Abstract
To support the design and operation of the decontamination system using KURION media for the treatment of highly contaminated water accumulated in Fukushima Daiichi Nuclear Power Station, Central Research Institute of Electric Power Industry has urgently carried out many kinds of research and development programs to support the operation of the decontamination system using columns filled with three kinds of KURION media (H, AGH and SMZ). Since the contaminated water at Fukushima Daiichi Nuclear Power Station contained seawater and oil, the effects of sea salt and dissolved oil on Cs adsorption behavior were examined closely by batch type. The concentration of sea salt in the solutions was varied between 0.0 and 3.4 wt%. The Cs adsorption capacity of KURION herschelite in seawater decreased to nearly 1/10th of that in pure water, but it was still concluded that herschelite has sufficient adsorption capacity to remove Cs from the contaminated water. The effect of dissolved oil could be ignored because of its low solubility in seawater. Langmuir-type adsorption isotherm equations, which can be applied for estimating Cs adsorption in sea salt containing water, were developed.
1. Introduction
Since the accident at Fukushima Daiichi Nuclear Power Station (NPS), the injection of cooling water into the reactors has been continuous and a large amount of highly contaminated water leaked from the damaged reactors has been accumulated on the site. Radioactive materials in the contaminated water should be separated and the decontaminated water returned to the reactor as cooling water so that the accumulation of contaminated water could be minimized and environmental contamination due to leakage of the contaminated water prevented.
Among the radioactive elements in the contaminated water, Cs is one of the major sources of radiation emission and, zeolite, which was used in the liquid waste treatment after the Three Mile Island (TMI) Unit 2 accident, is regarded as the best material for separating Cs from the contaminated water by adsorption [Citation1,2]. However, the contaminated water at Fukushima Daiichi NPS contained a large amount of seawater because seawater was initially injected into the reactors as cooling water as an emergency measure. Seawater also entered the turbine building as a result of tsunami, and heavy oil stored in tanks near the building as well as turbine oil also got mixed with the contaminated water.
KURION herschelite-type zeolite was selected for the radioactive wastewater treatment at Fukushima Daiichi NPS [Citation3]. This zeolite was modified to exhibit high Cs adsorption performance in highly concentrated Na solution; however, it was not known if herschelite would work well in the seawater contaminated by the oils. Therefore, in Japan, Tokyo Electric Power Company (TEPCO), Japan Atomic Energy Agency, Toshiba and Central Research Institute of Electric Power Industry (CRIEPI) evaluated the performance of KURION herschelite in the oil-contaminated seawater using several kinds of herschelite samples supplied by KURION.
Fundamental data on the herschelite-type zeolite, which was applied using the KURION decontamination system, were indispensable for estimating the system design proposed by KURION.
2. Overview of the contaminated water treatment system in Fukushima Daiichi Nuclear Power Station
The process flow diagram of the contaminated water treatment system presented by TEPCO [Citation4] is shown in . As the water was contaminated with turbine oil and sea water, an oil separator and a desalination plant were employed. A throughput as high as 1200 m3/day of contaminated water was required depending on the amount of accumulated water and on the amount necessary to cool the damaged reactors. The system consisted of an oil separator installed by Toshiba, a cesium adsorption device installed by KURION, a coagulating sedimentation device installed by AREVA and a desalination plant installed by Hitachi GE. CRIEPI was asked to perform supporting research and development on the KURION “adsorption” system, in which most of the radioactive Cs was expected to be removed, because CRIEPI has extensive experience in developing zeolite column systems for pyro-reprocessing, in which fission products are removed from spent molten chloride salt used in electrorefining [Citation5].
Figure 1. Diagram of water treatment system for highly contaminated water accumulated in Fukushima Daiichi NPS.
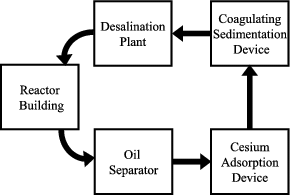
The system diagram of one unit of the Cs adsorption instrument is shown in [Citation6]. The Cs adsorption instrument consisted of three skids, i.e., the surfactant-modified zeolite (SMZ) skid for removing oil and Te, the H (herschelite) skid for removing Cs, and the silver-impregnated engineered herschelite (AGH) skid for removing I. The H skid consisted of four columns, three of which are in service and one is a spare containing fresh media for the operation [Citation7]. The Cs adsorption instrument consisted of four units operated in parallel. Since a maximum throughput of 1200 m3/day was required, each unit should have a throughput of 12.5 m3/h.
