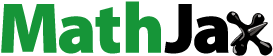
Abstract
The deuterium (D) permeation behaviors for ion-damaged tungsten (W) by 3 keV D2+ and 10 keV C+ were studied. The D permeability was obtained for un-damaged W at various temperatures. For both D2+ and C+ implanted W, the permeability was clearly reduced. But, for the D2+ implanted W, the permeability was recovered by heating at 1173 K and it was almost consistent with that for un-damaged W. In the case of C+ implanted W, the permeability was not recovered even if the sample was heated at 1173 K, indicating that the existence of carbon would prevent the recovery of permeation path in W. In addition, transmission electron microscope (TEM) observation showed the voids were grown by heating at 1173 K and not removed, showing the existence of damages would not largely influence on the hydrogen permeation behavior in W in the present study.
Keywords:
1. Introduction
Tungsten (W) is considered as a promised material for plasma facing components of International Thermonuclear Experimental Reactor (ITER) and future fusion devices [Citation1,Citation2]. Evaluation of fuel behavior, namely hydrogen isotope behavior, for W is one of critical issues for fusion safety [Citation3]. In our previous studies, the behavior of deuterium (D) retentions for Fe2+ irradiated W with the damage of 0.025–3 dpa was compared with that for neutron-irradiated W with 0.025 dpa [Citation4–10]. It was reported that D2 thermal desorption spectroscopy spectra for Fe2+-irradiated W could not represent that for neutron-irradiated one, indicating that the D trapping and desorption mechanism for neutron-irradiated W has a difference from that for ion-irradiated one. This means that the evaluation of hydrogen isotope in W with various damage conditions is quite important to understand the mechanism of hydrogen behavior in W. On the other hand, it is thought that surface modification is caused by the change in the surface chemical state by the reaction of W with carbon, an impurity, which will enhance hydrogen isotope trapping and introduce irradiation damage structure by energetic ion implantation [Citation11–15]. The simultaneous C+ and D2+ implantation enhanced the D retention in W. Especially, D was stably trapped by damages produced by C+ implantation. Therefore, energetic C+ implantation largely contributes on the D retention behavior.
Recently, Tanabe has reviewed the retention and permeation behavior of hydrogen retention in W [Citation16]. He has mentioned that both defects and impurities could work as trapping sites and the H/W ratio on the surface under energetic H implantation should be 0.001–0.1. The reliable diffusion coefficients by plasma-driven permeation and gas-driven permeation have been proposed [Citation17,Citation18]. However, it is quite important to evaluate the diffusion behavior for damaged W to extrapolate the actual hydrogen isotope behavior under actual fusion environment. Therefore, in this study, the damages were introduced by energetic D2+ or C+ implantation and gas permeation behavior at various temperatures was evaluated and this result was compared with that for undamaged W.
2. Experimental
shows the flow chart of the gas permeation measurement system. The heating part for D permeation including sample holder consisted of double tubing of 1/4 inch inner stainless steel tubing and 1/2 inch outer quartz tubing to reduce the oxidation during heating. The sample was fixed with 1/4 inch VCR (Sewgelok Inc.) tubing by sandwiching between silver-coated metal gaskets. A thermocouple was introduced to inner tubing and contacted with the upstream side of sample surface directly to measure the sample temperature. Ar gas was flowed between the inner stainless steel tubing and outer quartz tubing to reduce the oxidation of inner stainless steel tubing. Before the permeation test, both of upstream side and downstream side was evacuated by a turbo molecular pump up to the vacuum less than 10−5 Pa. Thereafter, D2 gas was introduced into upstream side. The D permeated through the sample was directly introduced into a quadrupole mass spectrometer (SPECTRA Microvision plus), which was placed in the downstream side.
