Abstract
For the better understanding of chemical behavior of Sr-90 released from the damaged reactor cores of Fukushima Daiichi nuclear power station into the seawater system, the dissolution behavior of Sr(II) to the mixed seawater was experimentally investigated by the solubility measurement of Sr(II) under several solution conditions (mixing degree of seawater, pH, strontium concentration and temperature) and the microscopic analysis of the formed solid phase. The obtained results of the experiment were compared with thermodynamic equilibrium calculations for an interpretation of chemistry of Sr(II) in the targeted solution system. It was revealed that the Sr solubility became higher than the reported value in generic seawater system, while the solubility limiting solid was identified as SrSO4 which corresponded with the result of equilibrium calculation. Furthermore, under the unsaturated condition, about 6% of Sr(II) interacted with natural nano-size colloids existing in the Fukushima seawater and was captured by 3 kDa filter.
1. Introduction
On 11 March 2011, the loss of coolant accident occurred at Fukushima Daiichi nuclear power station (NPS) operated by Tokyo Electric Power Company (TEPCO), due to the mega earthquake and the following big tsunami struck. After the NPS black out, over 4000 tons of seawater was injected into the high-temperature reactor cores for the emergency cooling. The water-soluble radionuclides, such as Cs-137/134 and Sr-90, were released into the cooling water from the damaged reactor cores. Consequently, this emergency core cooling activity generates huge amount of contaminated water at the NPS. The main components of this contaminated water are currently reported as Cs-137/134, Sr-90 and H-3 [Citation1]. From the beginning of this core cooling action, radioactive Cs was recognized as the main contributor for the extremely high radiation dose from the contaminated water; therefore, Cs removal systems, i.e., AREBA-coprecipitation system, KURION and SARRY-zeolite absorption systems, were installed on the site in short order. However, these systems do not work for the removal of Sr-90 from the contaminated water; as a consequence, Sr-90 is accumulated in the treated-contaminated water stored in the temporally storage tanks on the NPS site. Since these tanks were constructed as the “temporally storage” in the emergency situation, the durability and robustness of them were insufficient to store such highly radioactive water for long time period. Consequently, several times of unexpected leaking of contaminated water which contained Sr-90, H-3 and other radioactivities occurred from the storage tanks at the NPS. To decrease the risk of re-spread of Sr-90 to the environment from the contaminated water, TEPCO has constructed new water decontamination plant named ALPS (advanced liquid processing system) which was designed to remove 62 radionuclides including Sr-90 [Citation2]. However, this new plant ALPS currently does not work perfectly due to technical problems (e.g., TEPCO's press releases on 18 and 19 March 2014). It is commonly recognized that Sr-90 is one of the most serious radioactive contaminants on the NPS site and surrounding environment including forest and sea, due to its high solubility to the water and high affinity to human body. Therefore, the behavior of Sr-90 on the NPS site and the surrounding area is a great concern for the people involved in the post-accident activities. An important factor which makes the prediction of Sr-90 behavior relatively difficult is the mixing of seawater into the reactor cooling system and into the groundwater around the NPS site located on the coast area. It is completely different situation from the accident at Three Mile Island, PA, USA, occurred in 1979, where no seawater was used for emergency core cooling and groundwater at that place contained no seawater. Since seawater contains high amount of alkaline earth metals, i.e., about 9 × 10−5 M of “stable” Sr, 0.01M of Ca and 0.05 M of Mg [Citation3], we have to take them into account to predict the chemical behavior of radioactive Sr-90 on the Fukushima NPS site and the around area. Due to the mixing of the seawater in the contaminated water, one has to remove huge amount of stable Sr and very few physical amount of Sr-90 simultaneously for the decontamination process of the contaminated water, which have caused difficulties for the operation of the new plant ALPS and in the countermeasure activities for the leaked Sr-90. In addition, variety of components in seawater, i.e., alkaline and alkaline earth metals, anions like chloride, sulfate, phosphate, carbonate and organic/inorganic natural colloids/particles may interact with Sr-90 and change its chemical species from free cation to complexes or pseudo-colloids. Consequently, these phenomena are expected to prevent Sr-90 interaction with the absorbent installed in the decontamination system and give negative impact on the decontamination activity conducted on the NPS site. Therefore, to re-construct an effective decontamination strategy for Sr-90, it is very important to make better scientific understanding of chemical speciation of Sr-90 in the “real” environment of the contaminate water on the Fukushima NPS site.