3. Experimental
3.1. Materials
All kinds of media applied to the Cs absorption instruments were supplied by KURION, immediately after the decision to adopt KURION system was made. Adsorption tests with SMZ, H and AGH produced by KURION [Citation8] were carried out by CRIEPI. A surface-modified hereschlite (KH) by potassium hexacyanoferrate (KCCF) was also used in the adsorption test.
3.2. Solution
The radioactivities of Cs-134 and Cs-137 in the contaminated water sampled at the turbine building were similar at about 2.0 × 106 Bq/ml [Citation9]. The total concentration of Cs in the contaminated water was calculated to be about 1.0 wt. ppm on the basis of the specific radioactivities of Cs-134 and Cs-137.
The initial Cs concentration in the solutions for the batch-type adsorption test was determined so that the final Cs concentration in the solution in the equilibrium state would remain in the range between 1.0 × 10−7 and 1.0 × 10−1 mmol/ml. Then, the initial Cs concentration was set to be between 2 and 3000 ppm considering the solution volume and the detection limit of Cs. As the source of Cs, CsCl powder of 99.9% purity, purchased from Wako Pure Chemical Industries, was dissolved in each test solution.
Since there was initially no information on the sea salt concentration in the contaminated water, it was assumed that the contaminated water had a similar composition to actual seawater in the most severe case. In the present study, instead of using actual seawater, simulated seawater was prepared using commercial salt for synthetic seawater named reef crystals (aquarium systems). The composition of major elements (Na, Mg, Ca, K, Sr) in the synthetic seawater was found to be the same as that of natural seawater in our ongoing another study to estimate the effect of these elements on Cs adsorption. The sea salt concentration in the solution was varied from 0.0 wt% (containing no sea water) to 3.4 wt% (corresponding to actual seawater) to evaluate the effects of sea salt on the Cs adsorption properties of each herschelite.
Additionally, no information was available on the type or amount of oil in the contaminated water. Therefore, some solutions were prepared by gradually mixing water with commercial turbine oil (FBK turbine oil, JX Nippon Oil & Energy Corporation) or heavy oil (Marine T103, JX Nippon Oil & Energy Corporation) in 200-ml glass bottles on a shaking table for 6 days. Then, appropriate amount of CsCl was dissolved in the solutions for use in the adsorption study.
3.3. Adsorption test method
The procedure for the batch-type adsorption test was as follows:
About 0.1 g of herschelite and 10 ml of each sample solution were placed in a centrifugation tube, so that the solution volume/solid weight ratio (V/S) was 100 ml/g.
The tubes were fixed on a shaking table.
Herschelite and a sample solution were mixed for 72 h at about 60 cycles/min at room temperature.
After mixing, the sample solution was separated from herschelite using a filter of 0.45 μm pore size.
The Cs concentration in the solution was measured using an atomic absorption spectrometer (Thermo Fisher Scientific, S4).
The concentration of Cs in the analyzed solution was adjusted to be about 2.0 ppm and the atomic absorption spectrometer can measure nearly 0.1 ppm. Thus each data might include 5% error.
Some adsorption tests were carried out for 1–4 days, and an equilibrium state was attained after 3 days (72 h). In some conditions, more than two test samples were prepared and the replicate of the adsorption experiments was confirmed from the obtained data.
4. Results
4.1. Cs adsorption properties of each herschelite in pure water and seawater
The Cs loading (Q) in hershelite was calculated from the Cs concentrations of the solution before and after contact with hershelite using the following equation:
(1) where C0 is the initial Cs concentration (mmol/ml) in the experimental solution prior to contact, C1 is the equilibrium Cs concentration in the solution after contact (mmol/ml), V is the volume of solution (ml) and M is the mass of herschelite (g).
The results of Q in each herschelite sample in the equilibrium state are shown in for pure water and in for the seawater.
Figure 3. Cs loading as function of equilibrium Cs concentration for KURION herschelite in pure water.
