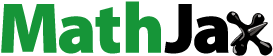
Abstract
Yields of precipitated UO2(NO3)2(NRP)2 (NRP = N-alkylated 2-pyrrolidone) were precisely determined by considering reduction of the solution volume through the precipitation, which can be estimated from difference in acid concentrations of the liquid phases before and after the precipitation. The studied NRPs were N-n-butyl (NBP) and N-n-propyl (NProP) derivatives. In both systems, the precipitation yields precisely determined were always higher than those simply calculated from the ratio of uranium concentrations before and after the precipitation. However, the differences between them are in the range of 0.6%–2.6%. If such a difference is practically negligible, the volume reduction through the precipitation does not have to be taken into account for simplicity of the analytical manipulation.
1. Introduction
The nuclear fuel cycle is one of the issues of special interests under the current social situation in Japan after the accident in the Fukushima Daiichi nuclear power plants. Even under such a circumstance, the spent nuclear fuels discharged from the power plants must be treated properly. Therefore, it is mandatory to find an appropriate method to manage them. Previously, we found that several N-alkylated 2-pyrrolidone derivatives (NRPs, ) may precipitate U(VI) selectively from other metal ions in HNO3 aq. [Citation1–6]. A formula of the U(VI) precipitate is UO2(NO3)2(NRP)2, where two NO3− and two NRP form trans geometry in the equatorial plane of [UO2]2+. On this basis, we have proposed a new reprocessing method for the spent nuclear fuels [Citation7].
Figure 1. Schematic structure of N-alkylated 2-pyrrolidone derivatives (NRPs, R = −CnH2n+1, cycloalkyl).
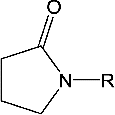
In the previous works, we used precipitation yield (y, %) to evaluate the capability of NRPs as a precipitant for U(VI). This quantity has been simply calculated by taking into account only the U(VI) concentration in the supernatant and that in the initial solution. In the recent phase of our research project, use of a concentrated initial solution of U(VI) was preferred because of a requirement to reduce volume of liquid wastes exhausted from the total process [Citation8]. Accordingly, the actual experiments were typically operated at ca. 1.5 mol L−1 (M) U(VI) in HNO3 aq., which is 1.5 times higher than that in our former studies and the commercial reprocessing plant. To prepare this condition, ca. 15 grams of UO2(NO3)2·6H2O must be dissolved in 20 mL HNO3 aq. When a large part of U(VI) is precipitated by addition of NRP in this system, volume of the liquid phase will be reduced, because UO2(NO3)2 precipitated from the aqueous solution also has some volume. If the volume reduction of the liquid phase is not negligible, the amount of U(VI) in the supernatant after the precipitation would be overestimated. This directly affects evaluation of the precipitation yield, which will be underestimated.
To solve this problem, it is necessary to determine the volume reduction of the liquid phase through the precipitation of UO2(NO3)2(NRP)2. These data are taken into account for precise estimation of the precipitation yield. The difference between the precipitation yields with and without consideration about the volume reduction of the liquid phase is also of interest, because we need to judge if the previous evaluation has been practically reasonable or not. N-n-butyl-2-pyrrolidone (NBP) and N-n-propyl-2-pyrrolidone (NProP) were selected as precipitants in this study.
2. Experimental
2.1. Principles for precise determination of precipitation yields
For precise evaluation of the precipitation yield (y, %) of UO2(NO3)2(NRP)2, the following relationship should be considered:
(1)
(1) where CU and V denote the concentrations of uranium (M) and volumes of solutions (L), respectively. The subscripts ini and sup denote initial solution and supernatant after the precipitate formation, respectively. The uranium concentrations were determined experimentally by inductively coupled plasma atomic emission spectroscopy (ICP-AES, PerkinElmer Optima 3000). Usually, we perform the precipitation experiments of [UO2]2+ in HNO3 system, while any acidic components are not included in the precipitate. This means that the moles of H+ in the liquid phase remain unchanged through the precipitate formation process as follows:
(2)
(2) where the H+ concentrations are denoted by CH with the subscripts indicating the sample status as mentioned above. Thus, we can obtain the volume ratio between the supernatant and initial solution by determining the H+ concentrations in these solutions. Combining Equations (1) and (2), precise evaluation of the precipitation yield y is fulfilled.
