Abstract
Cesium was recovered from plant samples obtained from Fukushima prefecture. The isotopic ratios of 134Cs/137Cs and 135Cs/137Cs were analyzed by thermal ionization mass spectrometry with a single filament method using a TaO activator. Samples containing 5 Bq of 137Cs were analyzed with typical analytical errors of approximately 0.5% for 134Cs/137Cs and approximately 0.1% for 135Cs/137Cs. Measurements of both ratios showed profiles that were characteristic of the measurements of among other environmental samples reported in the literature. The results showed the isotopic ratios of 134Cs/137Cs and 135Cs/137Cs were applicable for the source analysis of radioactive Cs in Fukushima prefecture.
1. Introduction
Large amounts of radioactive nuclides as both fission products and nuclear fuel materials were released because of the Fukushima Daiichi Nuclear Power Plant (FDNPP) accident. Radioactive Cs releases, generated as fission products and neutron-activation products, have exceeded the peta-Bq level [Citation1]. Many studies of radioactive Cs were performed to estimate external and internal exposures and to analyze the source of radioactive nuclides [Citation2–4]. These studies were typically performed by γ-ray spectrometry of 134Cs (T1/2 = 2.06 y) and 137Cs (T1/2 = 30.2 y).
Although mass spectrometry has been used for the analysis of radioactive nuclides and actinides, few studies have reported the analysis of radioactive Cs. Because the generation of radioactive 135Cs depends on the neutron flux, the isotopic ratio of 135Cs (T1/2 = 2.3 × 106) and 137Cs is used for analyzing the operations of nuclear facilities [Citation5–9]. This suggests that the isotope ratio analysis of the 135Cs and 137Cs by mass spectrometry may also provide important information for the source analysis of radioactive nuclides in the contaminated areas of Fukushima prefecture, though to date there have been no reports in the literature concerning this.
The purpose of the present study was to apply thermal ionization mass spectrometry (TIMS) to analyze the isotopic ratios of 134Cs/137Cs and 135Cs/137Cs in environmental samples obtained from Fukushima prefecture for assessing the safety and determining the source of radioactive Cs. As an initial step in the isotope ratio analysis of radioactive Cs, we determined the applicability of TIMS to analyze 134Cs/137Cs and 135Cs/137Cs in the plant samples for studying the fate of radioactive Cs in the biosphere.
2. Experimental
2.1. Sample preparation
Environmental samples, such as grasses, moss, and bark, were obtained from in four points showing high dose rate (over 12 μSv/h in August 2011) of public areas of Fukushima prefecture (). The samples were washed with pure water and dried at 373 K. Some portions of the dried material (∼2.5 g) were dry-ashed at 873 K, and then immersed in 14 M HNO3 and evaporated to dryness. Diluted HNO3 was added after the evaporation step and the insoluble residues were removed by centrifugation at 3000 rpm for 10 minutes. The resulting supernatant was added to a TeflonTM beaker, which was heated to dryness. The final residue was dissolved in 50 mL of 0.1 M HNO3 for the recovery of Cs.
Table 1. Samples ID, location, and type for Cs isotope ratio analysis.
2.2. Recovery of cesium
The cation-exchange resin DOWEXTM 50WX8 (100–200 mesh), the anion-exchange resin DOWEXTM 1 × 8 (100–200 mesh), and ammonium phosphomolybdate (AMP) were used for the recovery of Cs.
The cation-exchange resin initially conditioned with HCl, and a 4 mL portion of conditioned resin was added to a mini column. A 30 mL of 0.1 M HNO3 was added to the resin as a final conditioning step. The sample solution was then poured into the column and followed by a 50 mL of 3 M HNO3 to elute alkali metal elements and residual high valence elements such as alkaline earth metals, transition metals, and transuranium elements. The pH of the eluted solution was adjusted to 2.0, and 0.1 g of AMP was added to the solution. After stirring for several hours, the supernatant was removed from the mixed solution by centrifugation. A 20 mL of 7 M ammonium hydroxide solution was used to dissolve the residue for subsequent anion-exchange ion chromatography.
After conditioning with ammonium bicarbonate following methods [Citation8], a 4 mL portion of the anion-exchange resin was added to a mini column, followed by pure water as a final conditioning step. The sample solution was added to the column, and the resulting eluate was collected and heated to dryness. The residue was dissolved in a 30 mL of 0.1 M HNO3, and the sample solution for Cs analysis was obtained by final purification following the previously described cation-exchange method.
