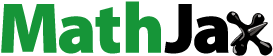
ABSTRACT
2,6-Bis(5,6-diisohexyl-1,2,4-triazin-3-yl)pyridine (isoHexyl-BTP) is a nitrogen-donor chelating ligand which shows high extraction selectivity for minor actinides (MA) over lanthanides (Ln). We synthesized a macroporous sillica/polymer-based isoHexyl-BTP adsorbent (isoHexyl-BTP/SiO2-P) for the separation of MA(III) from Ln(III) in high-level liquid waste. The work focused on the isoHexyl-BTP/SiO2-P adsorption mechanism towards Ln(III) in nitric acid solution and the coordination chemistry between Ln(III) and isoHexyl-BTP/SiO2-P through batch adsorption experiments, extended X-ray absorption fine spectroscopy, acid-base titration and ion chromatography. It was found that both H+ and NO3− directly participated in the adsorption reaction. For the middle and heavy Ln(III), Ln(isoHexyl-BTP/SiO2-P)3(NO3)3·3HNO3 was supposed as the main product of the adsorption. The Ln(III)-N (Ln(isoHexyl-BTP/SiO2-P)33+) bond length of the first coordination layer decreased as the atomic number of Ln(III) increased, which explains the increased adsorption affinity of isoHexyl-BTP/SiO2-P towards middle and heavy Ln(III) in relatively high concentration nitric acid as the Ln(III) atomic number increased.
1. Introduction
Minor actinides (MA: Am, Cm, etc.) are the main long-term radiotoxicity contributors of high-level liquid waste (HLLW) issued from spent fuel reprocessing process plutonium uranium recovery by extraction (PUREX). If these radionuclides are removed from the waste and transmuted into stable or short-lived nuclides by neutron fission, which is the so-called partitioning & transmutation project (P&T) [Citation1], the residual waste loses most of its long-term radiotoxicity. Thus P&T is considered as the key step for both reducing the burden on geological disposal of nuclear waste and facilitating HLLW long-term management. However, since some lanthanides (Ln), e.g., Sm, Eu, Gd, are neutron poisons with large neutron absorption cross-sections and can absorb neutron severely during the transmutation of MA, it is necessary to separate MA from Ln [Citation2]. MA(III) and Ln(III) have overlapping ionic radii and the same valence state leading to their similar chemical properties. In addition, Ln contents are up to 50 times of those of MA in HLLW, so it is a challenging task to realize the separation [Citation2]. The task of MA–Ln separation is not feasible using common oxygen-donor chelating agents. However, some chelating agents with soft donor atoms such as N- or S- can achieve the selectivity, e.g., CYANEX 301 [Citation3–5], BTPs used in SANEX processes [Citation6]. 2,6-Di(1,2,4-triazin-3-yl)pyridines (BTPs), which were firstly used for MA–Ln separation by Kolarik et al [Citation7], are highly effective chelating agents for the separation of MA from Ln in nitric acid solution with the separation factor reaching to around one hundred and are potential candidates for partitioning MA from HLLW [Citation7–28]. In addition, since BTPs only contain C, H and N, they can be incinerated to gaseous products after use up, thereby reducing the volume of secondary radioactive waste.
IsoHexyl-BTP/SiO-P adsorbent (isoHexyl-BTP: 2,6-bis(5,6-diisohexyl)-1,2,4-triazin-3-yl)pyridine) was synthesized by impregnating isoHexyl-BTP into macroporous silica/polymer composite support (SiO2-P) particles and was used in the partitioning process of MA from HLLW based on extraction chromatography proposed by Wei et al. Its adsorption behavior towards MA and Ln has been studied previously [Citation10–15]. IsoHexyl-BTP/SiO2-P exhibited high adsorption affinity and selectivity towards 241Am(III) over 152Eu(III) with the separation factor SF(Am/Eu) reaching to around 100 [Citation12]. Moreover, it showed relatively good stability in 3 M (M = mol/L) HNO3 solution [Citation13]. These studies showed that isoHexyl-BTP/SiO2-P is a very promising candidate for separating MA from HLLW. However, the adsorption mechanism of isoHexyl-BTP/SiO2-P towards MA and Ln(III) has not been clarified satisfactorily. This work focused on the adsorption mechanism of isoHexyl-BTP/SiO2-P towards Ln(III) in simulated HLLW through batch adsorption experiment, extended X-ray absorption fine spectroscopy (EXAFS), acid-base titration and ion chromatography. The work was mainly performed from the following two aspects. First, the effect of H+ and NO3− on the adsorption which was evaluated by comparing the different adsorption behavior of isoHexyl-BTP/SiO2-P towards Ln(III) in nitric acid system and in 0.01 M HNO3–NaNO3 system. Second, the coordination chemistry of isoHexyl-BTP/SiO2-P towards the middle and heavy Ln(III), i.e., Sm(III), Eu(III), Gd(III) and Dy(III), was analyzed using EXAFS to understand the adsorption coordination chemistry and selectivity of isoHexyl-BTP/SiO2-P towards Ln(III) on a molecular level.