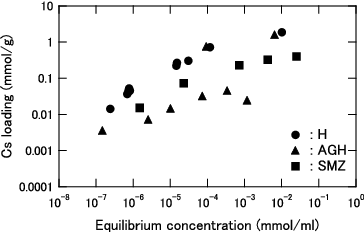
Figure 4. Cs loading as function of equilibrium Cs concentration for KURION herschelite in seawater (3.4 wt% salt).
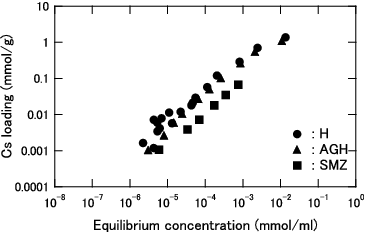
The distribution coefficient (Kd) is calculated from the Cs loading divided by the equilibrium Cs concentration, as shown in Equation (2). The obtained Kd values are shown in and for pure water and seawater, respectively.
(2)
Figure 5. Distribution coefficient (Kd) for Cs as function of equilibrium Cs concentration for KURION herschelite in pure water.
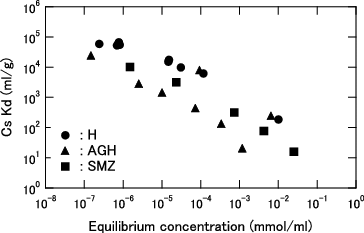
Figure 6. Distribution coefficient (Kd) for Cs as function of equilibrium Cs concentration for KURION herschelite in seawater (3.4 wt% salt).
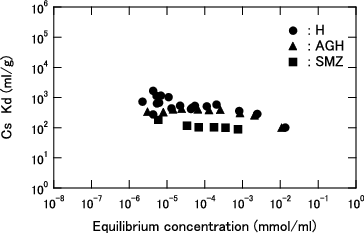
At an equilibrium concentration lower than 1.0 × 10−3 mmol/ml, the Cs loading of each herschelite sample is higher in pure water than in seawater, and the difference further widens with decreasing equilibrium concentration. Although the Cs loadings are in the order of H > SMZ > AGH in pure water, H and AGH can load almost the same amount of Cs in the seawater. Additionally, the difference between H and SMZ was smaller in the seawater.
The KURION decontamination system was designed such that almost all Cs in the contaminated water is removed by H. The obtained Kd values of H in pure water were varied from 6.2×103 to 6.7×104 at each equilibrium concentration from 2.4×10−7 to 1.2×10−4 mmol/ml, as shown in . On the other hand, the reported Kd values of IE-96 zeolite are varied from 7.6×103 to 4.6×104 at each equilibrium concentration from 1.2×10−10 to 5.2×10−5 mmol/ml [Citation10]. IE-96 zeolite was adopted in Cs removal in the contaminated water treatment facility in TMI. Therefore, it was confirmed that KURION H herschelite has almost the same performance in pure water as IE-96 zeolite.
The fundamental performance of the process proposed by KURION had to be evaluated in Japan at an early stage in the facility design consideration. In particular, our major concern was determining the amount of zeolite necessary and the number of spent zeolite columns that would be generated. From the viewpoint of facility operation, the radiation dose from zeolite columns has to be evaluated in order to protect the operators.
As mentioned above, the Cs concentration in the contaminated water is estimated to be 1–2 ppm (7.5 × 10−6 to 1.5 × 10−5 mmol/ml). shows that 1 g of H herschelite can load 1.0 × 10−3 to 1.0 × 10−2 mmol of Cs in equilibrium with the contaminated water. Herschelite near the inlet of the solution in the column is always in contact with the contaminated water containing sea salt. Thus, the maximum total Cs loading per one column is estimated from above the Cs loading per 1 g of zeolite and the amount of the zeolite in the column. Using the evaluated maximum Cs loading, tentative estimations of the amount of herschelite necessary or the number of generated spent columns were made.
To confirm the safety aspect of the KURION system, we also evaluated the total radiation dose or heat generation rate by a simplified method when the Cs concentration in the column is equal to the maximal Cs loading.
4.2. Effect of sea salt contents on adsorption
The Cs loadings of H herschelite in the solutions with various sea salt contents are shown in . The data in pure water (0.0 wt%) and seawater (3.4 wt%) are same as those of H herschelite in and , respectively. The sea salt dissolved in the solution has a negative effect on the Cs loading if the concentration of the sea salt is relatively high. Since there is little difference between the Cs loadings in the pure water (0.0 wt%) and seawater (0.034 wt%), the effects of sea salt can be ignored if the seawater is diluted 100-fold.