The W foil (The Nilaco Corporation) (10×10×0.05 mm3) was preheated at 1173 K for 30 minutes under ultrahigh vacuum to remove the surface impurities and intrinsic damages. After preheating, the sample was exposed to the D2 gas. The pressure range of D2 gas was between 3.00 and 45.00 kPa, and the temperature range was set to be 773–1173 K.
The damage was introduced in the sample by 3.0 keV D2+ or 10.0 KeV C+ ion implantation, with the flux of 1.0×1018 D+ m−2 or 1.0×1017 C+ m−2 up to the fluence of 3.0×1022 D+ m−2 or 1.0×1021 C+ m−2 at room temperature, respectively. The detail experimental setup was described in our previous papers [Citation11–15]. Thereafter, the sample was placed in the gas permeation measurement system and permeation experiment was done at the temperature between 623 and 1173 K.
3. Results and discussion
shows the temperature dependence on permeation coefficient for un-damaged W. The previous results were also included in this figure [Citation18–20]. According to the diffusion-limited permeation, the permeation coefficient at the temperature above 773 K (P) was determined to be as follows;
(1)
(1) However, the activation energy was determined to be 0.76 eV for the present study with the temperature range of 773–1173 K, and 1.4 eV for Frauenfelder's result with the temperature range of 1100– 2400 K [Citation19], and 0.58 eV for Ikeda's result with the temperature range of 473–673 K [Citation18]. The difference between the present research and pervious research may be caused by the difference in experiment temperature range. The activation energy for diffusion inside the grain boundaries was reported to be 0.23 eV [Citation21] by first principles calculations, although that between crystal interstitial was 0.38 eV [Citation18], and these results were almost consistent with our present results. Thus, for the temperature above 773 K, the hydrogen diffuse through both lattice and grain boundaries will be the possible diffuse path, with an activation energy of 0.76 eV.
Figure 2. D permeability for D2+ implanted W at various temperatures, as compared with un-damaged W and other experiments data.
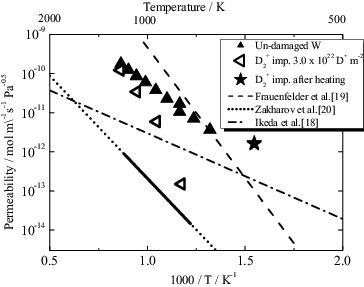
The D permeation coefficient for D2+ implanted W is also shown in . It was clear that the reduction of D permeation coefficient by D2+ implantation was found. Especially, large reduction could be seen at lower temperature of ∼973 K. The D permeation coefficient for D2+ implanted W was reached to the same value with that for un-damaged W as the sample was heated up to 1173 K.
In , the additional desorption stage for D2+ implanted W with higher fluence above 2.5×1022 D+ m−2 was found at 700 K, according to our previous study, which would be caused by the accumulation of irradiation damages, namely voids [Citation15]. Also, according to the transmission electron microscope (TEM) image in , nano-voids was formed after annealing, these nano-voids should be formed by the accumulation of irradiation damages, such as vacancy and void which formed before annealing. As the D2+ implantation was the only difference between un-damaged sample and the D2+ implanted sample, thus the defects produced by D2+ implantation should be the one reason for the reduction of D permeation coefficient. The perfect crystal will be a good diffusion path for D, while most of irradiation damages will be possible trapping sites for D. However, due to the D2+ implantation, these crystal interstitials became trapping sites, and were filled by D after steady-state permeation. These trapping sites will refrain the D diffusion in W, leading to the reduction of D permeation.
Figure 3. D2 TDS spectra for D2+ implanted W with the ion fluence between 1.0×1022 D+ m−2 and 1.0×1023 D+ m−2.
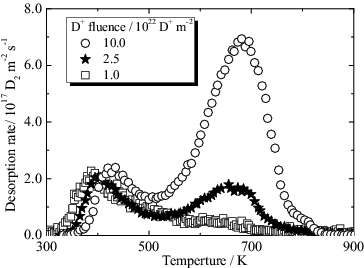
Figure 4. TEM micrographs for D2+ implanted W with the ion fluence of 1.0×1022 D+ m−2 and 1.0×1023 D+ m−2, both before and after annealing micrographs are shown here.