2. Experimental
2.1. Reagents
The chemical reagents Ln(NO3)3·nH2O (Ln: Y, La, Ce, Pr, Nd, Sm, Eu, Gd, Dy, Er, Yb, Lu, n = 5 or 6), Ho2O3 and Tm2O3 were commercial reagents of analytical grade. Other reagents such as nitric acid, dichloromethane, etc., were analytical grade and used without further treatment.
The purity of synthesized reagent, isoHexyl-BTP, was 99%. SiO2-P refers as the macroporous silica/polymer composite support particles (SiO2-P) with the pore size of 0.6 μm, pore fraction of 0.69 and a diameter of 40–60 μm, where P refers to macroreticular styrene-divinylbenzene copolymer (SDB) and is immobilized in porous silica (SiO2) particles with the content of 17–18 wt% in SiO2-P [Citation28]. IsoHexyl-BTP/SiO2-P was prepared by impregnating 5.0 g isoHexyl-BTP into 10.0 g SiO2-P under reduced pressure with the isoHexyl-BTP content of 33 wt% in the adsorbent. The details of the synthesis procedures were described in previous study with isoHexyl-BTP replacing nBu-BTP [Citation28]. The chemical structure of isoHexyl-BTP and the SEM image of isoHexyl-BTP/SiO2-P adsorbent are shown in (a) and 1(b), respectively.
2.2. Batch adsorption experiment
The adsorption properties of isoHexyl-BTP/SiO2-P adsorbent towards Ln(III) were tested by batch adsorption experiment. 0.10 g isoHexyl-BTP/SiO2-P was combined with 5.0 mL of aqueous solution in a glass vial. The vial was shaken mechanically at 120 rpm at 25 ± 1 °C for a predesigned time. Then, the aqueous phase was filtrated through G5 sand core funnel with the pore size of 1.5–2.5 µm under reduced pressure. The concentrations of Ln(III) in the aqueous phase before and after adsorption were determined by inductively coupled plasma-atomic emission spectrometry (ICP-AES: Shimadzu ICPS-7510).
The distribution coefficient Kd (mL·g−1) and adsorption amount q (mmol·g−1) are calculated by EquationEquations (1)(1)
(1) and Equation(2)
(2)
(2) , respectively:
(1)
(1)
(2)
(2) where Co (mM) and Ce (mM) denote the concentration of metal in the aqueous phase before and after adsorption, respectively. V (mL) and W (g) indicate the volume of aqueous phase and the mass of dry isoHexyl-BTP/SiO2-P adsorbent, respectively.
2.3. Acid–base titration and ion chromatography analysis
To evaluate whether H+ and NO3− directly participated in the adsorption, the concentrations of H+ and NO3− before and after adsorption were measured. To measure H+ concentration, an auto-titration system (Mettler Toledo Titration Excellence T50) with the electrode of Mettler Toledo DGi1111-SC was used. An amount of 40 mL of diluted sample solution was added to the titration reactor and the titration was performed with 0.1 M NaOH standard solution to neutralize the H+ in the sample. For the same sample, at least two tests were performed and averaged.
To determine NO3− concentration, an ion chromatography (Metrohm) equipped with a 818 IC pump, two 833 IC liquid handling units, a 819 IC detector, a 820 IC separation center, 830 IC interface and a 6.1006.530 METROSEP SUPP 5–250 mm column were used. The sample volume for test was 20 μL. A solution containing 3.2 mM Na2CO3 and 1.0 mM NaHCO3 was used as an eluent with the flow rate of 0.7 ml/min. At least two tests were performed and averaged for the same sample.
