Abstract
The leaching properties of soluble components (Na+, NO−3, and NO−2) in synthetic bituminized wastes with various characteristics, such as salt content, salt composition, and bitumen matrix, were quantitatively evaluated by means of an aqueous leaching test. Micropores were formed in the bituminized waste by the leaching of soluble salts. The penetrability of bitumen and the salt content affected the size and the shape of the micropores observed by scanning electron microscopy. The linear relationship between leaching fraction of the soluble components and square root of leaching time was observed, and the diffusion coefficient of the soluble component was determined by Fick's law. The diffusion coefficients of ionic species of interest were similar to each other. Moreover, the diffusion coefficient agreed among bituminized wastes with the same salt content, even if salt composition and bitumen matrix were different. The diffusion coefficient of the component was proportional to the salt content in the synthetic bituminized wastes. It was found that the salt content in the bituminized waste was one of the key factors to determine the diffusion coefficient.
1. Introduction
Low-level radioactive concentrate, which was generated by the reprocessing of spent nuclear fuels, was solidified as bituminized waste (BW) in the Tokai reprocessing plant from 1982 to 1997. During this period, approximately 6000 m3 of the Tokai BW was produced and stored in asphalt solidification storages. The Tokai BW was categorized into two groups according to the origin of the low-level radioactive concentrate. One is the low activity group: ca. 2600 m3 of waste with 108 Bq/t of total specific α-ray radioactivity and 1010 Bq/t of total specific β-ray and γ-ray radioactivities, and the other is the middle activity (MA) group: ca. 3600 m3 of waste with 109 Bq/t of total specific α-ray radioactivity and 1011 Bq/t of total specific β-ray and γ-ray radioactivities. The MA group of the BW will be disposed in a deep geological disposal site in Japan [Citation1].
The Tokai BW mainly consists of ca. 55 wt.% Blown Bitumen AD Compound (Showa Shell Sekiyu KK), which is the name of a commodity that has the same physical properties as the Blown Bitumen R85/40, and ca. 45 wt.% salts, which in turn consist of ca. 60 wt.% sodium nitrate, ca. 24 wt.% sodium nitrite, ca. 7 wt.% other soluble salts, and ca. 9 wt.% insoluble salts [Citation2]. There are, however, a certain amount of BWs with different compositions depending on campaigns of the liquid waste treatment. Bitumen has very low permeability to water, and its ability to restrict the transfer of water makes bitumen work as a semipermeable membrane [Citation3,4]. Hence, when the BW encounters water, the water penetrates the BW, the embedded soluble salts dissolve in the penetrating water, and the BW swells by the osmotic effect of the water. Then, the dissolved soluble salts leach out and micropores are formed in the BW after the leaching of soluble salts [Citation5–9]. The leached soluble components, such as NO3−, have the potential to decrease the sorption coefficients of the dissolved radionuclide ions on the engineered and natural barriers of the disposal site and to promote localized corrosion on the metallic containers used for the disposal of high-level radioactive wastes [Citation10]. Therefore, it is important to understand the leaching behavior of the soluble component in the BW in contact with water.
There are practically bituminized radioactive wastes with various salt contents (i.e. the salt/bitumen weight ratio: S/B ratio) and salt compositions in the Tokai BWs. Also, other BWs with different salt contents, salt compositions, and the bitumen matrices from the Tokai BWs have been produced in overseas countries. When the leaching behavior of the soluble components in the Tokai BWs is compared with that for the other BWs, quantitative evaluation of the effects of salt content, salt composition, and bitumen matrix on the leaching behavior of the BW is required. However, the effects of them have not been quantitatively and experimentally determined. In this study, we systematically conducted aqueous leaching tests for several synthetic BWs with various salt contents, salt compositions, and the bitumen matrices. We quantitatively evaluated the diffusion coefficients of the soluble components (Na+, NO−3, and NO−2) in the BWs by using Fick's law from the leaching fractions of the components. The diffusion coefficient by Fick's law was used in order to compare with that for the other BWs (synthetic COGEMA, which was the French reprocessing company, type and Eurobitum BWs) [Citation7,8].