In the field of environmental chemistry, several researches have been conducted to understand the chemical behavior of Sr on the terrestrial environment. In the marine environment, the dominant solid phase of Sr are commonly recognized as celesite (SrSO4) presenting as the skeletal material of Acantharia [Citation4,5] and as the major impurity of barite (BaSO4) crystals in marine sediments [Citation6]; therefore, the solubility of celesite has been experimentally determined in seawater or brine conditions [Citation4,Citation7–10]. The incorporation phenomenon of Sr2+ into calcite (CaCO3) had been also understood to play an important role for the chemistry of Sr in the marine environment, and it was thereby enthusiastically investigated [Citation11–13]. Besides, it is recognized that carbonate and variety of colloids and/or suspended particles, such as silicate, organic and organo-mineral colloids, could control the speciation and migration of Sr-90 in freshwater system like river or lake [e.g., Citation14–16]. This knowledge obtained in the preceding studies can be and should be utilized for understanding of the current chemical state of radioactive Sr in the Fukushima NPS site. Nevertheless, the condition and the composition of the contaminated water in the NPS site is really complicated and different from generic seawater or freshwater environment due to the mixing of the seawater and the thermal gradient caused by the reactor cores. The purpose of this study, therefore, is predicting chemical state and speciation of radioactive Sr in the contaminated water condition of the Fukushima NPS by an experimental approach. The solubility measurements of Sr were conducted under the condition of undiluted and diluted seawater at ambient (25 °C) and high (90 °C) temperatures, where real Fukushima seawater and artificial seawater were used. To investigate the solubility limiting solid of Sr, the formed solid phase was analyzed by X-ray powder diffraction (XRD) and scanning electron microscope-energy dispersive X-ray (SEM-EDX). The interaction of radioactive Sr and natural colloids existing in the Fukushima seawater was also experimentally investigated. The obtained results of experiment have been interpreted based on the knowledge of thermodynamics and the preceding studies.
2. Experimental
2.1. Reagents
Non-radioactive SrCl2·6H2O (Wako Pure Chem. Ltd.) and radioactive 85Sr were used for two kinds of apparent solubility measurement as described in the following. Radioactive tracer 85Sr was purchased from PerkinElmer (MA, USA) as HCl solution having the specific activity more than 111 GBq/g. Natural surface seawater was collected at Urajiri coast in Odaka-ku, Minamisoma city, Fukushima, distanced 11 km north from Fukushima Daiichi NPS and supplied for the following experiments. The seawater was corrected using a hand pump from the seashore and kept in a cool dark place. Artificial seawater was prepared by dissolving Daigo's Artificial Seawater SP (Nihon Pharmaceutical) with ultrapure Milli-Q water. To control and keep the pH values, the fresh NaOH and HClO4 (Wako Pure Chem. Ltd.) stock solutions were prepared, and the pH buffer reagents of TAPS and CHES (Dojindo Molecular Technologies, Inc.) were also used. All chemicals used were reagent grade.
2.2. Solubility measurement
A sample solution was prepared to measure the apparent solubility of strontium ([Sr]app) and to form its precipitate as the solubility limiting solid. Non-radioactive strontium was mixed with 500 mL of the supernatant of the Fukushima seawater without pre-filtration, the artificial seawater, or 0.5 mol/dm3 (M) NaCl solution in a polypropylene vessel. The seawater diluted 2 and 10 times by ultrapure Milli-Q water was also used as the diluted seawater samples. The pH was adjusted to neutral by adding small amount of NaOH or HClO4 solutions, using combination glass electrodes (9611-10D Horiba Ltd.). The internal solution of the electrode was replaced with 3.6 M NaCl + 0.4 M NaClO4 to avoid precipitation of KClO4 at the junction of the electrode and the solution. Neutral-pH buffer reagents, i.e., TAPS and CHES, were added to keep the equilibrium pH ranges of 8–9 and 9–9.5, respectively, and the buffer concentration was set to 10−3 M. After 30 days aging, the pH values of the supernatants of the sample solutions at 25 and 90 °C under atmospheric conditions were measured. The supernatants were filtered through a Dismic 0.45 μm filter (Advantec, Toyo Roshi) and Amicon Ultra-0.5 centrifugal filter (EMD Millipore) with a nominal molecular weight limit of 10 kDa (approximately 3 nm pore sizes). Aliquots of the filtrate were acidified and diluted with 0.1 M nitric acid for the determination of the Sr concentration by inductively coupled plasma-mass spectroscopy (ICP-MS; ELAN DRC2, PerkinElmer) with a detection limit of approximately 10−10 M. Meanwhile, the precipitate in the bottom of sample tube was collected and dried in a vacuum desiccator for the solid-phase analysis described later.