2.4. EXAFS measurements and analysis
EXAFS is highly element selective by excluding the undesirable matrix effect in the system, which make it a very powerful and effective tool for investing a small field for target element to probe the chemical environment. To analyze the H+ or NO3− ionic strength effect on the hydration status of Dy(III) in solution during the adsorption, Dy(NO3)3·5H2O was dissolved in three typical liquid solution, i.e., pure water, 3 M HNO3, 0.01 M HNO3–0.99 M NaNO3, respectively, with Dy(III) concentration 0.1 M. Dy(III) content was about 1.6 wt% in the solution and was high enough for EXAFS measurement in fluorescence mode. The solution samples were transferred to polyethylene self-sealing bags for EXAFS measurements.
To study the coordination chemistry of Ln(III) adsorbed onto isoHexyl-BTP/SiO2-P adsorbent, the isoHexyl-BTP/SiO2-P samples loading Sm(III), Eu(III), Gd(III), Dy(III), respectively, were prepared through batch adsorption experiment. 0.4 g adsorbent was combined with a measured volume of 20 mL aqueous solution with Ln(III) concentration of 6 mM in a 40 mL glass vial. The vial was shaken mechanically at 120 rpm at 25 °C for 48 h to achieve adsorption equilibrium. Then, the aqueous phase was filtrated through G5 sand core funnel under reduced pressure to remove the solution. Then, the isoHexyl-BTP/SiO2-P adsorbent which had adsorbed Ln(III) was put into the silica gel drier for one day before test and ground into tiny uniform particles and spread on test adhesive tape for EXAFS measurement.
Sm L3, Eu L3, Gd L3 and Dy L3 EXAFS measurements were performed at room temperature in fluorescence mode at beam line BL14W1 of the Shanghai Synchrotron Radiation Facility (SSRF), China. The station was operated with a double crystal Si(111) monochromator. During the measurement, the synchrotron was operated at energy of 3.5 GeV and a current between 150 and 210 mA. Sm, Eu, Gd L3-edge spectra were calibrated with a Fe foil while Dy L3-edge spectrum was calibrated with a Co foil, respectively. A high purity Ge solid-state detector was used to collect the fluorescence signals.
The obtained spectra data were analyzed with the IFEFFIT 8.5 software code [Citation29]. The EXAFS threshold energies, E0, were defined at 6716, 6977, 7243 and 7790 eV at Sm-, Eu-, Gd- and Dy-L3 edges, respectively. The EXAFS spectra were pre-edge background removed, normalized, smoothed and Fourier transformed (FT) with the k range of 2.6–10 Å for the liquid samples and 2.6–11.5 Å for the Ln(III) adsorbed onto isoHexyl-BTP/SiO2-P solid samples using the IFEFFIT-Athena software code. Then, the data were further performed by least squares fit to the EXAFS equation in R-space using the IFEFFIT-Artemis software code. Metric parameters describing the coordination structure, i.e., coordination numbers (N), interatomic distances (R), EXAFS Debye–Waller factors (σ2), and relative shifts in threshold energy (ΔE0) were obtained. The amplitude reduction factor S02 was held constant at unity during fits. The data were disposed without phase shift correction.
3. Results and discussion
3.1. The effect of H+ and NO3− on the adsorption
3.1.1. Adsorption behavior in different HNO3 and NaNO3 concentration solution
To study the effect of nitric acid concentration or nitrate concentration on the adsorption of isoHexyl-BTP/SiO2-P towards Ln(III), batch adsorption experiments were performed in HNO3 system with the HNO3 concentration changing in 0.01–4 M and in 0.01 M HNO3–NaNO3 system with the NaNO3 concentration changing in 0.09–3.99 M, respectively.