2.3. Analysis of cesium
The total concentration of radioactive Cs was measured by γ-spectrometry. Isotopic ratios of Cs were measured by TIMS (TritonTM T1, Thermo Fisher Scientific) using a single filament method. The sample solution was prepared as 5000 Bq mL−1 of 137Cs in 1 M HNO3. A 1 μL aliquot of each solution containing 5 Bq of 137Cs was loaded onto a rhenium filament with a TaO activator [Citation10]. The filament current was approximately 1 A, and the mass spectrum was obtained with a secondary electron multiplier detector because of the low total amounts of radioactive Cs loaded onto the filament.
3. Results and discussion
3.1. Mass spectrometry of cesium
Three peaks, representing 134Cs, 135Cs, and 137Cs, were observed on the typical mass spectra in this study (). When studying Cs isotopes by mass spectrometry of Cs, isobaric interferences with Ba must be considered [Citation5–9]. Although a higher filament current would enhance the sensitivity of Cs isotope analysis, the typical filament current both of this study and other reports [Citation5–9] was set to approximately 1 A to avoid the isobaric interference of Ba. The mass spectrum obtained in this study shows no peak at atomic mass of 138 (), which indicated that no Ba ion was detected during our measurement.
A TaO activator was used to assist in the formation of strong and stabilized ion beams. Use of a TaO activator was initially reported for the isotope ratio analysis of Sr and Rb [Citation10], and the application has since been reported for other elements such as Ca [Citation11], Ra [Citation12], and Nd [Citation13]. In the current study, the use of a TaO activator resulted in at least 100 times increase in the intensity of ion beams.
The effect on analytical precision from varying of the amounts of Cs loaded on the filament for the analytical precise was studied (). The standard error in ± 2SE was observed to decrease with increasing amount of Cs. When 5 Bq of 137Cs (equal to ∼1.6 pg) was loaded on the filament, the isotopic ratio was analyzed with average analytical errors of ∼0.5% for 134Cs/137Cs and ∼0.1% for 135Cs/137Cs. The average reproducibility of three sample runs was observed to agree within the analytical error (). The significant figure of the calculation results using codes such as ORIGEN-II was less than four orders of magnitude, which meant that the analysis of the isotopic ratio with 5 Bq of 137Cs was sufficient for the source analysis of radioactive Cs.
Table 2. Reproducibility of Cs isotope ratio analysis.
Figure 2. Analytical precision of Cs isotope ratio as a function of amount of Cs loaded on filament (in terms of 137Cs).
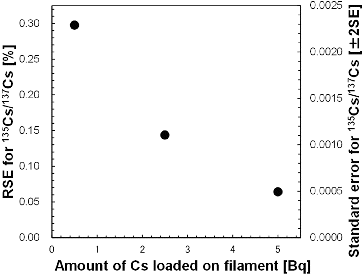
Because there is no standard material for the isotope ratio analysis of Cs by mass spectrometry, the instrumental mass fractionation was not monitored in the current study, and thus, the isotopic ratios were not corrected for mass fractionation. Therefore, the isotopic ratio of 134Cs/137Cs of the OKMDNP sample by TIMS was compared to the results obtained by γ-ray spectrometry (). The observed 134Cs/137Cs isotope ratio obtained by TIMS appeared to agree with the results from γ-ray spectrometry within experimental error.
Table 3. Comparison of 134Cs/137Cs ratio analyzed by γ-ray spectrometry and thermal ionization mass spectrometry.
3.2. Analysis of cesium isotopic ratios in environmental samples
The results of the isotope ratio analysis of environmental samples obtained from Fukushima prefecture were compared to the radiation dose contour map () as reported by Ministry of Education, Culture, Sports, Science, and Technology (MEXT) (as in August 2011) [Citation14].
Figure 3. Results of Cs isotope ratio analysis obtained from environmental samples in Fukushima prefecture. Contour lines of radiation dose rate were made using published data from MEXT [Citation14]. Errors represent here mean ± 2SE. Value in square brackets represent time-corrected radioactive ratios.
![Figure 3. Results of Cs isotope ratio analysis obtained from environmental samples in Fukushima prefecture. Contour lines of radiation dose rate were made using published data from MEXT [Citation14]. Errors represent here mean ± 2SE. Value in square brackets represent time-corrected radioactive ratios.](/cms/asset/038f3890-8591-4053-915a-e60452668778/tnst_a_891954_f0003_oc.jpg)
The isotopic ratio of 134Cs/137Cs by TIMS ranged from 0.0301 to 0.0318; these values were expressed in the time-corrected radioactive (Bq) ratio (referred to as the 134Cs/137Cs activity ratio), which ranged from 0.999 to 1.054. This range was significantly greater than the activity ratio of global fallout (reported as ∼0.0002 corrected on 11 March 2011) [Citation15], and agreed with γ-ray spectrometry results reported in the literature [Citation3].