2.3. Recovery investigation of tracer Sr-85
The recovery test of tracer amount of Sr-85 (added to be ca. 7 × 10−11 M corresponding to 200 kBq) from the Fukushima seawater sample was also conducted. Each 40-mL sample in 50-mL polypropylene tube was kept at the neutral pH without any control at 25 and 90 °C. Since the Fukushima seawater contains a trace but variety of dissolved solids as suspension and/or colloidal forms, the seawater pre-filtrated by 0.45-μm filter was also used as a reference test. Same three batches were prepared to check the experimental reproducibility. After 7 and 30 days aging periods, the pH values of the supernatants were measured. The supernatants were filtered through 0.45 μm, 0.2 μm, 100 kDa, 10 kDa and 3 kDa membranes, followed by measuring the gamma-ray activity of Sr-85 (514 keV) to evaluate the recovery ratio to the initial input of Sr-85 tracer.
2.4. Solid-phase analysis
2.4.1. Analysis of the solubility limiting solid
The vacuum-dried precipitate samples obtained by the solubility measurement experiments described in Section 2.2 were analyzed by the following techniques to know the composition and structure of the solubility limiting solids of strontium. For the phase analysis of the precipitate, XRD was conducted by Ultima IV (Rigaku) diffractometer using monochromatized Cu-Kα radiation (40 kV, 40 mA). The measurement was performed 2θ = 20o–60o by continuous scanning with a scan rate of 2o min−1 and an interval of 0.02o. The measured XRD pattern of the precipitate was compared with that in Inorganic Crystal Structure Database (ICSD) to identify the phase and crystal structure. The morphology and elemental composition of the precipitate was observed by using a scanning electron microscope (SEM, SU1510, Hitachi) in secondary electron mode with an energy dispersive X-ray analyzer (EDX, EMAX x-act, Horiba Ltd.). For the SEM-EDX observation, the dried precipitate sample was put on an aluminum stage using carbon double face tape, then it was coated by Au–Pd to increase conductivity of the sample by using a magnetron sputter (MSP-1S, Vacuum Device).
2.4.2. Analysis of colloid particles in the Fukushima seawater
Natural colloidal particles with molecular weight distributed from 3 kDa to 10 kDa were collected from the Fukushima seawater for the SEM-EDX observation. An amount of 200 mL of the seawater was pre-filtered by a 0.2-μm pore size membrane filter, and then the filtrate solution was provided for the pressurized (0.1 MPa) ultra-filtration using a 10-kDa filter (Ultracel, 10 kDa, Millipore). This filtrate solution was again provided for the second pressurized (0.2 MPa) ultra-filtration using a 3 kDa filter (Ultracel, 3 kDa, Millipore) to collect colloidal particles having a molecular weight cut-off of 3 kDa to 10 kDa. Then, 20 mL of distilled water was passed through the used 3 kDa filter to rinse the remaining seawater and salts out of the collected colloidal particles on the filter. After that, the center part of the 3 kDa filter with the colloidal particles was cut into 1-cm square and put on an aluminum SEM stage using carbon double-face tape. This filter on the SEM stage was then freeze dried by a freeze dryer (FDU-2200, EYELA). Finally, the colloidal particles on the filter were coated by Au–Pd using the magnetron sputter for the SEM-EDX analysis.
3. Result and discussion
3.1. Solubility of Sr(II) in aqueous system mixed with seawater
3.1.1. Solubility measurement of bulk quantity Sr
Apparent solubility of strontium at 25 °C in the Fukushima seawater and the artificial seawater obtained by filtration with 0.45 μm filter is shown in (a) and (b), respectively.
Figure 1. Strontium solubility (a) in the Fukushima seawater (SW) and (b) in the artificial seawater (AW) at 25 °C after 0.45-μm filtration. pH value in legends is the rounded figure.
