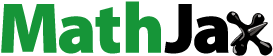
Abstract
The effects of impurity Zr on dissolution behavior into nitric acid of sintered Mo pellets were investigated using simulated fuels to accumulate basic data and knowledge concerning the matrix of Mo-cermet fuels. Mo pellets containing 1– 10 mol% Zr were prepared by conventional powder metallurgical route. After characterization tests using optical microscopy (OM), X-ray diffraction and secondary electron microscopy-energy dispersive X-ray spectrometry (EDX), the sintered pellets were subjected to dissolution tests by nitric acid. The apparent dissolution rate of Mo pellets containing impurity Zr was found to be followed by the first-order chemical reaction form, which is generally known as a simple chemical reaction. The rate of dissolution of sintered Mo-cermet fuels by nitric acid was found to decrease with the increase of the amount of impurity Zr.
1. Introduction
The molybdenum (Mo)-incorporated fast reactor cycle is one option for the recovery and utilization of Mo fission product [Citation1,Citation2]. In the cycle, Mo fission product is thought to be recycled as an inert matrix of Mo-cermet fuel and is expected to separate from nitric acid (HNO3)-containing solution from spent fuels by aqueous processes [Citation3]. Mo-cermet fuel is expected to have many advantages such as high thermal performance, adaptability to the conventional fabrication process, adaptability to aqueous reprocessing systems and so on [Citation4,Citation5]. A basic understanding of the behavior in sintering and dissolution into HNO3 are key issues for the design of suitable schemes for the fabrication and reprocessing of Mo-cermet fuel. Although several fundamental studies on sintering and dissolution behavior have been conducted to date [Citation2,Citation3,Citation6,Citation7], many factors affecting sintering and dissolution behaviors require further investigation. It is known that zirconium (Zr) can accompany recovered Mo as an impurity, [Citation5] and this would affect several properties of Mo-cermet fuel. In this study, the effects of impurity Zr on sintered density and dissolution behavior into HNO3 of matrix phase Mo pellets were investigated to accumulate basic data and knowledge concerning the matrix of Mo-cermet fuels.
2. Experimental
In this study, Mo-metal powder mixed with a small amount of ZrO2 powder was used for sintering and dissolution tests. The composition of the mixed powder assumes that recovered Mo is synthesized from HNO3 solution containing separated Mo and a small amount of Zr as impurity [Citation3] by means of a simple method of heating Mo- and Zr-containing HNO3 solution [Citation2]. Since the conversion of the precursor oxide of Mo with Zr impurity that is synthesized from the HNO3 solution is usually performed by heating in a reductive atmosphere with oxygen potential slightly less than MoO2/Mo, ZrO2 should remain as an oxide in such oxygen potential due to the much stronger affinity of Zr to oxygen than Mo. As a result, the final heat-treated product of the recovered Mo should be Mo-metal powder with ZrO2 like the test powder. Specimens containing 1, 5 and 10 mol% Zr in Mo were prepared by a conventional powder metallurgical route. Powders of Mo (A.L.M.T. Corp.; 99.95% purity; average particle size, 3 mm) and ZrO2 (RARE METALLIC Co. LTD.; purity 99.99%) were used as raw materials. Appropriate amounts of Mo and ZrO2 powders were weighed using an electronic balance in order to adjust the predetermined weight of the metallic ratio of Zr and Mo. The weighed powders with a small amount of polyvinyl alcohol binder were thoroughly mixed in an agate mortar with a pestle in acetone medium. The mixed powder was then compacted into pellet specimens with a uniaxial pressing unit at a pressure of 200 MPa. The compacted pellets were then heated at 1873 K for 4 h in a flow of Ar containing 4% H2. As a reference, pure Mo pellets were also prepared by the same procedure. The diameter and height of sintered pellets were approximately 5.3 and 4.1 mm, respectively.
The densities were calculated using the measured weights, diameters and heights of the sintered pellets. The sintered pellets were then subjected to characterization tests. The pellet specimens were mounted to holders using epoxy resin, and then were ground and mirror polished with an anhydrous lubricant. The specimens were observed by OM and secondary electron microscopy (SEM). OM was conducted using BX51M model (OLYMPUS) and SEM observations were carried out using the Tiny-SEM Mighty-8 model (Technex Lab Co., Ltd.). Immediately prior to SEM observations of the specimens, a thin film of Au–Pd was applied to their surfaces by vacuum evaporation to avoid electron charging effects. The crystal structures of the specimens were analyzed by X-ray diffraction (XRD) method using Cu Kα radiation at room temperature with a Rigaku model RAD-C system and MiniFlex II.