The Tokai BWs were produced by using the AD Compound, but the AD Compound is currently unavailable; therefore, the synthetic BWs with various salt contents were produced by the Blown Bitumen 20/30 (Showa Shell Sekiyu KK), which shows its penetration depth of 2.0–3.0 mm at 25 °C, as bitumen with basic characteristics similar to the AD Compound (). A major difference in physical properties of the bitumen matrices between the AD Compound and the Blown Bitumen 20/30 is the penetrability of the bitumen. Blown Bitumen 20/30 has lower penetrability than the AD Compound, indicating that the Blown Bitumen 20/30 is harder than the AD Compound. In contrast, the density, the softening points, and the ductility of the AD Compound and Blown Bitumen 20/30 are almost the same.
Table 1. Characteristics of the Blown Bitumen AD Compound and 20/30.
2. Experimental
2.1. Synthetic bituminized wastes
A liquid solution simulating the low-level radioactive concentrate in the Tokai reprocessing process was prepared; the salt concentrations in the liquid solution were 192 g/dm3 NaNO3, 20 g/dm3 NaNO2, 72 g/dm3 Na2CO3, 1.7 g/dm3 Na3PO4, and 17 g/dm3 NaOH. Eleven grams of Ba(OH)2 was added to every liter of the liquid solution, and the pH of the liquid solution was adjusted to 9 by adding conc. HNO3. Then, 6.8 g K4Fe(CN)6, 5.8 g NiSO4, 3.8 g Na2SO3, and 0.05 g AgNO3 were added to every liter of the liquid solution. The Blown Bitumen 20/30 was melted by heating in a beaker at 130–180 °C. The liquid solution was added at 2–10 cm3/min to melted bitumen while stirring at 300 rpm. A synthetic BW with an S/B ratio of 45/55 (BW45, 6.5 kg) was produced from the simulated solution. The prepared BW45 was divided into six steel vessels (approximately 1 kg BW in each vessel). The BW45 was cut into three parts; the upper, the center, and the lower parts of the vessel. The bitumen matrix of each part was dissolved in xylene, the salts were separated by filtration under reduced pressure, and the separated salts were weighed. The salt weight percent of the upper, center, and lower parts showed the same values (45 ± 1%) within the margin of error, indicating that the salt was homogenously distributed in the synthetic BWs.
BWs synthesized with NaNO3 powder were prepared to adjust the S/B ratio and the size of salts. In this preparation, NaNO3 was crushed using an agate mortar, and the NaNO3 powder (<40 μm) was recovered in a 390-mesh size sieve. Instead of the liquid solution, the NaNO3 powder was added to the melted bitumen. Five synthetic BWs with S/B ratios of 10/90, 25/75, 40/60, 55/45, and 70/30 (BW10, BW25, BW40, BW55, and BW70, respectively) were prepared with the NaNO3 powder.
The synthetic BWs with the AD Compound produced in 1999 (BWAD) was provided from the Tokai reprocessing technology development center. The BWAD was prepared as follows [Citation11]. A twin-screw extruder was used at a retaining speed of 275 rpm. Then, 5.3 dm3/h of the simulated solution was fed to 1.9 dm3/h of AD Compound at 180 °C for 48 h. The BWAD obtained after the apparatus became stable was employed. The basic characteristics of the synthetic BW samples are summarized in .
Table 2. Basic characteristics of the synthetic BWs.
The total amount of the soluble components (C0) was determined in a sample of the synthetic BWs. A part of the synthetic BW was weighed and then dissolved in toluene. Then, the soluble salts were dissolved by adding de-ionized water and shaking. The soluble components in the recovered de-ionized water were measured by inductively coupled plasma-atomic emission spectrometry (ICP-AES) and ion chromatography.
2.2. Aqueous leaching test
Specimens of the synthetic BWs were prepared for aqueous leaching tests. The specimens were molded using a stainless steel container of dimensions Ø 38 mm×H 25 mm. Silicon grease was applied to the inner walls of the container. The synthetic BW (ca. 12 g) was placed in the container and was heated by an infrared lamp. Specimens of Ø 38 mm×H (7–9) mm were cast, and the silicon grease on the specimens was wiped off with a paper. The entire surface of the specimen was brought in contact with water.