Figure 7. Cs loading as function of equilibrium Cs concentration for H herschelite in several sea salt containing solution. (Dashed lines: Results of calculation using Langmuir equation for each sea salt content).
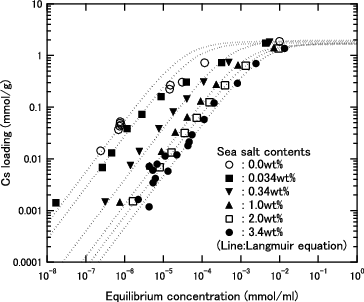
The ion exchange capacity, which corresponds to the maximum loading in , is estimated to be about 2.0 mmol/g for H herschelite at an equilibrium Cs concentration higher than 1.0 × 10−2 mmol/ml, regardless of the sea salt content. Compared with the Na concentration of 0.47 mmol/ml in the 3.4 wt% sea salt solution, the Cs ion exchange capacity of H herschelite is not affected by Na ions of a 50 times higher concentration than the Cs ions.
The ion exchange capacity of H herschelite is almost the same as that of IE-96, which is 2.0–2.5 meq/g (in the Cs case, meq = mmol) in the commercial catalog [Citation11].
From , it was assumed that the sea salt content would have the same effect on the Cs loadings of AGH and H herschelite. As for SMZ, the adsorption performance in the seawater was not examined in detail, because SMZ was replaced with Si sand immediately after the start of the full-scale operation [Citation12].
4.3. Effect of oil content on adsorption behavior
The effects of the amount of oil dissolved in the solution on the Cs loading of H herschelite are shown in with comparison of the data obtained for the normal pure water and seawater. At a low Cs concentration in pure water, the oil content in the solution affects the Cs loading to some degree. However, it was concluded that the oil content has little impact on the Cs adsorption behavior of H herschelite both in pure water and seawater.
The amounts of oil dissolved in pure water and seawater were measured by the Japanese Industrial Standards (JIS) method, in which the oil in the solution is extracted into carbon tetrachloride and the amount of extracted oil is analyzed. The amount of oil in carbon tetrachloride was measured by infrared absorption spectroscopy (Thermo Fisher Scientific, Nicolet iS FT-IR).
Regardless of the volume ratio of the aqueous solution to the oil phase, 10–200 ppm turbine oil was found to dissolve in pure water and seawater after a 3-day contact with turbine oil, and a similar amount of oil is expected to dissolve in the actual contaminated water. Therefore, if suspended oil particles are removed using filtration or an SMZ (or silica sand) column, the remaining oil in the contaminated water has little effect on the Cs adsorption performance on H herschelite.
4.4. Cs adsorption properties of KH
KH is prepared by precipitating KCCF within pores or on surface of H herschelite in order to enhance its Cs adsorption [Citation8]. The results of Cs loading for KH in the solutions with various sea salt contents under batch type adsorption conditions are shown in . At an equilibrium concentration lower than 1.0 × 10−5 mmol/ml, more Cs can be loaded on KH than on H herschelite, which is shown in .
5. Discussion
5.1. Adsorption isotherm equation for simulation code
Adsorption isotherm equations are essential in developing a simulation code for the zeolite column, which has been developed aiming at evaluating the performance and predicting the operating conditions [Citation13]. Several standard isotherm equations are already proposed for the zeolite column applied to the radioactive solution treatment [Citation14]. We tried to make the adsorption isotherm equation, which can be applied to a zeolite column system designed by KURION using the present adsorption data.
The contaminated water accumulated at Fukushima Daiichi NPS, initially contained sea salt at a relatively high concentration, but this concentration decreased gradually with the injection of pure water into the reactor. In the simulation code, the change in the sea salt concentration in the contaminated water must be monitored, and the effect of sea salt on the adsorption behavior must also be evaluated appropriately.
Among well-known isotherm equations, the following Langmuir-type equation shown as Equation (3) was adopted, because the flat shape of the adsorption isotherm at higher concentrations can be expressed easily:
(3) where Q is the amount of Cs absorbed, a1 is the a coefficient, b1 is the b coefficient and Cl is the concentration of Cs in solution.