(a) shows Kd of Ln(III) towards isoHexyl-BTP/SiO2-P adsorbent as a function of initial HNO3 concentration. As can be seen, it showed no obvious adsorption towards Ln(III) in ≤ 1 M HNO3. When the nitric acid concentration increased in the range of 1–4 M, isoHexyl-BTP/SiO2-P adsorption ability towards the middle and heavy Ln(III) increased significantly. Furthermore, the adsorption ability increased as the atomic number increased with the order of Dy(III) > Gd(III) > Eu(III) > Sm(III) > Y(III), while isoHexyl-BTP/SiO2-P showed only weak adsorption towards light Ln(III), such as La(III), Ce(III) and Nd(III). (b) shows Kd values of isoHexyl-BTP/SiO2-P adsorbent towards Ln(III) in 0.01 M HNO3–NaNO3 system. In this system, isoHexyl-BTP/SiO2-P adsorbent exhibited very weak adsorption towards Gd(III) and Dy(III) as NO3− concentration increase, but showed almost no adsorption towards Ln(III) lighter than Gd(III). The Kd values were all less than 5 mL·g−1.
Figure 2. Effect of HNO3 concentration (a) and NaNO3 concentration (b) on the distribution coefficients of Ln(III) by isoHexyl-BTP/SiO2-P (adsorbent: 0.1 g, solution: 5 mL, metal: 1 mM, temperature: 25 °C, contact time: 24 h, shaking speed: 120 rpm).
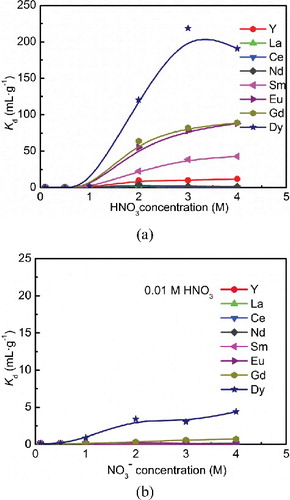
Comparing (a) and 2(b), it is concluded that H+ and NO3− played key effect on the adsorption behavior. To explain the phenomenon more clearly, two aspects were further taken into consideration, i.e., (a) If H+ or NO3− ionic strength would have an effect on the Ln(III) hydration state in the solution, e.g., the coordination numbers of water and NO3−, different hydration state may have effect on the adsorption behavior. (b) If H+ and NO3− would directly participate in the coordination reaction during the adsorption process. If so, how many H+, NO3− would take part in during the adsorption. To answer the questions, two experiments, i.e., EXAFS study of Dy(III) hydration state in three different solution systems and direct evaluation of the roles of H+ and NO3− in the adsorption, were designed in the following work.
3.1.2. EXAFS study of Dy(III) hydration state in three different systems
To understand the different adsorption behavior of isoHexyl-BTP/SiO2-P towards middle and heavy Ln(III) in nitric acid and nitrate systems more clearly, Dy(III) hydration state in three typical solution systems, i.e., water, 3 M HNO3 and 0.01 M HNO3 - 0.99 M NaNO3 systems with the target element Dy(III) concentration of 0.1 M, were studied respectively using EXAFS.
shows the experimental k2-weighted L3-edges EXAFS spectra of Dy(III) hydration state in the above three systems and their corresponding FTs. The EXAFS oscillations in 3 M HNO3 or in 0.01 M HNO3–0.99 M NaNO3 solution system appeared to be almost identical with those in water. It has been reported that Ln(III) ions form a symmetrical complex with nitrate ions, such as [Ln(NO3)n·mH2O]−(3-n) (n = 0–3, m ≤ 9) with bidentate in nitrate containing solution[Citation4], [Citation23,Citation24] and these bidentate-coordinate nitrate ions make the EXAFS oscillation more intricate, especially at higher k range due to the strong feature of multiple scattering (MS). The main peaks at ∼2 Å were expected to be the mixture of two different O shells, i.e., Oco(NO3−) and O(H2O). In principle, these two O shells are indistinguishable if their difference is below the resolution limit. However, small peaks which may be attributed by the distal O atoms (Odist(NO3−)) and the MS of nitrate ions were observed at ∼3 Å and ∼3.8 Å [Citation18], and in consequence, we can estimate the structural parameters for Oco(NO3−) and N atoms of nitrate ions by correlating these parameters with those of Odist(NO3−) and MS paths in the fitting procedure. Dy(III) corresponding to nitrate crystal Dy(NO3)3•6H2O with Cartesian coordinates reported in reference [Citation30] with the ICSD number of 154430 was used for the feff calculation. The Dy(III) solution sample data were modelled using four coordination shells in the fits, i.e., Oco(NO3−), O(H2O), N(NO3−), Odist(NO3−) in the R-range 1.2–5 Å. The curve fits took account of all possible single scattering Dy→Oco(NO3−)→Dy, Dy→O(H2O)→Dy, Dy→N(NO3−)→Dy, Dy→Odist(NO3−)→Dy and MS paths, e.g., Dy→O(H2O)→N(NO3−)→Dy, Dy→Oco(NO3−)→N(NO3−)→Dy.