The specimen of the synthetic BW was placed in a plastic bottle and soaked in 100 cm3 of de-ionized water. The plastic bottle was then inserted in a constant-temperature water bath at (25 ± 1) °C. An appropriate volume (5–10 cm3) of the leaching solution was sampled for analysis for 1–45 days. After sampling, the corresponding volume of de-ionized water was added. The concentrations of Na+, NO3−, and NO2− in the leaching solution were measured by ICP-AES and ion chromatography. The concentration of the components in the leaching solution at a certain time is labeled Ct.
Dried specimens of the synthetic BWs were cut before and after the aqueous leaching tests. The cross-section near the surface of the specimen was observed by scanning electron microscopy (SEM). The 100× magnification was chosen, and the beam voltage was set at 1 kV in order to avoid melting of the BW. Pressure and temperature in the observation room were continuously kept 10−7 Pa and room temperature, respectively.
3. Equations of diffusion
3.1. Diffusion in a solid for molecular diffusion
Leaching from solidified wastes is normally considered as a physical process [Citation12]. Regarding solidification of salts in a BW, it is assumed that the salts are immobilized in bitumen without chemical bonding. When the BW is brought in contact with water, a component of the salts dissolved by water can be transported in the BW by diffusion. The diffusion of the component in one direction can be described by Fick's law as shown below:
(1) where J is the flux of the component in the x-direction in the BW, D is the diffusion coefficient of the component in the BW (based on molecular diffusion), C is concentration of the component, and X is the distance in x-direction.
A simple leaching model was used for the evaluation of leaching of a component for the BW [Citation7,8]. This simple leaching model assumes that no interaction occurs between the bitumen and the component, that the concentration of the component at the interface between the solid and solution is zero (infinite bath assumption), and that the component is homogenously distributed in the BW before leaching. The solution of the mass transport equations for diffusion of the component from a semi-infinite medium yields [Citation12,13]
(2) where S and V are the bulk surface area and bulk volume of the BW, which are calculated from the diameter and the height of the specimen, respectively. In this study, the leaching property of the BWs was evaluated by Equa-tion (2) as well as the references [Citation7,8].
3.2. Diffusion in porous media
The part of a BW, in which soluble salts are leached in contact with water, can be regarded as a porous medium [Citation14]. Fick's law can be applied to diffusion through this porous medium. For diffusion of the component through the porous medium, the proportionality factor in Equation (1) is not shown as the diffusion coefficient based on molecular diffusion, but as an effective diffusion coefficient [Citation12,Citation15],
(3) where De is effective diffusion coefficient in the BW regarded as a porous medium in which chemical interaction (e.g. adsorption and desorption of the ions to inner surface of the path) does not occur. The effective diffusion coefficient generally depends only on the physical properties such as porosity, tortuosity, and constrictivity. If all the pores are connected and parallel, the effective diffusion coefficient De can be simply related to the diffusion coefficient,
(4) where ϵ is the porosity, δ is the constrictivity, τ is the tortuosity factor, and D0 is the diffusion coefficient of a component in the water filling pores.
4. Results and discussion
4.1. SEM observation of synthetic bituminized wastes
The cross-sectional SEM images of BWAD, BW45, BW10, and BW55 are shown in . Since BW10, BW25, BW40, BW55, and BW70 were produced by the same solidification method (see Section 2.1), the salt size and salt dispersion for BW25, BW40, and BW70 were similar to those for BW10 and BW55. On the SEM images, no inner pores or cracks working as paths for leaching of the water and the soluble components were observed before the leaching test. The BWAD and BW45 were produced using the simulated solution, whereas the BW10 and BW55 were produced using NaNO3 powder (see Section 2.1). The salt particle sizes in BWAD, BW10, and BW55 were comparable, and smaller than 40 μm as shown in (a), 1(c), and 1(d), respectively. The particle sizes and shapes of the salt in BW10 and BW55 remained the same after the preparation process of heating and mixing with the NaNO3 powder. In contrast, the particle sizes of the salts in BW45 varied, and the maximum size was 250 μm as shown in (b), indicating that difference in the particle sizes of the salts in the BWAD was due to mixing methods of the simulated solution with bitumen (see Section 2.1).
Figure 1. SEM images of the cross-sections of synthetic BWs. (a) BWAD, (b) BW45, (c) BW10, and (d) BW55.