Using the data-sets of the Cs loading for different sea salt concentrations shown in , individual Langmuir equations for H herschelite were adopted for the solutions containing 0.0 (pure water), 0.034, 0.34, 1.0, 2.0 and 3.4 wt% of sea salt. The a1 and b1 coefficients of the Langmuir equation for each sea salt concentration are estimated by regression analysis, and the estimated values are listed in . The adsorption isotherms estimated using the Langmuir equations are shown in and compared with the adsorption data. At arbitrary sea salt concentrations between 0.0 and 3.4 wt%, the coefficients a1 and b1 in the Langmuir equation are calculated by linear interpolation [Citation13].
Table 1. Langmuir coefficients for H herschelite at various sea salt concentrations.
5.2. Isotherm equation model of KH
At an equilibrium Cs concentration lower than 1.0×10−5 mmol/ml, KH can load more Cs than H herschelite, as described in Section 4.4, and the amounts of Cs loading by H and KH are almost same at an equilibrium Cs concentration of more than 1.0×10−4 mmol/ml.
It is assumed that Cs ions may be exchanged at two different kinds of ion-exchange site: the KCCF site and the original site of H herschelite. It is also assumed that the ion exchange at the KCCF site may be predominant at lower equilibrium Cs concentration and that this site is saturated at an equilibrium Cs concentration of more than 1.0×10−5 mmol/ml.
On this basis, the following two-site Langmuir equation model is used for KH:
(4)
The Langmuir coefficients a1 and b1 are assumed to be the same as those in Equation (3) derived from the H herschelite data. The coefficients a2 and b2 are estimated using only the KH data obtained at an equilibrium Cs concentration lower than 1.0 × 10−5 mmol/ml. However, owing to the limit of Cs detection by the atomic absorption spectrometer, only two or three data-sets were obtained at an equilibrium Cs concentration below 1.0 × 10−5 mmol/ml. Here, it is assumed that the saturated Cs loading at the KCCF site is 0.02 mmol/g at an equilibrium Cs concentration above 1.0 × 10−5 mmol/ml. With this assumption, the relation between a2 and b2 can be fixed, because the saturated Cs loading corresponds to a2/b2 = 0.02 in the Langmuir equation. Moreover, one more data-set, that is, the Cs loading of 0.02 mmol/g at an equilibrium Cs concentration of 1.0×10−5 mmol/ml, can be introduced when the Langmuir equation coefficient a2 for each sea salt concentration is estimated by regression analysis.
The estimated a2 and b2 values for each sea salt concentration are listed in . The adsorption isotherms estimated using the first term of the two-site Langmuir equation with only a1 and b1 and using the second term with only a2 and b2 are shown in (a)–(d), respectively, for each sea salt content. The summation of all the Cs loadings obtained using the first and second terms of the two-site Langmuir equation shows good agreement with the obtained data for KH.
Table 2. Langmuir coefficients of KCCF site in two-site Langmuir equation for KH at each sea salt concentration.
Figure 10. Comparison between Cs loading data and adsorption isotherms estimated using two-site Langmuir equation for KH.
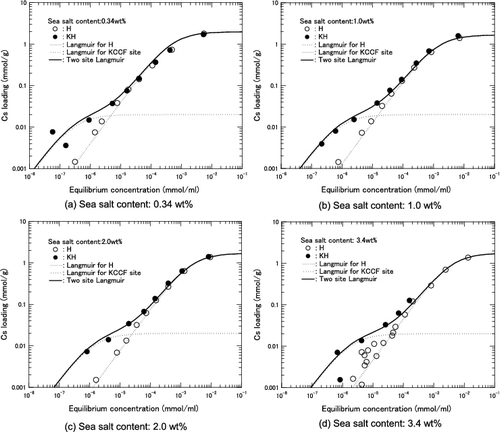
Therefore, it can be concluded that the two-site Langmuir equation model is applicable to the estimation of the Cs adsorption behavior of KH.
6. Conclusions
KURION herschelite-type zeolite was adopted for Cs removal in the radioactive waste water treatment facility at Fukushima Daiichi NPS. The adsorption properties of various kinds of KURION herschelite were examined in Japan and used as basic data for the design discussion. Since the contaminated water contained seawater and oils such as turbine oil, the effects of sea salt and dissolved oil content on Cs adsorption behavior were examined closely.
