample of Cs-134, Cs-137, and Sr/Y-90
The mixture sample of Cs-134, Cs-137, and Sr/Y-90 was measured for 4379 s in live time. shows the obtained plots of the apparent electron emission rate from the sample. Second-order polynomial extrapolation curves were drawn to determine the beta ray emission rates of Cs-134, Cs-137, and Sr/Y-90 for efficiency tracing. The beta ray emission rate was estimated to be 98.5 s−1 by averaging the two extrapolations. The value was 0.5% larger than the known emission rate. This shows good agreement within the uncertainties of the extrapolation (1.3%, k = 1).
From gamma ray spectrometry using the efficiencies calibrated by the previous measurement of the single sample of Cs-134 and the mixture sample of Cs-134 and Cs-137, the activities of Cs-134 and Cs-137 in the mixture sample were estimated to be 16.2 and 26.2 Bq, respectively. The values were 1.8% and 2.9% smaller than the known activities. The Sr/Y-90 radioactivity in the mixture sample was estimated to be 53.2 Bq by subtracting the contributions of Cs-134 and Cs-137 from the entire electron emission rate. The value was 3.1% larger than the known radioactivity. The difference was within the uncertainty which is described below.
3.4. Uncertainties
shows an estimated uncertainties budget sheet for the determination of Sr/Y-90 radioactivity in the mixture with Cs-134 and Cs-137. Counting statistical uncertainty included counting of the beta ray detector and the gamma ray detector at measurement for each of the samples. Dominator in the total uncertainty was due to the extrapolation technique for the 4πβ−γ coincidence counting method. This was mainly because beta ray detection efficiency of the present setup was not sufficient for detection of weak scintillation derived from low-energy beta rays. Uncertainty of the window in the gamma ray pulse height distribution was estimated by considering variation in pulse height channel. Uncertainty of the extrapolation was derived from deviation of the fitting curve and difference between the several fitting curves. Uncertainty of the background component was divided into influence for the extrapolation and the gamma ray spectrometry. Perturbation of the background was based on actual measurements before and after measurements for the samples. Uncertainty of repeatability in sample setting was estimated by actual repeated measurement for same sample. It was mainly considered for difference of optical contact with grease between the plastic scintillator and the PMTs. Uncertainty of the source position in gamma ray spectrometry was estimated using a Monte Carlo simulation. Uncertainty of dead time and resolving time was considered for variation in the data acquisition system. Uncertainty of timer was derived from calibration. The estimated total uncertainty for Sr/Y-90 radioactivity of Sr/Y-90 in the mixture was estimated as 7.9%.
Table 1. Uncertainty budget for estimation of Sr/Y-90 activity in a sample mixed with Cs-134 and Cs-137.
4. Discussion
It is validated that this measurement method using an assembly of plastic and NaI(Tl) scintillation detectors can be used to detect Sr/Y-90 in a mixture of Cs-134 and Cs-137 in comparison with the known radioactivity. A principal specification for radioactivity measurement of Sr/Y-90 in mixture with Cs-134 and Cs-137 is the uncertainty. In the case of the present sample, it is expected that the radioactivity of Sr/Y-90 in a mixture with Cs-134 and Cs-137 can be detected at a ratio greater than uncertainty. The estimated total uncertainties indicate that the measurement is applicable to detection of Sr/Y-90 at levels as low as one-tenth the total radioactivity of Cs-134 and Cs-137, as described in “indices for limiting food and drinks ingestion” [Citation14]. The uncertainty is important for monitoring of contamination because the detectable level might be reflected in the criteria for judgments.
5. Conclusion
We invented orthogonal detectors with plastic and NaI(Tl) scintillation detectors that realized measurement with a large solid angle of both beta and gamma rays. A facing pair of PMTs read out events that coincided with each other for beta ray counting. It was necessary to acquire weak scintillation signals by low-energy beta rays. The gamma ray detectors had high counting efficiencies with as large a solid angle as possible.
The conducted measurement successfully estimated Sr/Y-90 radioactivity in a mixture of Cs-134 and Cs-137 with its uncertainty. During the analysis by the extrapolation technique in 4πβ−γ coincidence counting and gamma ray spectrometry, each result was compared with the known activities to check the validity of this method. The uncertainty was less than 10%, and can be reduced by improving the beta ray counting efficiency, especially at low-energy region.