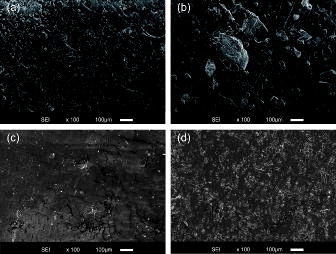
The cross-sectional SEM images of the synthetic BWs after the leaching test are shown in . No salt was observed in the synthetic BWs, and micropores appeared after leaching the salts. The sizes of the micropores for BWAD, BW45, BW10, and BW55 after the leaching test were up to 100, 300, 50, and 50 μm, respectively, which were determined as the average of the major axis and minor axis of the pores as an ellipse. The sizes of the micropores for BWAD, BW45, BW10, and BW55 grew approximately 2.6, 1.2, 1.3, and 1.3 times, respectively, as large as the particle sizes of the salts before the leaching test. It was observed that the specimen of the leached BWAD also swelled. The growth of micropores could occur according to additional water uptake in a high-concentrated liquid solution, which was generated by dissolution of the soluble salts, based on osmosis effect. The growth rate of the size of micropores in the soluble salt in the BWAD was larger than that in the BW45, BW10, and BW55. This means that the sizes of the micropores reflect the penetrability of the bitumen (i.e. hardness), since the penetrability of the AD Compound was approximately twice as high as that of the Blown Bitumen 20/30 (). In addition, the shapes of the micropores of BWAD, BW45, and BW55 are not spherical, but the shape of the micropores of BW10 does seem to be spherical. In the BW with a low salt content (e.g. BW10), because a micropore had a small amount of other micropores around itself, the growth of this micropore was not influenced by that of other micropores, and then this micropore was homogenously expanded and kept spherical in shape. In contrast, in the BW with a high salt content (e.g. BW55), many micropores existed in a small area, homogenous expansion of micropore interfered each other, and the shape of micropore was deformed.
4.2. Diffusion coefficient of soluble component in synthetic bituminized waste
illustrates the relationship between the leaching fractions (Ct/C0) of the soluble components (Na+, NO3−, and NO2−) and the square root of time. The lines were derived by the least-squares regression using the leaching fractions with a good correlation (i.e. the correlation coefficient > 0.99) during the early period. The Ct/C0 of the components increased with increasing time, and was proportional to the square root of time in the comparatively early periods. As time passed, the Ct/C0 of the soluble components was gradually deflected away from the lines. As a possible reason for the deflection, the solution of which the concentration was similar to that of the leaching solution might increase in the micropores in the BW, when many components were leached. The linear relationship during the early periods could be explained by Equation (2). The diffusion coefficient D could be calculated from the gradients of the lines.
Figure 3. Relationship between the leaching fraction (Ct/C0) and the square root of time of the soluble components for (a) BWAD and (b) BW45. □: Na+, ◯: NO3−, and ♦: NO2−.
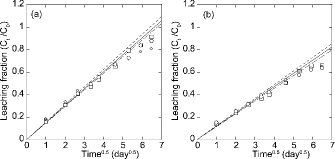
The diffusion coefficients D of the soluble components (Na+, NO−3, and NO−2) are summarized in . No remarkable difference was observed in the D values of the components in each BW. The agreement of the D values of the components in each BW may suggest that chemical interaction between the soluble components and the inner surface of the path in which the soluble component transferred did neither occur nor were different among the ionic species. The obtained D values were also influenced by many factors such as the dissolution rates of salts, the relation of the ion size and the pore size, and so on. The water-soluble salts (NaNO3 and NaNO2) are easily dissolved in water. Moreover, the inter-diffusion coefficients of the water-soluble salts and water at 25 °C are approximately 10−9 m2/s [Citation16], which is significantly faster than diffusion of the soluble components (Na+, NO−3, and NO−2) in the synthetic BWs (1×10−12 m2/s). This means that dissolution of the soluble salts is not one of the rate-controlled steps for diffusion of the ions in the BWs. For the sizes of the ions and the micropore, it is clear that the sizes of micropores are significantly larger than that of the ions.
Table 3. Diffusion coefficient of sodium, nitrate, and nitrite in the synthetic BWs at 25 °C.