The sintered pellets were subjected to dissolution test in HNO3 solution. Each of the specimens was placed into a beaker and 8 M HNO3 was poured on the specimen. The amount of 8 M HNO3 was 13 ml per 1 g specimen. The dissolution tests on reference Mo pellets were first carried out for approximately 200 min at temperatures from 293 to 373 K to select a suitable temperature for Mo dissolution. The specimens containing Zr impurity were then subjected to dissolution test at an appropriate temperature. The dissolution ratio of Mo was calculated using their concentrations in the solution, which were determined by inductively coupled plasma atomic emission spectrometry (ICP-AES).
3. Result and discussion
shows the relative density (the measured density against the theoretical density; %TD) of sintered Mo pellets as a function of Zr content. The sintered relative density of Mo pellets decreased with the increase of impurity content for Zr. The microstructures of the Mo pellets containing 1, 5 and 10 mol% Zr observed by OM and SEM are shown in . Dark gray phase inclusions appeared clearly on the specimens; and the size and number of these precipitations increased with increasing Zr content. The porosity of the Mo pellets also increased with increasing Zr content; and significant porous structure was observed in the Mo pellet specimen containing 10 mol% Zr, as shown in . Energy dispersive X-ray spectrometry (EDX) point analysis on the surface of Mo pellet containing Zr showed Zr in the dark gray inclusions, whereas Mo was detected in the matrix of the pellet. An example of XRD pattern obtained at room temperature on the Mo pellet specimen containing impurity Zr is provided in . In addition to the XRD patterns of Mo matrix, weak peaks derived from ZrO2 were obtained. According to the results of the above characterization tests, the impurity Zr was dispersed throughout the pellet as oxide (ZrO2) in the experimental condition of this study. As shown in , the density decreases monotonically with the increase of impurity Zr. It is thought that impurity Zr acts as an inhibitor to suppress the grain growth of Mo during the sintering to produce the porous structure of the pellets. From these results, Zr should be removed at the Mo separation stage because of its unfavorable influence on sintered density, which affects the thermal conductivity of the Mo-cermet matrix.
Figure 2. The microstructures of the Mo pellets containing 1, 5 and 10 mol% Zr observed by OM and SEM.
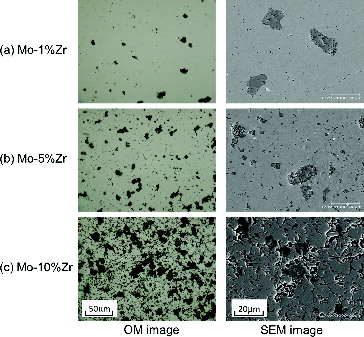
The dissolution ratio of Mo in HNO3 after dissolution tests at 293, 313, 323 and 373 K in the reference Mo pellets was determined by the results of ICP-AES analysis. The dissolution ratio of Mo decreased with the increase of temperature. Molybdenum was completely dissolved at 293 K in HNO3 and was practically insoluble at 373 K. A white-colored precipitate was observed in dissolution tests at 323 and 373 K. The precipitate formed in the dissolution test at 373 K was washed with water, filtered by millipore filter, dried at 373 K and subjected to XRD analysis. No diffraction angles derived from Mo metal were observed, and they showed values near to those of MoO3·1/2H2O. Benard et al. [Citation8] reported that MoO3·1/2H2O precipitation formed in 6M HNO3 at 333 K. This implies that Mo was initially dissolved into HNO3 solution and that the precipitation of Mo oxides occurred simultaneously in the HNO3 solution at a higher dissolution temperature. It was found that the HNO3 at approximately room temperature is suitable for the dissolution of Mo. This result supports the pre-removal of Mo at room temperature, in which the proposed reprocessing scheme concept for the cermet fuel [Citation2] is reasonable.