Although the H+ concentration can be estimated by ordinary acid–base titration with monitoring pH, it is necessary to take special care to prevent hydrolysis of [UO2]2+, which releases H+ and upsets the titration. According to Motojima and Izawa [Citation9], excess sulfate ion should be added to test solutions to mask [UO2]2+ from the undesired reaction and to determine CH correctly. In such a system, soluble sulfato complexes, [UO2(SO4)n]2−2n (n = 1–3), will be formed [Citation10,11]. The species distribution curves simulated under a typical condition (, [[SO4]2−] = 0.76 M, 1 M ionic strength (I)) are displayed in . In accordance with this diagram, the masking by sulfate is effective up to pH 5. Therefore, the acid–base titration has to come to an end point at pH lower than 5 to avoid perturbation by the [UO2]2+ hydrolysis.
Figure 2. Simulated species distribution diagram in aqueous solution containing [UO2]2+ (50 mM) and [SO4]2− (0.76 M) at 1 M ionic strength [Citation10,11].
![Figure 2. Simulated species distribution diagram in aqueous solution containing [UO2]2+ (50 mM) and [SO4]2− (0.76 M) at 1 M ionic strength [Citation10,11].](/cms/asset/4c23baf2-f47e-4420-bed0-c148810c5657/tnst_a_937782_f0002_b.gif)
Furthermore, the precisely determined y allows us to evaluate how much percentage of added NRP is efficiently used to form the UO2(NO3)2(NRP)2 precipitate. This quantity, E (%), is defined by Equation (3).
(3)
(3) where CNRP denotes the total concentration of NRP added. The coefficient 2 in the numerator originates from the stoichiometry of NRP in the formula of the precipitate.
In addition, it is obvious that the volume reduction of the liquid phase through the precipitation of UO2(NO3)2(NRP)2 arises from the exclusion of UO2(NO3)2 from the solution. If the concentration of the added NRP in the supernatant is negligibly low, i.e., E is close to 100%, one can estimate molar volume (VUNM, L mol−1) of UO2(NO3)2 in this system. This quantity can be provided by the following equation:
(4)
(4)
2.2. Experimental procedure
A stock solution dissolving UO2(NO3)2·6H2O (1.5 M) and HNO3 (3 M) was prepared. To aliquots of this solution (2 mL each) in test tubes were added NBP or NProP under different molar ratios to [UO2]2+, followed by vigorous agitation with a poly(tetrafluoroethylene) stir tip under vortex mixing. The test tubes were stored at 298 K in a thermostat water bath for 1 h. The supernatant in each tube was separated from the precipitate by centrifugation. The uranium concentrations in the initial solution and supernatants were determined by ICP-AES (PerkinElmer Optima 3000). The H+ concentrations in the initial solution and supernatants were evaluated by the following procedure. An aliquot of the liquid phase in each test tube (0.5 or 0.4 mL) was mixed with 0.76 M (NH4)2SO4 aq. (8 mL), followed by titration with 1 N NaOH aq. (f = 0.9901, standardized by sulfamic acid). The end point of each run was defined as a first-order differential peak of the titration curve.
3. Results and discussion
3.1. NBP system
The obtained data in the NBP system are summarized in . All the titration experiments finished below pH 5, indicating that in each sample was correctly determined. With increasing
increases in the first two points, and then reaches plateau at the higher CNBP/CUini conditions. All
determined are higher than CHini, indicating that the volume of the liquid phase is certainly reduced through the precipitation of UO2(NO3)2(NBP)2. From Equation (2), the volume reduction of the liquid phase in each condition has been estimated to give Vsup/Vini. Finally, the precise yields (y) of the UO2(NO3)2(NBP)2 precipitate were determined by using Equation (1). The resulting values were plotted as a function of CNBP/CUini in . The slope of this plot is 53.5, indicating that the stoichiometry of NBP to [UO2]2+ in the precipitate is 2. We also calculated the precipitation yields without taking into account the volume reduction through the precipitation (i.e., Vsup/Vini = 1 in Equation (1)) as we did so previously [Citation7,8]. This quantity is denoted by y′, and also listed in . The difference between y and y′ (Δy, %) is in the range of 0.6%–2.4%, and y is always larger than y′, as predicted. If such a difference is virtually insignificant to consider actual processes like the nuclear fuel reprocessing, the volume reduction through the precipitation does not always have to be taken into account for simplification of experimental manipulation.