Figure 3. k2-Weighted L3-edge EXAFS (left) and their corresponding Fourier transform (FT) (right) data for Dy(III) hydration states in three different systems: (a): water, (b): 3 M HNO3, (c): 0.01 M HNO3 – 0.99 M NaNO3 ((Dy(III)): 0.1 M, experimental data are shown as dot, smoothed data are shown as lines and fit curves as open circles.).
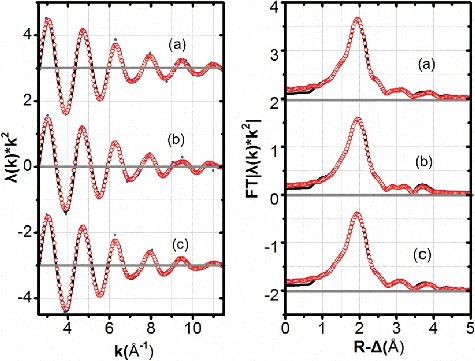
The EXAFS structural parameters obtained from the curve fitting are given in . The parameters obtained in water, 3 M HNO3 or 0.01 M HNO3–0.99 M NaNO3 solution systems are almost the same. The coordination number of NO3− and H2O are about 2 and 6, respectively. While for all samples, Debye–Waller factor of Oco(NO3−) shell is larger than that of other shells, the reason is not cleat at present. According to the results above, the most possible complex of Dy(III) in above three systems are supposed to be [Dy(NO3)2·6H2O]+. The results are consistent with those reported in the references [Citation4,Citation23,Citation24]. This suggests that although higher NO3−, H+ and Na+ concentration in the solution may reduce the activity of H2O, the Dy(III) ions keep their hydration state unchanged at least in their primary coordination sphere in the above three solution systems. Dy(III) hydration states in 3 M HNO3 system or in 0.01 M HNO3–0.99 M NaNO3 system are basically the same and are not supposed as the key factors on the difference of the adsorption behavior of isoHexyl-BTP/SiO2-P towards Dy(III) in 3 M HNO3 system or in 0.01 M HNO3-0.99 M NaNO3 system as shown in
Table 1. Summary of EXAFS structural parameters of Dy(III) hydration states in three solution systems.
3.1.3. Evaluation of the role of H+ and NO3− in the adsorption
To elaborate whether H+ and NO3− directly participate in the adsorption behavior of isoHexyl-BTP/SiO2-P towards Ln(III), the concentrations of Dy3+, H+ and NO3− in the solution before and after the adsorption were analyzed. During the experiment, the added isoHexyl-BTP/SiO2-P adsorbent amount increased gently, i.e. 0.05g, 0.1g, 0.15g, 0.20g, while the aqueous volume kept constant, i.e., 2.5 mL with Dy(III) concentration of 20 mM in 3 M HNO3 in the initial solution. If H+ or NO3− directly participates in the adsorption process, their concentrations will accordingly decrease.
(a) shows the adsorbed amount of Dy(III) versus the added amount of isoHexyl/BTP/SiO2-P. As seen, the adsorbed amount of Dy(III) increased linearly with the added isoHexyl-BTP/SiO2-P with a very small intercept of −0.0002, which means the adsorbed amount of Dy(III) nearly proportionally increased with the adsorbent increase. The slope was 0.29 whose reciprocal is 3.4, indicating it takes about 3.4 molecule of isoHexyl/BTP/SiO2-P to uptake 1 molecule of Dy(III), and the ratio does not change with the adsorbent increase in this experiment. (b) shows the adsorbed amount of H+ or NO3− versus adsorbed amount of Dy(III), where the adsorbed amount of H+ and NO3− also nearly proportionally increased with the adsorbed amount of Dy(III) with high correlation coefficients of over 0.99. The slopes of the adsorbed amount of H+ and NO3− versus the adsorbed amount of Dy(III) were 3.1 and 6.2, respectively, which suggests that H+ and NO3− would directly participate in the adsorption process and thus can explain the results shown in . According to the results above, the adsorption reaction of isoHexyl-BTP/SiO2-P towards middle and heavy Ln(III) is speculated as EquationEquation (3)(3)
(3) , and similar results have been reported in solvent extraction systems[Citation5].