Referring to the observed effect of temperature on the dissolution behavior of Mo pellet, it was decided to conduct dissolution testing with a focus on the effect of impurity Zr on Mo pellet dissolution behavior at 293 K. shows a plot of ln (w/w0) vs. t which indicates the change of the weight of the Mo pellets containing impurity Zr in the dissolution test in HNO3 at room temperature (293 K) together with the reference Mo pellet. The weight of pellet specimens, which were removed from the HNO3 solution with tweezers, was measured after rinsing with a sufficient amount of pure water and dried well at 373 K. The weight change ratio of the specimen pellet, w/w0, where w is the weight of each time step and w0 is initial weight, corresponds to the change in concentration of dissolved materials into the solution. The values of w/w0, therefore, are an indicator of the apparent dissolution rate of Mo into solution. The data in is limited to the specimens that could be removed with tweezers. Small pieces which could not be picked up with tweezers, therefore, remained in the HNO3 solution as residue. Although the weight change behavior of reference Mo pellet and 1 mol% Zr containing Mo pellet was nearly the same, the change of the weight of the specimens slowed with increasing impurity Zr content. As shown in , the linearity was good for each specimen. This implies that the time dependence of the weight change ratio of the specimen pellets could be described as
(1)
(1) where k is a rate constant. It was found that the apparent dissolution rate of Mo pellets containing impurity Zr was followed by the first-order chemical reaction form, which is generally known as a simple chemical reaction. By comparing the value of k, which is determined by the gradient of the respective data in , the dissolution rate of Mo pellet decreased with the increase of impurity Zr content; that is, the apparent dissolution rates at 5% and 10% Zr-containing Mo pellet were one-third and one-tenth that of Mo, respectively. The amount of Mo in HNO3 solution after the dissolution test for each specimen in was determined by ICP-AES analysis, and the dissolution ratio of Mo was calculated by comparing the initial amount of Mo. shows the dissolution ratio of Mo in Mo pellets containing impurity Zr at room temperature. The dissolution ratio of Mo decreased with increasing impurity Zr. Quantitative image analyses were carried out on SEM images using Win ROOF ver.5.8 (Mitani Corp.). The areal fractions of ZrO2 phase on Mo pellets containing 1, 5 and 10 mol% Zr were found to be approximately 2.0%, 10.6% and 19.4%, corresponding to the areal fractions of Mo, 98.0%, 89.4% and 80.6%, respectively. Although the values of the areal fractions of Mo did not correspond completely to the dissolution ratio of Mo, the tendency toward decreasing value was similar. A possible reason for the decrease of dissolution ratio of Mo with the increase of impurity Zr in this study, therefore, is the decrease of effective contact area of Mo to HNO3 solution with the increase in the amount of ZrO2 phase. It is known that Mo has multivalences and forms various complexes in aqueous solution, which increase the complexity of Mo solution-chemistry significantly [Citation9]. Although the detailed dissolution mechanism of Mo pellets containing impurity Zr remains unclear, the existence of impurity Zr in Mo is unfavorable for efficient dissolution in the proposed reprocessing scheme [Citation2]. In view of these fundamental results, Zr should be removed at the Mo separation stage because of its unfavorable influence on the dissolution behavior of Mo-cermet. Results obtained in this study may also be useful in defining various basic criteria for fuel fabrication, such as tolerance data for impurities in the recovered Mo from the viewpoint of sintering and reprocessing for Mo-cermet fuels.
4. Summary
In order to accumulate basic data and knowledge concerning the matrix of Mo-cermet fuels, the effects of impurity Zr on sintered density of compacted Mo pellets and on dissolution behavior in nitric acid of sintered Mo pellets were investigated using simulated fuels. The sintered density of Mo pellets decreased with the increase of impurity content of Zr. The apparent dissolution rate of Mo pellets containing impurity Zr was found to be followed by the first-order chemical reaction form, which is generally known as a simple chemical reaction. The dissolution ratio of Mo in HNO3 decreased with increase of the amount of impurity Zr. In view of these fundamental results, Zr should be removed at the Mo separation stage because of its unfavorable influence on the sintered density and dissolution behavior of Mo-cermet.
Acknowledgements
The authors would like to express their appreciation to Messrs. Takayuki Seki, Naoki Kashimura and Ken Sekioka (Inspection Development Company) for their technical support in the experiments.
References
- Osaka M, Koi M, Takano S. A novel concept for americium-containing target for use in fast reactors. J Nucl Sci Technol. 2006;43:367–374.
- Osaka M, Takano S, Yamane Y, Misawa T. On a fast reactor cycle scheme that incorporates a thoria-based minor actinide-containing cermet fuel. Prog Nucl Energy. 2008;50:212–218.
- Osaka M, Miwa S, Tanaka K, Akutsu Y, Ikeda K, Mimura H, Suzuki T, Usuki T, Yano T. Reformation of hazardous wastes into useful supporting materials for fast reactor fuels. Ann Nucl Energy. 2011;38:2661–2666.
- Chauvin N, Konings RJM, Matzke HJ. Optimization of inert matrix fuel concept for americium transmutation. J Nucl Mater. 1999;275:105–111.
- Fernandez A, Konings RJM, Somers J. Design and fabrication of specific ceramic–metallic fuels and targets. J Nucl Mater. 2003;319:44–50.
- Osaka M, Tanaka K. Densification of molybdenum-cermet fuel by sintering with metal additives. J Nucl Sci Technol. 2008;45:970–974.
- Osaka M, Tanaka K, Sekine S, Akutsu Y, Suzuki T, Mimura H. Morphology-controlled synthesis of Mo compounds from a nitric acid solution by the microwave heating and/or Zr-addition. J Nucl Mater. 2012;427:384–388.
- Benard P, Seguin L, Louer D, Figlarz M. Structure of MoO3 1/2H2O by conventional X-ray powder diffraction. J Solid State Chem. 1994;108:170–176.
- Cotton FA, Wilkinson G. Advanced inorganic chemistry. 5th ed. New York (NY): John Wiley & Sons, Inc.; 1988.