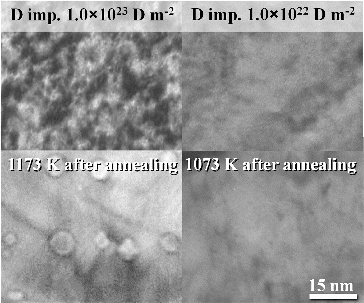
By heating at 1173 K, the permeation coefficient was increased and almost consistent with that for un-damaged W. However, the TEM observation as shown in indicated that the damages introduced by D2+ implantation was not recovered by heating at 1173 K and damages would be accumulated and nano-voids should be grown largely. Especially for the high fluence case, many nano-voids were formed after annealing, which is consistent with our previous results [Citation15]. This fact implies that the existence of damages after annealing in W does not influence on the D permeation behavior, which was quite different from the D retention behavior, where the nano-voids would be potential stable trapping sites for hydrogen isotopes. For the present experiments, as shown in , the D retention were dramatically increased for large D2+ implantation fluence, which should be trapped by the irradiation damages, such as vacancy and voids. The simulation results also showed that the vacancy-like defects could be the possible trapping sites for hydrogen isotopes [Citation22]. These facts were consistent with the previous report by Y. Ueda that the damages did not largely affect the hydrogen permeation behavior in W [Citation23].
To evaluate the impurity effect on D permeation in W, the 10 keV C+ was implanted into W and its D permeation behavior was compared with that for un-damaged W and D2+ implanted W. As shown in , the D permeation was reduced at the temperature of 973 K, which was almost the same as that for D2+ implanted W. The D permeation coefficient was still lower than that for un-damaged W at 1173 K, which is different from that for D2+ implanted W. By changing the temperature from 1173 to 973 K, the D permeation coefficient was not recovered. This behavior was quite different from that for D2+ implanted W. However, as shown in , the voids have grown by heating for C+ implanted W. These results indicate that the existence of C as impurity would prevent the recovery of trapping sites, because the C will still occupy the irradiation defects even after annealing, thus D could not diffuse through the trapping side due to the existence of C. So, C could block D diffusion in W, thus prevent the recovery of permeation coefficient. The implanted C was not removed by heating at 1173 K. The carbon has high affinity with hydrogen and dissolved hydrogen would be trapped by W–C mixed layer and fill the trapping/diffusion sites in W, leading to the reduction of permeation coefficient for C+ implanted W. It can be concluded that the existence of carbon has a large influence on hydrogen permeation behavior in W.
4. Conclusions
The D permeation behaviors for ion damaged W by 3 keV D2+ and 10 keV C+ were studied. The D permeability was obtained for un-damaged W with the temperature range of 773– 1173 K. For the D2+ implanted W, the permeability was clearly reduced, but it was recovered by heating at 1173 K and it was almost consistent with that for un-damaged W. However, it was not recovered by heating at 1173 K for the C+ implanted W, indicating that the existence of carbon would largely prevent the recovery of permeation path in W.
Acknowledgements
This work is performed with the support and under the auspices of the NIFS – University of Toyama Collaboration Research program, NIFS13KUHR021, ITER BA activity and Kyushu University collaboration program.
Disclosure statement
No potential conflict of interest was reported by the authors.
References
- Roth J, Schmid K. Hydrogen in tungsten as plasma-facing material. Phys Scr. 2011;T145:014031.
- Ueda Y, Lee H, Ohno N, et al. Recent progress of tungsten R&D for fusion application in Japan. Phys Scr. 2011;T145:014029.
- Alimov VK, Hatano Y, Tyburska-Püschel B, et al. Deuterium retention in tungsten damaged with W ions to various damage levels. J Nucl Mater. 2013;441:280–285.