(3)
(3)
Figure 4. Evaluation direct role play of H+ and NO3− in the adsorption (a) adsorbed amount of Dy(III) versus added amount of isoHexyl/BTP/SiO2-P. (b) Adsorbed amount of H+ or NO3− versus adsorbed amount of Dy(III) (solution: 2.5 mL, acid: 3 M HNO3, (Dy): 20 mM, temperature: 25 °C, contact time: 48 h, shaking speed: 120 rpm).
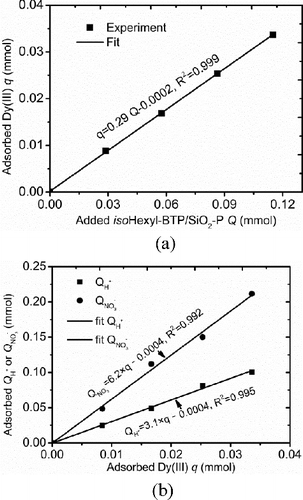
3.2. Adsorption selectivity and coordination chemistry
3.2.1. Adsorption towards Ln(III) in 3 M HNO3
As shown in (a), isoHexyl-BTP/SiO2-P exhibited different adsorption affinities towards Ln(III) in concentrated nitric acid solution in Ln(III) coexistence state. Furthermore, the adsorption behavior of isoHexyl-BTP/SiO2-P towards Ln(III) in single element system was performed in 3 M HNO3 solution at 25 °C for 24 h contact time. The results are shown in . IsoHexyl-BTP/SiO2-P showed almost no adsorption towards light Ln(III), such as La(III), Ce(III), Pr(III), Nd(III), while towards the middle and heavy Ln(III), the adsorption ability increased quickly as the atomic number increased until Er(III) with the Kd values reaching to thousands, then the adsorption ability decreased as the atomic number increased further from Er(III) to Lu(III), but still kept the Kd values in a high level of over hundreds. The reason for this behavior was explained by the size fitting of the isoHexyl-BTP/SiO2-P cavity being preferable for the intermediate-to-heavy Ln(III) in the reference [Citation13]. Compared with (a), another fact was that Kd values in single element system were higher than those in coexistence system due to the competition adsorption among Ln(III) when elements coexisted.
3.2.2. EXAFS study of Ln(III) adsorbed onto isoHexyl-BTP/SiO2-P samples
To study the different adsorption affinities of isoHexyl-BTP/SiO2-P towards different Ln(III) shown in and the adsorption coordination chemistry between Ln(III) and isoHexyl-BTP/SiO2-P, EXAFS was used to analyze the solid samples of Ln(III) adsorbed onto isoHexyl-BTP/SiO2-P adsorbent. Four typical middle and heavy Ln(III) elements, i.e., Sm(III), Eu(III), Gd(III) and Dy(III), were chosen.
The k2-weighted EXAFS of Ln(III) complexes adsorbed on isoHexyl-BTP/SiO2-P are shown in . A 55-atom cluster (1 central Ce(III) ion and 54 C and N atoms from three BTP ligands, excluding the rest groups on the triazine rings) having Cartesian coordinates for the Ce-complex reported in reference [Citation26] with the CCDC number of 161598 was used for the feff calculation. The central metal ion Ce and the atomic number were changed into the corresponding elements, i.e., Sm, Eu, Gd, Dy and 62, 63, 64, 66, respectively, in feff.inp during the data process. The Ln(III) complexes adsorbed onto isoHexyl-BTP/SiO2-P data were modelled in the fitting in a manner analogous to that of references [Citation20–22] using four coordination shells in the R-range of 1.2–5.5 Å. The first shell is 9 nearest Npy and Ntz atoms directly bound to the Ln(III) ions, where Npy and Ntz atoms refers to the N atoms in pyridine functional groups and in 1,2,4-triazine functional groups, respectively. The second and third shells of 18 C2/N2 atoms each are designated as C2/N2 and C3/N3, respectively. A fourth, the most distant shell of 9 C atoms is corresponding to the C atom located para to the ligating N atoms. Three significant three-legged multiple-scattering paths (Ln→N1→C2/N2→Ln, Ln→C2/N2→C3/N3→Ln, and Ln→N1→C3/N3→Ln paths) were also included in the fits with their effective path lengths correlated to single scattering distances involved. A global ΔE0 was used while σ2 values were varied.