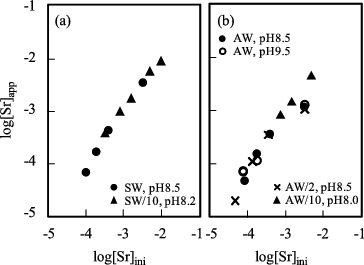
With increasing the initial concentration of Sr (= [Sr]ini), the apparent solubility increased linearly in both cases. The added strontium could be dissolved up to about 10−2 M, while a matrix Sr in the natural and artificial seawaters has been reported as ca. 9 × 10−5 and 8 × 10−5 M, respectively [Citation3,Citation17]. The reason of the observed higher solubility than generic seawater system is supposed to be the short aging time of the solubility limiting solid phases; nevertheless, the apparent solubility obtained by the experiment in 30 days aging is very informative for understanding of chemical state of radioactive Sr in the contaminated water at the initial stage of the NPS accident. Small amount of white precipitate was obtained from the batch of 10 times diluted Fukushima seawater sample at the initial Sr concentration 10−2 M. The SEM image of this precipitate is shown in (a).
Figure 2. SEM images of the precipitates from the Sr solubility measurement experiment. (a) 10 times diluted Fukushima seawater sample at pH 8.1, T = 25 °C, [Sr2+]int. = 10−2 M, (b) artificial seawater sample (no dilution) at pH 8.6, T = 25 °C, [Sr2+]int. = 10−2.5 M and (c) artificial seawater sample (no dilution) at pH 9.4, T = 90 °C, [Sr2+]int. = 10−2.5 M.
![Figure 2. SEM images of the precipitates from the Sr solubility measurement experiment. (a) 10 times diluted Fukushima seawater sample at pH 8.1, T = 25 °C, [Sr2+]int. = 10−2 M, (b) artificial seawater sample (no dilution) at pH 8.6, T = 25 °C, [Sr2+]int. = 10−2.5 M and (c) artificial seawater sample (no dilution) at pH 9.4, T = 90 °C, [Sr2+]int. = 10−2.5 M.](/cms/asset/4e84c56e-545d-40f2-a4a6-6e7e7ae7b5b2/tnst_a_935510_f0002_b.gif)
The EDX spectra of this precipitate showed the existence of only three elements, i.e., Sr, S and O with the atomic abundance ratio Sr:S:O = 1:0.9:9.7, which predicts that the precipitate was SrSO4•xH2O. More amount of precipitates were obtained from the batch of the no-diluted artificial seawater samples at pH 8.6 having the initial Sr concentration 10−2.5 M. This precipitate was devoted to the SEM-EDX and XRD analyses. The SEM image of this precipitate is shown in (b), where Sr, S and O were detected with the atomic abundance ratio Sr:S:O = 1:1.1:3.7 by the EDX analysis, clearly indicating the composition of SrSO4. In (a), the XRD pattern of the precipitate shown in (b) is compared with the standard XRD patterns of SrSO4, CaCO3 and SrCO3 listed in ICSD.
Figure 3. XRD patterns of the precipitates obtained by the solubility measurement of Sr(II). (a) Precipitate from the artificial seawater sample at 25 °C, pH 8.6 (image is shown in Figure 2(b)), (b) from the artificial seawater sample at 90 °C, pH 8.5, (c) from the artificial seawater sample at 90 °C, pH 9.4 (image is shown in Figure 2(c)), (d) from the two times diluted artificial seawater sample at 90 °C, pH 8.7 and (e) from the Fukushima seawater sample at 90 °C, pH 8.5.
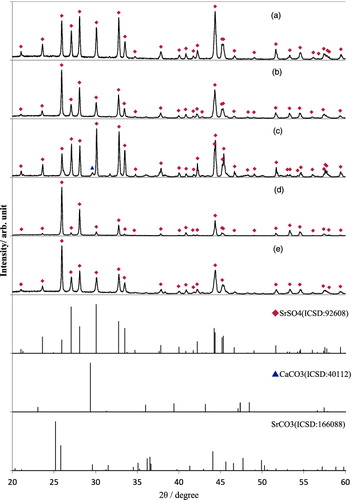
It is obvious that the precipitate obtained from the batch of the artificial seawater at pH 8.6 (shown as (b) and (a)) is simple SrSO4 phase, which agrees well with the prediction by the EDX analysis.
shows the strontium solubility at 90 °C in (a) Fukushima seawater and (b) artificial seawater. This temperature might have been arisen at the contaminated water in/around the reactor cores.