It is necessary to discuss the objective and applications of this measurement method. The effect of self-absorption in the sample is a significant point for application. Furthermore, the detectable amount and concentration of radioactivity in sample depend on the sensitivities of the detectors. It is predicted that actual samples contain K-40, a naturally occurring radioactive material. It is expected that the K-40 activity is dealt with appropriately by the separation from sum peak of Cs-134 using beta ray coincidence because 1461 keV from K-40 is emitted in electron capture.
The detector assembly and analytical methods enable radioactivity measurement of Sr/Y-90 in a mixture of Cs-134 and Cs-137 without chemical separation. Professional skill is not needed to set up the sample. This procedure is expected to reduce the amount of time required and the problems of residual chemical products. The cost of preparing the instrument is not high, unlike that of a high-purity germanium detector, and the plastic scintillator in particular is disposable.
Acknowledgements
The authors wish to express great thanks to Dr Kawada who gave us valuable comment and support. We also appreciate National Institute of Advanced Industrial Science and Technology (AIST) and High Energy Accelerator Research Organization (KEK) members who supported this work.
Additional information
Funding
References
- Kobayashi T, Nagai H, Chino M, Kawamura H. Source term estimation of atmospheric release due to the Fukushima Dai-ichi Nuclear Power Plant accident by atmospheric and oceanic dispersion simulations. J Nucl Sci Technol. 2013;50:255–264.
- Bé MM, Chisté V, Dulieu C, Browne E, Baglin C, Chechev V, Kuzmenco N, Helmer R, Kondev F, MacMahon D, Lee KB. Table of radionuclides. Paris (France): Bureau International des Poids et Mesures; Vol. 7, Monographie BIPM-5; 2013. Available from: http://www.bipm.org/utils/common/pdf/monographieRI/Monographie_BIPM-5_Tables_Vol7.pdf
- Bé MM, Chisté V, Dulieu C, Mougeot X, Chechev V, Kondev F, Nichols AL, Huang X, Wang B. Table of radionuclides. Paris (France): Bureau International des Poids et Mesures; Vol. 3, Monographie BIPM-5; 2006. Available from: http://www.bipm.org/utils/common/pdf/monographieRI/Monographie_BIPM-5_Tables_Vol3.pdf
- Smith LL, Orlandini KA, Alvarado JS, Hoffmann KM, Seely DC, Shannon RT. Application of EmporeTM Strontium Rad Disks to the analysis of radiostrontium in environmental water samples. Radiochim Acta. 1996;73:165–170.
- Unno Y, Sanami T, Hagiwara M, Sasaki S, Yunoki A. Application of beta coincidence to nuclide identification of radioactive samples contaminated by the accident at the Fukushima Nuclear Power Plant. Prog Nucl Sci Technol. Forthcoming.
- International Commission on Radiation Units and Measurements. Particle counting in radioactivity measurements (ICRU report; no. 52). Oxford (UK): Oxford University Press; 1994.
- Kawada Y. A new method of measuring the response of a 4πβ-counter to γ-rays and internal conversion electrons. Int J Appl Rad Isot. 1969;20:413–422.
- Yura O. 4πβ(LS)-γ coincidence counting in the electrotechnical laboratory. Bull Electrotech Lab 1983;47:815–821.
- Kawada Y, Ohtuka M, Wang QW, Hino Y. Absolute radioactivity measurements by the use of a 4πβ–4πγ detector configuration. Appl Rad Isot. 2004;60:357–362.
- National Council on Radiation Protection and Measurements. A handbook of radioactivity measurements procedures 2nd edition (NCRP report; no. 58). Bethesda: National Council on Radiation Protection and Measurements; 1985.
- Ratel G, Michotte C, Hino Y, Wätjen U. Activity measurements of the radionuclide 137Cs for the NMIJ, Japan and the IRMM, Geel in the ongoing comparison BIPM.RI(II)-K1.Cs-137 and update of the KCRV. Metrologia. 2005;42. Technical Supplement; 06009.
- Baerg AP. The efficiency extrapolation method in coincidence counting. Nucl Instrum Methods. 1973;112:143–150.
- Miyahara H, Momose T, Watanabe T. Optimisation of efficiency extrapolation functions in radioactivity standardisation. Int J Appl Rad Isot. 1986;37:1–5.
- Nuclear Safety Commission of Japan (JP). Regulatory guide: emergency preparedness for nuclear facilities. Tokyo: Nuclear Safety Commission of Japan, 2010. Available from: http://www.mhlw.go.jp/stf/shingi/2r98520000018iyb-att/2r98520000018k4m.pdf