The D value of Na+ in the BW40 was also determined and compared with those in the previous reports by the synthetic COGEMA-type BW [Citation7] and Eurobitum BW [Citation8] in . Nakayama et al. [Citation7] investigated the leaching behavior of Na+ in the synthetic COGEMA-type BW using de-ionized water at 25 °C. The synthetic COGEMA-type BW contained 60 wt.% of straight bitumen 60/80 (penetrability = 43 ± 9) and 40 wt.% of salts. The salts in the synthetic COGEMA-type BW consisted of 44 wt.% NaNO3, 50 wt.% (Ba,Sr)SO4, and 6 wt.% CsI. The D value of Na+ in the synthetic COGEMA-type BW after swelling obtained from Equation (2) was 3×10−13 m2/s. Since the soluble components leached from the synthetic COGEMA-type BW in two stages (before swelling and after swelling), the D value in the synthetic COGEMA-type BW could not be simply compared with that obtained in this study. However, swelling of the BW should occur as well as water uptake and leaching of the soluble components, and therefore, the D value in the synthetic COGEMA-type BW after swelling was used. Sneyers and Van Iseghem [Citation8] obtained the D value of Na+ in Eurobitum BW in contact with clay water (pH 7.0–7.5) at 23 °C. Eurobitum BW contains 60 wt.% of Mexphalt R85/40 bitumen (penetrability = 40–50) and 40 wt.% of salts. The compositions of the salts in Eurobitum BW were 75 wt.% NaNO3, 13 wt.% CaSO4, and 12 wt.% insoluble salts [Citation6,Citation8]. The D value of Na+ in Eurobitum BW was 1.4×10−13 m2/s. In this study, the D value of Na+ in BW40 was (3.0 ± 2.2)×10−13 m2/s. The D values of Na+ in the synthetic COGEMA-type and Eurobitum samples were similar to that in BW40, although there is a difference in the bitumen matrix types and salt compositions among the BWs. Moreover, the D values of the soluble components between BWAD and BW45 agreed well; nevertheless the basic characteristics (e.g. bitumen matrix, particle size of the salt ranging 250 μm, and salt composition) were different between BWAD and BW45 as shown in and and . Consequently, even if the bitumen matrices and the salt composition were different among the BWs, when the total salt content was the same, the D values of the components were in the same range. If the components transfer only through micropore, the D values for their BWs should change depending on the soluble salt content (i.e. the D values: BW40 > Eurobitum BW > synthetic COGEMA-type BW). However, the fact that the agreement of the D values for BW40, Eurobitum BW, and synthetic COGEMA-type BW suggests that water and the soluble components could transfer not only through the micropores, but also through the contact area between the insoluble salts and the bitumen.
Table 4. Diffusion coefficient of Na+ obtained in the aqueous leaching test of the bituminized wastes.
4.3. Effect of salt content on diffusion of soluble component in synthetic bituminized waste
We attempted to evaluate the effects of salt content on the leaching properties of the synthetic BWs. The D values in BW10, BW25, BW40, BW55, and BW70 could be determined by Equation (2), but it is not yet clear whether the components could be diffused in the BWs only through molecular diffusion, because many micropores were formed in the leached BWs from the SEM observation as shown in . If the BWs are regarded as porous media, the diffusion of the components in the BWs should be evaluated based on the effective diffusion coefficient De in Equation (4). Here, porosity can be assumed as a volume fraction of micropores. The volume of micropores in the BWs was calculated from the salt content, the densities of NaNO3 (2.26 g/cm3) and the Blown Bitumen 20/30 (1.03 g/cm3), and the growth rate of the micropore size. The latter occurred by swelling for the Blown Bitumen 20/30 by up to 1.3 times (see Sec-tion 4.1). The volume fraction of micropores was acquired by dividing the volume of micropores by that of the synthetic BWs.
The diffusion coefficient D of Na+ in the synthetic BWs (BW10, BW25, BW40, BW55, and BW70) was plotted against the volume fraction of micropores in . The vertical and horizontal errors of the plots show the standard deviation (n = 3–5) of the D values and the range of growth of micropores by swelling (1–1.3 times), respectively. The D values in the synthetic BWs were proportional to the volume fraction of micropores in the double-logarithmic plot. As shown in , it was found that the salt content in the synthetic BWs was clearly correlated with the diffusion coefficient D.