Figure 4. Strontium solubility (a) in the Fukushima seawater and (b) in the artificial seawater at 90 °C after 0.45-μm filtration. pH value in legends is the rounded figure.
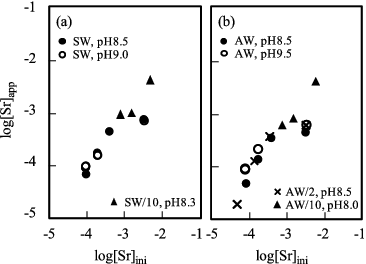
In comparison with at room temperature, the solubility at the higher initial concentration decreased in both of the Fukushima and the artificial seawater systems, which indicates more Sr solid phases were formed at 90 °C. This result is interpreted as the formation of SrSO4 phase was promoted at the increased temperature, and consequently, the apparent solubility of Sr at 90 °C became lower than that at 25 °C. From the batches of 90 °C experiments, certain amount of white precipitate was formed at the bottom of the sample tube and collected for phase analysis. The similar rectangular shape of morphology to that obtained at 25 °C experiment was observed at 90 °C, and EDX spectra indicated the composition of SrSO4. The XRD patterns of the precipitates obtained at 90 °C, from the artificial seawater sample at pH 8.5, from the two-times diluted artificial seawater sample at pH 8.7 and from the Fukushima seawater sample at pH 8.5, are shown in (b), 3(d) and 3(e), respectively, where no peaks other than SrSO4 was identified. Meanwhile, Sr, S and O plus small amount of Ca were detected with the atomic abundance ratio Sr:S:O:Ca = 1:1.1:7.0:0.1 by the EDX analysis from the precipitates obtained at 90 °C and pH 9.4. The SEM image of this precipitate is shown in (c). Due to the progress of the deprotonation of hydrogencarbonate ion, more CaCO3 should be formed at pH 9.4 seawater than at pH 8.5. Therefore, from the morphology shown in (c) and the elemental composition, the bigger rectangular particles in the picture were considered to be SrSO4, while the smaller spherical particle was supposed to be CaCO3. The XRD pattern of this precipitate at pH9.4 is shown in (c). The comparison of this XRD pattern with the references clearly indicates this precipitate is a mixture of SrSO4 and CaCO3, which supports the above-mentioned hypothesis derived by SEM-EDX observation. It should be noted here that although the XRD peak positions shown in (a)–(e) correspond with that of standard SrSO4 pattern except a peak of CaCO3 in (c), the relative intensities of SrSO4 peaks are different from each other. This indicates that different SrSO4 phases were formed depending on the solution condition such as seawater concentration, temperature and pH. From these results, it is concluded that the solubility of Sr is controlled by the formation of SrSO4 solid in the undiluted or diluted seawater system (including both the Fukusima and the artificial seawater) in the temperature range of 25–90 °C.
Our result indicates that the supply of sulfate anion from seawater takes very important role for the chemical behavior of Sr(II) in the studied system. Additionally, to get confirmation on our conclusion, no-sulfate anion system, i.e., 0.5 M NaCl water system, was also applied for the Sr solubility experiment. The added strontium was completely dissolved in the 0.5 M NaCl system even in the highest initial Sr concentration ([Sr2+]int. = 10−2.3 M), without forming any precipitate. No influence of the initial Sr concentration and of the aging temperature on the solubility was observed. This supports our conclusion about the solubility limiting solid. Furthermore, the solubility after 10-kDa filtration was same as that after 0.45-μm filtration in all experimental batches, indicating that a precipitation solid distributing in between 0.45 μm and 3 nm (corresponding to 10 kDa), and/or a sorption of strontium ion on existing suspended matter, is negligible.