When comparing the observed variations of 134Cs/137Cs activity ratios between the different environmental samples (), it was observed that the ITT07 sample (whole grass) had the highest 134Cs/137Cs activity ratio value of 1.054 ± 0.005, while the ratio of sample FTBR35 (grass roots) had the lowest value observed at 0.999 ± 0.012. The ratios of the OKMDNP (moss) and FTB01 (bark) samples were observed to fall between these two values.
The amount of 134Cs and 137Cs in each reactor has been calculated by the ORIGEN-II code [Citation16]. Using this information, the individual 134Cs/137Cs activity ratios of each reactor were estimated as follows: 0.945 for reactor 1, 1.087 for reactor 2, and 1.049 for reactor 3. Although this trend agreed with γ-ray spectrometry measurements of polluted water in each reactor [Citation3], where the activity ratio for reactor 1 ranged from 0.89 to 0.93, for reactor 2 ranged from 0.96 to 1.05, and for reactor 3 ranged from 0.97 to 1.04, the data by γ-ray spectrometry showed a wider range.
By comparing the experimental results of this study with the calculated results, the following conclusions were made.
The 134Cs/137Cs activity ratio of ITT07 was largely affected by radioactive Cs in the second and/or the third reactor.
The main origin of radioactive Cs in the OKMDNP and FTB01 samples was the second and/or third reactor, though there could be a small contribution of radioactive Cs from the first reactor.
The 134Cs/137Cs activity ratio of FTBR35 suggested that radioactive Cs from all reactors were mixed in this site.
The isotopic ratios of 135Cs/137Cs in the environmental samples were also obtained by TIMS measurements (), and these isotopic ratios were observed to range from 0.3808 to 0.3896. Because these values were also much greater than reported isotopic ratios of global fallout (∼0.5 for Chernobyl accident [Citation8], and ∼2.7 for nuclear weapon testing [Citation17], corrected on 11 March 2011), these values could be useful for the source analysis of radioactive Cs in Fukushima prefecture. The observed isotopic ratios of 135Cs/137Cs and the activity ratios of 134Cs/137Cs were compared to the ratios calculated with the ORIGEN-II code and to reported 134Cs/137Cs activity ratios in polluted water ().
Figure 4. 135Cs/137Cs ratios as a function of 134Cs/137Cs activity ratios. Isotope error bars represent ± 2SE. Plus sign (+) indicates 134Cs/137Cs activity ratios were corrected on 11 March 2011 values. Single asterisk (*) represents calculation results from estimation of radioactive nuclides with ORIGEN-II code [Citation16]. Double asterisk (**) represents values reported for 134Cs/137Cs activity ratio in polluted water [Citation3].
![Figure 4. 135Cs/137Cs ratios as a function of 134Cs/137Cs activity ratios. Isotope error bars represent ± 2SE. Plus sign (+) indicates 134Cs/137Cs activity ratios were corrected on 11 March 2011 values. Single asterisk (*) represents calculation results from estimation of radioactive nuclides with ORIGEN-II code [Citation16]. Double asterisk (**) represents values reported for 134Cs/137Cs activity ratio in polluted water [Citation3].](/cms/asset/39f2a0dc-3f62-49fa-ac29-b6b253762fef/tnst_a_891954_f0004_oc.jpg)
The isotopic ratios of 135Cs/137Cs of all samples were higher than that of the calculation results of the second and third reactors. Therefore, the effect of the first reactor could not be ignored, even though the activity ratio of ITT07, which was the highest observed 134Cs/137Cs activity ratio among the samples, suggested that the main source of radioactive Cs of ITT07 was the second and/or third reactor as mentioned previously.
The isotopic ratio of 135Cs/137Cs in the FTB01 sample was similar to the OKMDNP sample, whereas the 134Cs/137Cs activity ratio of FTB01 was significantly lower than OKMDNP. This suggested that the contribution of radioactive Cs of each reactor to FTB01 was different than the contribution to OKMDNP.
The 135Cs/137Cs ratio in the FTBR35 sample was weighted towards the second and/or third reactor. This suggests that the radioactive Cs in FTBR35 was dominated by contribution of the second and/or third reactor, considering the error of the 134Cs/137Cs activity ratio. Therefore, a characteristic isotope profile was found in the environmental samples, even when the observed 134Cs/137Cs activity ratio was close to a value of ∼1.
According to these results, the isotopic ratio of 135Cs/137Cs may provide significant information for the analysis of radioactive Cs in Fukushima prefecture. However, more data are required to clearly distinguish the effects of the second and third reactors, and thus, future experiments will focus on the analysis of radioactive Cs recovered from other environmental samples in Fukushima prefecture to assist in the evaluation of the FDNPP accident.