Figure 4. Relationship between the diffusion coefficients of Na+ in the synthetic BWs and the volume fraction of the micropores.
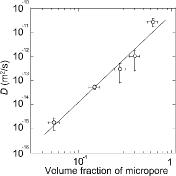
When the pore size is large enough for the diffusion ions, “δ/τ2” in Equation (4) can be empirically approximated “1/τ”. Because it was apparent that pore size in the BW was large enough by the SEM observation, Equation (4) was approximated as the following equation:
(5)
The lines in were derived by the least-squares regression for the diffusion coefficient D of the synthetic BWs and the volume fraction of the micropores. A good correlation between the diffusion coefficient D and the volume fraction of micropores (ϵ) was observed to follow
(6)
Equation (5) can be rewritten as log (De) = log (ϵ) + log (D0/τ). If D can be regarded as De, the proportional coefficient of log (ϵ) in Equation (6) should be 1. Given the large difference between 1 and 3.7, the diffusion coefficient D could not be replaced by the effective diffusion coefficient De. This large difference means the micropores were not connected and parallel in the leached BWs, and diffusion of the components in the BW could not simply show diffusion in porous media. The diffusion of the component in the leached BW would be influenced by alteration of pore structure by the growth of micropores.
We found that the diffusion of the components in the BW was affected by the salt content. However, the diffusion mechanisms of the components in the BWs have not yet been fully understood. Based on the results of the present work, the schematic images of the diffusion of a component in the BW were shown in . Swelling behavior of the synthetic BW was added in the schematic images described based on several reports as a reference [Citation4,5,Citation9]. The white line in the BW shows the concentration of the component along a path from the center in the BW to the interface between the BW and the water. The small and large white dots in the BW represent salts and micropores, respectively. The square including black dots in the waterside indicates the concentration of the component leached from the BW. When the BW begins to be in contact with water (t = t0), the BW has the initial concentration of the component (C0) and homogenous salt dispersion, and the salts have not been leached in water yet. After the BW gets in contact with water (t = t1), the micropores are formed by the leaching of salts in the near-surface BW. The BW consists of salt and micropore regions at t1. The salt region is a part of the BW in which water is penetrating and the dissolved salts are slowly diffused to the micropore region. The micropore region is a part of the BW in which almost all of the salts are already leached out. The component is diffused from the salt region to the waterside by a concentration gradient. The diffusion behavior is probably different in each region. The distance from the center in the BW to the interface between the BW and the water becomes long by the growth of the micropore region (i.e. X1 > X0). The concentration of the component in water (Ct) increases with increasing leaching amounts of the component from the BW (Ct = C1 > 0). The concentration of the component at the interface varies according to the concentration in the water. When the BW is brought in contact with water at t2 (t2 > t1) (see ), the volume of the BW with micropores grows by leaching of more salts, and the concentration of the components in the water increases (C2 > C1). The distance from the center in the BW to the interface also becomes longer (X2 > X1). Consequently, the salt content would influence the number of micropores, the growth of micropore, and the shape of swelled micropores in the micropore region as shown in . In this study, strong effect of the salt content on the D value was found. Therefore, in order to understand the diffusion of the components in the BW, it is important that diffusion of the components in the both micropore and salt regions is individually determined.
Figure 5. Schematic images of the diffusion of a component in the BW (t: time, t2 > t1 > 0; X: distance from the center of the BW to interface between the BW and water in one direction, X2 > X1 > X0; Ct: concentration of a component leached from the BW in time, C2 > C1 > 0; C0: initial concentration of a component in the BW).
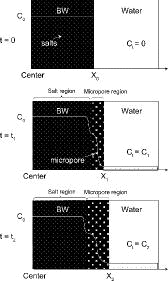
5. Conclusion
We conducted the aqueous leaching test for the synthetic BWs. The cross-sectional synthetic BWs before and after the aqueous leaching test were observed by using SEM. From the results in the leaching test, the diffusion coefficient D was obtained by Fick's law, and the effects of salt contents on the D value were investigated. The results are summarized as follows.
It was found that the penetrability of bitumen and the salt content affected the size and shape of the micropores.