3.1.2. Recovery of tracer Sr-85 from seawater
In the previous chapter, it was revealed that the solubility of Sr(II) is controlled by SrSO4 solid phase; in other words, all Sr(II) can thermodynamically exist as soluble species as long as the total concentration of Sr(II) is less than the solubility limit of SrSO4 solid phase. However, it is well known that trace amount of Sr can be sorbed on natural colloids and behave as pseudo-colloid in the real environment [Citation14–16]. There is a possibility that this pseudo-colloid formation occur for the radioactive Sr in the Fukushima NPS, and it might prevent function of the radioactivity decontamination system for the contaminated water. Therefore, the recovery test of Sr-85 tracer from the seawater was conducted to check the affection of the colloids, where the doped Sr tracer concentration was extremely low (ca. 5 × 10−11 M corresponding to 250 kBq) so that SrSO4 was not formed in the batch experiment. shows the size distribution of the abundance of Sr-85.
Figure 5. Dependence of filtration after aging 30 days at 25 (a) and 90 °C (b) on the recovery ratio of strontium-85 from the Fukushima seawater. The seawater was non-filtrated or filtrated by 0.45-μm filter prior to adding Sr-85.
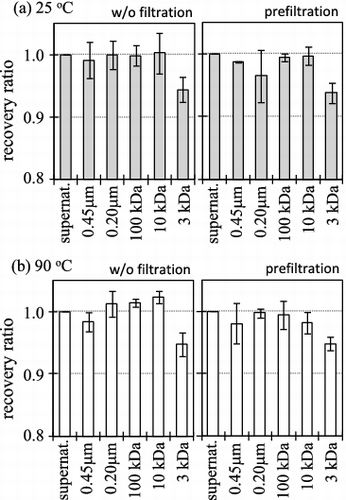
No significant change of the recovery ratios was observed between 7 and 30 days, so that the equilibrium steady state was considered to be achieved after at least 7 days. The ratios normalized to the initial input of Sr-85 tracer after aging for 30 days at 25 and 90 °C were found to be 1 for the supernatant and the filtrated solutions with 0.45 μm, 0.2 μm, 100 kDa and 10 kDa membranes. However, the 3-kDa filtration decreased the recovery ratio by about 6%, and this was constant with the aging temperature and the pre-filtration of seawater by 0.45-μm membrane. This implies the pseudo-colloid formation with a component of seawater. The captured fraction of Sr-85 by 3 kDa was about 6% in our experiment, which is quite small in comparison with reported colloidal fraction of radioactive Sr in freshwater system [Citation14–16]. This difference seems to be attributed to the high salinity of the seawater system since the surface negative charges on the colloids tend to be neutralized by the dominant cations in seawater; thus, the interaction between radioactive Sr and the colloids is suppressed. Accordingly, the natural colloids or suspended particles distributing in between 10 and 3 kDa sizes were collected from the Fukushima seawater on a 3-kDa filter by the procedure described in Section 2.3.2, and they were analyzed by the SEM-EDX. The observed SEM images of collected particles with elemental composition calculated from EDX spectra are shown in . It should be noted that the collected particles on the 3 kDa were originally smaller than 10 kDa since the sample seawater was pre-filtrated by 10 kDa filter before the collection, and then the collected particles were considered to have aggregated to bigger size than 1 μm during the freeze dry process. By the observation of these aggregate particles, we can derive information about originally existed colloids distributing in between 10 and 3 kDa.
The particle shown in (a) seems like an aluminosilicate from the elemental composition, while that shown in (b) can be regarded as silica colloid due to the low atomic composition of Al. On the contrary, the composition of a particle shown in (c) is somewhat complex. Since the major elements of this are Si and Al except for C and O, the particle is thought to be an aluminosilicate with Fe and sea salts. Due to the high atomic percentage of carbon, there is a possibility that this particle may include organic matters; however, it cannot be concluded here since the 3-kDa filter paper itself is made by carbon. From the result of the filter observation, a possibility is pointed out here, i.e., a part of Sr-85 has been sorbed on a few nano-meter size (< 10 kDa) silica colloids and/or clay colloids existing in the Fukushima seawater, and those pseudo-colloids containing Sr-85 were captured by 3-kDa filter. On the Fukushima NPS, it is reported by the TEPCO's press releases on 18 and 19 March 2014 that the designed performance for Sr-90 removal by ALPS has not been achieved. The formation of the pseudo-colloids containing radioactive Sr, which was observed in this study, might be a part of the reasons of this disorder of ALPS operation.