4. Conclusions
Cesium was recovered from the plant sample obtained from Fukushima prefecture, and the isotopic ratios of radioactive 134Cs, 135Cs, and 137Cs were analyzed by TIMS. The results confirmed that a TaO activator was beneficial for Cs isotope ratio analyses, and loadings of 5 Bq of 137Cs produced typical analytical errors of ∼0.5% for 134Cs/137Cs and ∼0.1% for 135Cs/137Cs. The isotopic ratio of 135Cs/137Cs of each sample produced a unique profile, suggesting that it could provide important information concerning the source analysis of radioactive Cs released by the FDNPP accident.
Acknowledgements
The authors thank T. Takahashi, N. Sato, M. Tanigaki, Y. Kobayashi, A. Uehara, H. Yoshino, H. Yoshinaga, L. Belkacemi, M. Kowatari, M. Konno, and S. Matsuzaki for their support on this study.
Additional information
Funding
References
- Chino M, Nakayama H, Nagai H, Terada H, Katata G, Yamazawa H. Preliminary estimation of release amounts of 131I and 137Cs accidentally discharged from the Fukushima Daiichi Nuclear power plant into the atmosphere. J Nucl Sci Technol. 2011;48:1129–1134.
- Sakaguchi A, Kadokura A, Steier P, Tanaka K, Takahashi Y, Chiga H, Matsushima A, Nakashima S, Onda Y. Isotopic determination of U, Pu and Cs in environmental waters following the Fukushima Daiichi Nuclear power plant accident. Geochem J. 2012;46:355–360.
- Komori M, Shozugawa K, Nogawa N, Matsuo M. Evaluation of radioactive contamination caused by each plant of Fukushima Daiichi Nuclear power plant station using 134Cs/137Cs activity ratio as an index. Bunseki Kagaku. 2013;62:475–483. [in Japanese].
- Steinhauser G, Brandl A, Johnson TE. Comparison of the Chernobyl and Fukushima nuclear accidents: a review of the environmental impacts. Sci Total Environ. 2014;470–471:800–817.
- Karam LR, Pibida L, McMahon CA. Use of resonance ionization mass spectrometry for determination of Cs ratios in solid samples. Appl Radiat Isotopes. 2002;56:369–374.
- Pibida L, MacMahon CA, Busharw BA. Laser resonance ionization mass spectrometry measurements of cesium in nuclear burn-up and sediment samples. Appl Radiat Isotopes. 2004;60:567–570.
- Taylor VF, Evans RD, Cornett RJ. Preliminary evaluation of 135Cs/137Cs as a forensic tool for identifying source of radioactive contamination. J Environ Radioactivity. 2008;99:109–118.
- Delmore JE, Snyder DC. Tranter T, Mann NR. Cesium isotope ratios as indicators of nuclear power plant operations. J Environ Radioactivity. 2011;102:1008–1011.
- Snyder DC, Delmore JE, Tranter T, Mann NR, Abbott ML, Olson JE. Radioactive cesium isotope ratios as a tool for determining dispersal and re-dispersal mechanisms downwind from the Nevada nuclear security site. J Environ Radioactivity. 2012;110:46–52.
- Brick JL. Precision K-Rb-Sr isotopic analysis: application to Rb-Sr chronology. Chem Geol. 1986;56:73–83.
- Heuser A, Eisenhauer A, Gussone N, Bock B, Hansen BT, Nagler Th.F. Measurement of calcium isotopes(δ44Ca) using a multicollector TIMS technique. Int J Mass Spectrom. 2002;220:385–397.
- Yokoyama T, Nakamura E. Precise analysis of the 228Ra/226Ra isotope ratio for short-lived U-series disequilibria in natural samples by total evaporation thermal ionizatin mass spectrometry (TE-TIMS). J Anal At Spectrom. 2004;19:717–727.
- Chu Z, Chen F, Yang Y, Guo J. Precise determination of Sm, Nd concentrations and Nd isotopic compositions at the nanogram level in geological samples by thermal ionization mass spectrometry. J Anal At Spectrom. 2009;24:1534–1544.
- Available from: http://www.mext.go.jp/b_menu/shingi/chousa/gijyutu/017/shiryo/__icsFiles/afieldfile/2011/09/02/1310688_1.pdf (accessed on Dec. 16, 2013).
- Carbol P, Salotie D, Erdmann N, Nylen T, Betti M. Deposition and distribution of Chernobyl fallout fission products and actinides in a Russian soil profile. J Environ Radioactivity. 2003;68:27–46.
- Nishihara K, Iwamoto H, Suyama K. Estimation of fuel compositions in Fukushima-Daiichi Nuclear power plant. Japan: Japan Atomic Energy Agency; 2012, JAEA-Data/Code 2012-018 [in Japanese].
- Lee T, Ku TL, Lu HL, Chen JC. First detection of fallout Cs-135 and potential applications of 137Cs/135Cs ratios. Geochim Cosmochim Acta. 1993;57:3493–3493.