- Oya Y, Shimada M, Kobayashi M, et al. Comparison of deuterium retention for ion-irradiated and neutron-irradiated tungsten. Phys Scr. 2011;T145:014050.
- Shimada M, Hatano Y, Calderoni P, et al. First result of deuterium retention in neutron-irradiated tungsten exposed to high flux plasma in TPE. J Nucl Mater. 2011;415:S667–S671.
- Shimada M, Cao G, Hatano Y, et al. The deuterium depth profile in neutron-irradiated tungsten exposed to plasma. Phys Scr. 2011;T145:014051.
- Shimada M, Hatano Y, Oya Y, et al. Overview of the US-Japan collaborative investigation on hydrogen isotope retention in neutron-irradiated and ion-damaged tungsten. Fusion Eng Des. 2012;87:1166–1170.
- Hatano Y, Shimada M, Oya Y, et al. Retention of hydrogen isotopes in neutron irradiated tungsten. Mater Trans. 2013;54:437–441.
- Hatano Y, Shimada M, Otsuka T, et al. Deuterium trapping at defects created with neutron and ion irradiations in tungsten. Nucl Fusion. 2013;53:073006.
- Kobayashi M, Shimada M, Hatano Y, et al. Deuterium trapping by irradiation damage in tungsten induced by different displacement processes. Fusion Eng Des. 2013;88:1749–1752.
- Oya Y, Kobayashi M, Fujishima T, et al. Behaviors of deuterium retention and microstructure change of tungsten simultaneously implanted with carbon and/or helium ions. Mater Trans. 2013;54:430–436.
- Oya Y, Kobayashi M, Kurata R, et al. Dynamics of hydrogen isotope trapping and detrapping for tungsten under simultaneous triple ion (C+, D-2(+) and He+) implantation. J Nucl Mater. 2011;415:S701–S704.
- Oya Y, Kobayashi M, Kurata R, et al. Comparison of hydrogen isotope retention and irradiation damage behaviors in tungsten and SS-316 with simultaneous C+-D-2(+) implantation. Fusion Eng Des. 2011;86:1776–1779.
- Oya Y, Suzuki S, Wang WJ, et al. Correlation between deuterium retention and microstructure change for tungsten under triple ion implantation. Phys Scr. 2009;T138:014051.
- Miura R, Fujishima T, Uchimura H, et al. Influence of tungsten–carbon mixed layer and irradiation defects on deuterium retention behavior in tungsten. Fusion Eng Des. 2013;88:1827–1830.
- Tanabe T. Review of hydrogen retention in tungsten. Phys Scr. 2014;T159:014044.
- Ikeda T, Otsuka T, Tanabe T. Application of tritium tracer technique to determination of hydrogen diffusion coefficients and permeation rate near room temperature for tungsten. Fusion Eng Des. 2011;60:1463–1466.
- Ikeda T, Otsuka T, Tanabe T. Hydrogen permeation in metals near room temperature by a tritium tracer technique. J Nucl Mater. 2011;417:568–571.
- Frauenfelder R. Solution and diffusion of hydrogen in tungsten. J Vac Sci Technol. 1969;6:388–397.
- Zakharov A, Sharapov V, Evko E. Hydrogen permeability of polycrystalline and single-crystal Mo and W. Fiziko Khim Mekhan Mat. 1973;9:29–33.
- Zhou H-b, Shuo J, Zhang Y, et al. Effects of hydrogen on a tungsten grain boundary: a first-principles computational tensile test. Prog Nat Sci. 2011;21:240–245.
- Liu YL, Zhang Y, Zhou HB, et al. Vacancy trapping mechanism for hydrogen bubble formation in metal. Phys Rev B. 2009;79:172103.
- Ueda Y, Lee HT, Peng HY, et al. Deuterium permeation in tungsten by mixed ion irradiation. Fusion Eng Des. 2012;87:1356–1362.