The Cs loading of H herschelite in the seawater was found to decrease to nearly 1/10th of that in pure water. It was, however, concluded that KURION herschelite has sufficient adsorption capacity to remove Cs from the contaminated water by the proposed process. The effect of dissolved oil could be ignored, because very little amount of oil remains in the seawater. To develop a simulation code that can analyze the adsorption behavior or predict the operation conditions of the actual decontamination system, necessary parameters and relational expression were also evaluated. Thus, Langmuir equations that can be applied to the measurement of Cs adsorption isotherms in the sea salt containing water were developed for H herschelite. Additionally, it was found that a two-site Langmuir equation can be applied to the analysis of KCCF-added KH, because KCCF functions as another ion-exchange site in addition to the normal site of H herschelite.
Acknowledgements
The authors express their many thanks to Mr S. Sakuno, Mr R. Sagawa and Mr N. Yahagi for their support in the analysis.
References
- Sanchez HF, Quinn GJ. Submerged demineralizer system processing of TMI-2 accident waste water. Idaho Falls (ID): EG&G Idaho Inc.; 1983. (Report no. GEND-031).
- Groove Engineering Inc. The cleanup of three miles island unit 2 – a technical history: 1970 to 1990. Rockville (MD): Groove Engineering Inc.; 1990. ( Final report NP-6931, project 2558 8 EPRI).
- Langton CA, Cook JR. Recent progress in DOE waste tank closure. Paper presented at: WM Symposium 2008; 2008 February 24–28; Phoenix, AZ. WSRC-STI-2007-00686.
- Website of TEPCO. Progress status of “roadmap towards restoration from the accident at Fukushima Daiichi Nuclear Power Station” on September 20, Appendix 2 “roadmap towards restoration from the accident at Fukushima Daiichi Nuclear Power Station, TEPCO”. Press release. 2011 Sep 20. p. 8.
- Uozumi K, Iizuka M, Kinoshita K, Tsukada T, Koyama T. Development of salt and metal waste treatment technology for pyroprocess in CRIEPI (paper no. 387026). Proceedings of GLOBAL 2011; 2011 Dec 11–16; Makuhari, Chiba.
- Tokyo Electric Power Company: Overview of decontamination instruments (adsorption tower)[Internet]. Tokyo: 2011 June 17. Available from: http://www.tepco.co.jp/en/ nu/fukushima-np/images/handouts_110617_04-e.pdf
- Tokyo Electric Power Company: Cesium decontamina- tion instrument diagram (Valve Status in H4 skid)[Internet]. Tokyo: 2011 June 23. Available from: http://www.tepco.co.jp/en/nu/fukushima-np/images/handouts__110623_01-e.pdf
- Denton MS, Bostick WD. Development and testing of two novel and vitrification compatible classes of ion specific media for application on the hanford waste treatment plant supplemental pretreatment and secondary waste streams – 11001. WM 2011 Conference; 2011 Feb 27–Mar 3; Phoenix, AZ.
- Website of Tokyo Electric Power Company. http://www. tepco.co.jp/nu/fukushima-np/images/handouts_110425_ 02-j.pdf. Japanese.
- Brown GN, Carson KJ, DesChane JR, Elovich RJ. Performance evaluation of 24 ion exchange materials for removing cesium and strontium from actual and simulated N-reactor storage basin water. Richland (WA): Pacific Northwest National Laboratory; 1977. (PNNL-11711,UC-2030).
- Product Information of INOVS IE-96 Ion Exchanger. UPO commercial catalog UOP Molecular Sieve Sales Office (2/24/97).
- Website of the Nuclear and Industrial Safety Agency at the Ministry of Economy. Available from: http://www. meti.go.jp/earthquake/nuclear/pdf/20110816nisa2.pdf. Japanese.
- Inagaki K, Hijikata T, Tsukada T, Koyama T, Ishikawa K, Ono S, Suzuki S. Early construction and operation of the highly contaminated water treatment system in Fukushima Daiichi nuclear power station (III) – A unique simulation code to evaluate time-dependent Cs adsorption/desorption behavior in column system. J Nucl Sci Technol. 2014 (this issue).
- Robinson SM, Kent TE, Arnold WD, Parrott JR Jr. The development of a zeolite system for upgrade of the process waste treatment plant. Oak Ridge (TN): Oak Ridge National Laboratory; 1993. (ORNL·M-12063).