3.2. Comparison of experimental results with thermodynamic calculation
For comparison with our experimental results, thermodynamic calculations of Sr speciation in the mixed seawater systems were done by using equilibrium stability constants listed in a database HYDRA [Citation18] and a calculation software MEDUSA [Citation19], where the generic seawater composition listed in the data table [Citation3] were inputted. The calculated Sr fraction diagrams for generic seawater condition and 10-times diluted condition are shown in and , respectively. Since seawater contains high concentration of carbonate anion which behaves as pH buffer, the pH is hardly changed by the addition of Sr(II) in the system. Therefore, these calculations were conducted under the fixed pH condition. The given solution conditions including pH are shown in the top of and .
indicates that 100% of Sr(II) exists in the seawater as soluble species up to about 0.08 mM, and then SrSO4 solid is formed as solubility limiting solid at higher Sr(II) concentration. In addition, the dominant soluble Sr(II) species in the seawater are calculated as strontium sulfate “aquo” complex (SrSO4(aq), ca. 60%) and free strontium cation (Sr2+, ca. 40%). Meanwhile, in the 10-times diluted seawater system, Sr(II) solubility limit becomes three times higher, i.e., 0.25 mM as shown in , and the soluble species are 80% of free strontium cation and 20% of strontium sulfate “aquo” complex. tells us a notable phenomenon, i.e., when total Sr(II) concentration exceeds 1.0 mM, the solubility of Sr(II) cannot be limited by SrSO4 solid anymore due to the lack of SO42− anion in the system, and consequently, the solubility of Sr(II) re-increases because of higher solubility of SrCO3(c) than that of SrSO4(c).
The thermodynamic calculation reasonably explains our above-mentioned experimental results, i.e., the observed solid phases from the undiluted and 10-times diluted seawater samples were analyzed as SrSO4(c) corresponding to the calculation. Based on the obtained experimental facts and the thermodynamic consideration, it is predicted that the chemistry of Sr-90 released from the reactor cores at Fukushima NPS was controlled by the components of seawater, especially “stable” Sr cation and sulfate anion. Since Sr concentration in seawater is relatively high (ca. 0.09 mM [Citation3]), one has to consider about the solubility limitation by SrSO4 and capacity occupation by “stable” Sr(II) when Sr-90 removal is tried by using a chemical separation technique from the contaminated water of Fukushima NPS site. This chemical condition seems a serious technical problem for the decontamination operation of Sr-90 on the NPS site and is completely different from that for Cs-137/134 decontamination conducted there, since “stable” Cs concentration in Japanese coast seawater is reported to be 1.8–2.5 nM [Citation20] which is so low that “stable” Cs in seawater is expected not to occupy the sorbent's capacity in the decontamination systems. Due to a fear of Sr-90 spreading to the open sea, TEPCO attempted to make a plan for decontamination of Sr-90 from the retained water in the port area of the NPS site. It should have been noticed that the retained water is simply separated from the open sea by silt fences which allow most of ions including Sr(II) in seawater come and go. Therefore, if one tries to separate Sr-90 completely from that retained water by using an ion exchange or an absorption system, that system has to extract infinite amount of “stable” Sr(II) which is supplied from the open sea forever. It seems quite unfeasible. To make a rational strategy on the countermeasure for Sr-90 in the NPS, the chemical behavior of Sr including the above-mentioned matters in this study has to be taken into account for the process designing.
4. Conclusion
For the understanding of chemical state and speciation of radioactive Sr in the contaminated water of the Fukushima NPS, the solubility measurements of Sr in the mixed seawater system were conducted using the real Fukushima seawater. It was revealed that the Sr solubility became higher than the reported value in generic seawater system due to the short aging time of the solid phase, while the solubility limiting solid was identified as strontium sulfate corresponding to the preceding study on the marine environment. Furthermore, the recovery experiment of tracer Sr-85 from the seawater under the unsaturated condition revealed that about 6% of Sr(II) interacted with natural nano-size colloids and was captured by 3-kDa filter. This pseudo-colloid formation might impede the performance of Sr-90 decontamination system. The experimental fact and the thermodynamic calculation have indicated that the chemistry of Sr-90 released from the reactor cores at Fukushima NPS was controlled in large part by the components of seawater, especially “stable” Sr cation and sulfate anion, which should be considered in the planning of countermeasure for the Sr-90.
Acknowledgements
This work was supported by JSPSKAKENHI grant-in-aid for Scientific Research (S) [grant number 24226021].