Table 1. Summary of NBP system (CUini = 1.56 M, CHini = 3.11 M).
Figure 3. Yield of UO2(NO3)2(NRP)2 precipitate (y, left axis, open symbols) and efficiency of NRP to form the precipitate (E, right axis, solid symbols) as a function of CNRP/CUini. NRP = NBP (circle), NProP (square).
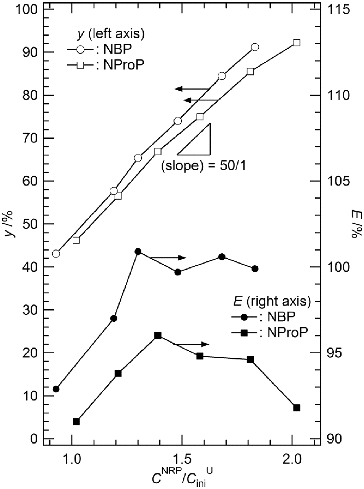
In and , it is noteworthy that E is almost equal to 100% at 1.30 ≤ CNBP/CUini ≤ 1.83. This means that there is little influence from the NBP residue in the supernatants to Vsup/Vini. This situation allows us to calculate VUNM, the molar volume of UO2(NO3)2 in the current system. The average value of VUNM in was evaluated as (5.5 ± 1.6) × 10−2 L mol−1.
3.2. NProP system
The similar experiments have been performed in the NProP system. The obtained data are summarized in . The end points of titrations for all samples were at pH < 5, demonstrating that in each sample has been determined correctly. With elevating
increases, while it turns to slightly decrease at CNProP/CUini > 1.8. All
estimated are greater than CHini, implying the volume reduction of the liquid phase through the precipitation of UO2(NO3)2 (NProP)2. The precise yield of UO2(NO3)2(NProP)2 precipitate (y) at each condition in the current system was estimated in a similar manner to the NBP system described above, and listed in together with the yield without taking into account the volume reduction (y′). The resulting values were also plotted as a function of CNProP/CUini in . The slope of this plot is 46.4, which is in agreement with the formula of UO2(NO3)2(NProP)2. The difference between y and y′ (Δy) is in the range of 0.6%–2.6%, and the relationship y > y′ is always kept. This consequence is similar to that in the NBP system mentioned above, and leads us to the same conclusion, i.e., if such a difference is practically negligible to consider the actual processes for the spent nuclear fuel treatment, the volume reduction of the liquid phase through the precipitation does not have to be taken into consideration.
Table 2. Summary of NProP system (CUini = 1.51 M, CHini = 3.09 M).
The efficiency of NProP to form the precipitate (E) is lower than that of NBP in whole CNRP/CUini series as shown in . Such a difference is reasonably explained by higher solubility of UO2(NO3)2(NProP)2, which is mainly in connection with the lower hydrophobicity of NProP than NBP [Citation6]. According to our former results, UO2(NO3)2(NProP)2 is soluble in 3 M HNO3 aq. up to 0.14 M, whereas the solubility of UO2(NO3)2(NBP)2 in the same solution is only 0.062 M. Since the unreacted NProP dissolves in the supernatants of all the samples, VUNM was not estimated in this system.