It was found that the D value in the synthetic BW did not change among the ionic species (Na+, NO−3, and NO−2) and the BWs with the same salt content (i.e. BW40, synthetic COGEMA-type BW, and Eurobitum BW).
The diffusion coefficient D for the BWs was proportional to the volume fraction of micropores in a double-logarithmic plot. It was concluded that the salt content was one of the key factors in the diffusion of a component in the BW.
The leaching mechanisms in the BW are complex, since the leaching of the component includes several processes such as water penetration, dissolution of salts, diffusion of water and the component in both the salt region and the micropore region, etc. To clarify the leaching mechanisms of the BW, a precise determination of the diffusion coefficients of components in each region is required separately for the BWs with various salt contents.
Acknowledgements
The authors are grateful to Mr. Yoshinaka (Japan Atomic Energy Agency) for providing the synthetic bituminized nonradioactive waste with the AD Compound.
References
- Japan Atomic Energy Agency and the Federation of Electric Power Companies of Japan. Second progress report on research and development for TRU waste disposal in Japan – repository design, safety assessment and means of implementation in the generic phase. JNC TY1400 2005-013. Japan: Japan Atomic Energy Agency; 2005. Japanese.
- Yoshinaka K, Takano Y, Kimura Y, Sugaya A, Onizawa T. Leaching test of bituminized waste and waste solidified by epoxy resin. JAEA-Technology 2008-063. Japan: Japan Atomic Energy Agency; 2008. Japanese.
- Morgan P, Mulder A. The Shell Bitumen industrial handbook. UK: Shell Bitumen; 1995.
- Sercombe J, Gwinner B, Tiffreau C, Simondi-Teisseire B, Adenot F. Modelling of bituminized radioactive waste leaching. Part I: constitutive equations. J Nucl Mater. 2006;349:96–106.
- Gwinner B, Sercombe J, Tiffreau C, Simondi-Teisseire B, Felines I, Adenot F. Modelling of bituminized radioactive waste leaching. Part II: experimental validation. J Nucl Mater. 2006;349:107–118.
- Valcke E, Marien A, Smets S, Li X, Mokni N, Olivella S, Sillen X. Osmosis-induced swelling of Eurobitum bituminized radioactive waste in constant total stress conditions. J Nucl Mater. 2010;406:304–316.
- Nakayama S, Iida Y, Nagano T, Akimoto T. Leaching behavior of a simulated bituminized radioactive waste form under deep geological conditions. J Nucl Sci Technol. 2003;40:227–237.
- Sneyers A, Van Iseghem P. The leaching behavior of bituminized radioactive waste in the geologic disposal conditions of the boom clay formation. In: McKinley IG, McCombie C, editors. Scientific basis for nuclear waste management XXI (Mat. Res. Soc. Symp. Proc. 506). Warrendale (PA): Material Research Society; 1998. p. 565–572.
- Gwinner B, Sercombe J, Simondi-Teisseire B, Adenot F, Felines I, Favre E. Experimental and theoretical study of the pore structure and diffusion properties of an evolving heterogeneous material: application to radioactive bituminized waste. Chem Eng Commun. 2007;194:234–247.
- Nuclear Waste Management Organization of Japan. Disposal technology and safety regarding low-level radioactive wastes in a geological site. NUMO-TR-10-03. Japan: Nuclear Waste Management Organization of Japan; 2011. Japanese.
- Suzuki H, Miura A. Experiment using laboratory scale extruder – fluid behavior in twin-screw extruder. JNC TN 8410 99-041. Japan: Japan Nuclear Cycle Development Institute; 1999. Japanese.
- Batchelor B. Leach models: theory and application. J Hazard Mater. 1990;24:255–266.
- Crank J. The mathematics of diffusion. 2nd ed. London: Oxford University Press; 1975.
- Mokni N, Olivella S, Valcke E, Marien A, Smet S, Li X. Deformation and flow driven by osmotic processes in porous media: application to bituminised waste materials. Transport Porous Media. 2011;86:665–692.
- Cussler EL. Diffusion: mass transfer in fluid systems. 3rd ed. Oxford: Clarendon Press; 2008.
- The Chemical Society of Japan. Handbook of Chemistry. Rev. 5th ed. Tokyo (Japan): Maruzen Ltd.; 2004: II-65. Japanese.