References
- Secretariat of the Team for Countermeasures for Decommissioning and Contaminated Water Treatment. Progress status and future challenges of the mid-and long-term roadmap towards the decommissioning of TEPCO's Fukushima Daiichi nuclear power station units 1-4. Tokyo: Tokyo Electric Power Company, Inc.; February 28, 2014. Available from: http://www.tepco.co.jp/en/nu/fukushima-np/roadmap/conference-e.html
- Tokyo Electric Power Company, Inc. Status of the Fukushima Daiichi nuclear power station – with focus on countermeasures for contaminated water. Tokyo: Tokyo Electric Power Company, Inc.; January 27, 2014. Available from: http://www.tepco.co.jp/en/nu/fukushima-np/water/index-e.html
- National Astronomical Observatory of Japan. [Chronological science tables 2005]. Tokyo: Maruzen; 2004. Japanese.
- North NA. Pressure dependence of SrSO4 solubility. Geochim Cosmochim Acta. 1974;38:1075–1081.
- Bernstein RE, Betzer PR, Feely RA, Byrne RH, Lamb MF, Michaels AF. Acantharian fluxes and strontium to chlorinity ratios in the North Pacific Ocean. Science. 1987;18:1490–1494.
- Monnin C, Cividini D. The saturation state of the world's ocean with respect to (Ba,Sr)SO4 solid solutions. Geochim Cosmochim Acta. 2006;70:3290–3298.
- Culberson CH, Latham G, Bates RG. Solubilities and activity coefficients of calcium and strontium sulfates in synthetic seawater at 0.5 and 25 °C. J Phys Chem. 1978;82:2693–2699.
- Reardon EJ, Armstrong DK. Celestite (SrSO4(s)) solubility in water, seawater and NaCl solution. Geochim Cosmochim Acta. 1987;51:63–72.
- Monnin C, Galinier C. The solubility of celestite and barite in electrolyte solutions and natural waters at 25 °C: a thermodynamic study. Chem Geol. 1988;71:283–296.
- Rushdi AI, McManus J, Collier RW. Marine barite and celestite saturation in seawater. Mar Chem. 2000;69:19–31.
- Mucci A, Morse JW. The incorporation of Mg2+ and Sr2+ into calcite overgrowths: influences of growth rate and solution composition. Geochim Cosmochim Acta. 1983;47:217–233.
- Pingitore NE Jr, Eastman MP. The coprecipitation of Sr2+ with calcite at 25 °C and 1 atm. Geochim Cosmochim Acta. 1986;50:2195–2203.
- Morse JW, Bender ML. Partition coefficients in calcite: examination of factors influencing the validity of experimental results and their application to natural systems. Chem Geol. 1990;82:265–277.
- Ilina SM, Viers J, Lapitsky SA, Mialle S, Mavromatis V, Chmeleff J, Brunet P, Alekhin YV, Isnard H, Pokrovsky OS. Stable (Cu, Mg) and radiogenic (Sr, Nd) isotope fractionation in colloids of boreal organic-rich waters. Chem Geol. 2013;342:63–75.
- Jones MT, Pearce CR, Jeandel C, Gislason SR, Eiriksdottir ES, Mavromatis V, Oelkers EH. Riverine particulate material dissolution as a significant flux of strontium to the oceans. Earth Planet Sci Lett. 2012;355/356:51–59.
- Dolin V, Shevchenko O, Brettain J. Artificial radionuclides speciation in river water of DNIEPER basin. In: Barany S, editor. Role of interfaces in environmental protection. Dordrecht, the Netherlands: Kluwer Academic Publishers; 2003. p. 135–144.
- Asano M, Sumino S, Jiku F. Decomposition of Benzo (a) Pyrene on artificial sea water using UV/photocatalytic oxidation process. J Environ Sci Eng A 1. 2012;1:195–201.
- Puigdomenech I. Hydrochemical equilibrium-constant database (HYDRA). Stockholm: Inorganic Chemistry, Royal Institute of Technology; 2009 [cited 2009 Aug 18].
- Puigdomenech I. Make equilibrium diagrams using sophisticated algorithms (MEDUSA). Stockholm: Inorganic Chemistry, Royal Institute of Technology; 2009 [cited 2009 Aug 26].
- Tateda Y, Koyanagi T. Concentration factors for Cs-137 in marine algae from Japanese coastal waters. J Radiat Res. 1994;35:213–221.