4. Conclusion
The precise determination of yield of UO2(NO3)2(NRP)2 precipitate has been done by taking into account the volume reduction of the liquid phases. Consequently, the precipitation yields evaluated precisely are always higher than those simply estimated from the ratio of uranium concentrations in the liquid phases. The actual deviation is in the range of 0.6%–2.6%. If such a difference is negligible in a practical sense, the volume reduction through the precipitation does not have to be considered for simplicity of the analytical manipulation. Regarding the precipitation yield in our previous publications, the reported data were always underestimated. However, the deviations from the correct values should be less than 3%. Therefore, this level of confidence is, at least, secured for the results on the precipitation of UO2(NO3)2(NRP)2 we reported previously.
Acknowledgements
This work is the result of ‘Development of Advance Reprocessing System Based on Use of Pyrrolidone Derivatives as Novel Precipitants with High Selectivity and Controllability’ entrusted to Tokyo Institute of Technology by the Ministry of Education, Culture, Sports, Science and Technology of Japan (MEXT).
References
- Varga TR, Sato M, Fazekas Z, Harada M, Ikeda Y, Tomiyasu H. New uranyl nitrate complex with N-cyclohexyl-2-pyrrolidone: a promising candidate for nuclear fuel reprocessing. Inorg Chem Commun. 2000;3:637–639.
- Varga TR, Bényei AC, Fazekas Z, Tomiyasu H, Ikeda Y. Molecular and crystal structure of bis(N-cyclohexyl-2-pyrrolidone)dioxouranium(VI) nitrate. Inorg Chim Acta. 2003;342:291–294.
- Ikeda Y, Wada E, Harada M, Chikazawa T, Kikuchi T, Mineo H, Morita Y, Nogami M, Suzuki K. A study on pyrrolidone derivatives as selective precipitant for uranyl ion in HNO3. J Alloys Compounds. 2004;374:420–425.
- Koshino N, Harada M, Nogami M, Morita Y, Kikuchi T, Ikeda Y. A structural study on uranyl (VI) nitrate complexes with cyclic amides: N-n-butyl-2-pyrrolidone, N-cyclohexylmethyl-2-pyrrolidone, and 1,3-dimethyl-2-imidazolidone. Inorg Chim Acta. 2005;358:1857–1864.
- Takao K, Noda K, Morita Y, Nishimura K, Ikeda Y. Molecular and crystal structures of uranyl nitrate complexes with N-alkylated 2-pyrrolidone derivatives: design and optimization of promising precipitant for uranyl ion. Crystal Growth Des. 2008;8:2364–2376.
- Takao K, Noda K, Nogami M, Sugiyama Y, Harada M, Morita Y, Nishimura K, Ikeda Y. Solubility of uranyl nitrate precipitates with N-alkyl-2-pyrrolidone derivatives (alkyl = n-Propyl, n-Butyl, iso-Butyl, and Cyclohexyl). J Nucl Sci Technol. 2009;46:995–999.
- Koshino N, Harada M, Morita Y, Kiikuchi T, Ikeda Y. Development of a simple reprocessing process using selective precipitant for uranyl ions: fundamental studies for evaluating the precipitant performance. Prog Nucl Energy. 2005;47:406–413.
- Morita Y, Takao K, Kim S-Y, Kawata Y, Harada M, Nogami M, Nishimura K, Ikeda Y. Development of advanced reprocessing system based on precipitation method using pyrrolidone derivatives as precipitants – precipitation behavior of U(VI), Pu(IV), and Pu(VI) by pyrrolidone derivatives with low hydrophobicity. J Nucl Sci Technol. 2009;46:1129–1136.
- Motojima K, Izawa K. Potentiometric titration of free acid and uranium in uranium(VI) solutions with alkali. Anal Chem. 1964;36:733–735.
- Grenthe I, Fuger J, Konings RJM, Lemire RJ, Muller AB, Nguyen Trung C, Wanner H. Chemical thermodynamics of uranium. Paris, France: OECD NEA; 1992.
- Guillaumont R, Fanghanel T, Neck V, Fuger J, Palmer DA, Grenthe I, Rand MH. Update on the chemical thermodynamics of uranium, neptunium, plutonium, americium and technetium. Amsterdam: Elsevier B.V.; 2003.