Figure 6. k2-Weighted L3-edge EXAFS (experimental data at top, interpolative smoothing data with the iterations number iterations 10 shifted along the y-axis for clarity at bottom) and their corresponding Fourier transform (FT) data for Sm-BTP3 and Gd-BTP3 (left) and Eu-BTP3 and Dy-BTP3 (right). (Experimental data are shown as lines and fit curves as open/filled circles.)
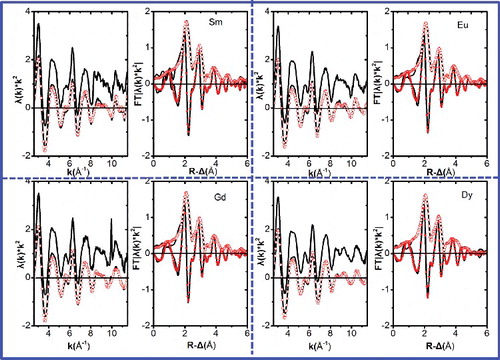
The fitting results of the spectra are listed in . The obtained R values met well with those in the references [Citation20–22], which further proves correctness of EquationEquation (3)(3)
(3) that three moles of isoHexyl-BTP/SiO2-P uptake one mole of middle and heavy Ln(III) in the experiment. The trend of R-values between different Ln(III) samples followed the tendency that as the atomic number increased, the R-values of the corresponding elements decreased. Further comparing the difference between the Ln–N distance R and the Ln(III) ionic radius r (CN = 9) [Citation19], i.e., R r (CN = 9), it also decreases in a small range as the atomic number increases, i.e., Sm > Eu > Gd > Dy. If this difference, i.e., Rr (CN = 9) between bond distance and ionic radius, is considered to be a measure for the bond strength or covalence, then we can conclude that the covalence of the metal cation–N bond increases as the atomic number increases, i.e., Sm < Eu < Gd < Dy [Citation20]. To some extent, it explains the increased adsorption affinity of isoHexyl-BTP/SiO2-P towards Ln(III) as the atomic number increases. As in some samples (especially in Gd), the distance for the forth shell (C4) is shorter than that expected from the ligand structure. The reason may be that during the EXAFS data process, as the lower coordination layers have effect on the higher ones, especially the coupling effect between low coordination layers, so the obtained information of higher layers may be not as exact as the lower ones.
Table 2. Ln(III) absorbed onto isoHexyl-BTP/SiO2-P complexes parameters from
4. Conclusions
In batch adsorption experiments, isoHexyl-BTP/SiO2-P showed adsorption towards middle-heavy Ln(III) in concentrated nitric acid solution (>1 M) and the adsorption ability increased as the atomic number increased, but it showed almost no adsorption towards Ln(III) in 0.01 M HNO3–NaNO3 system. EXAFS, ICP-AES, acid-base titration and IC analysis proved that H+ and NO3− directly participated in the adsorption process and Ln(isoHexyl-BTP/SiO2-P)3(NO3)3·3HNO3 was supposed as the main product for middle and heavy Ln(III) adsorbed on isoHexyl-BTP/SiO2-P. Moreover, it was found that both the metal–N distance R and the difference between the metal–N distance R and the metal cation radius r (N = 9), i.e., Rr (N = 9), decreased as the atomic number increased, which may be as evidence that the covalence of the metal cation–N bond increased as the atomic number increased. This to some extent can explain the adsorption ability of isoHexyl-BTP/SiO2-P adsorbent towards Ln(III) increased as the atomic number increased.
Acknowledgements
This work was supported by the National Natural Science Foundation under Grant No. 91126006, No.11305102; the Ministry of Education of Specialized Research Fund for the Doctoral Program of Higher Education under Grant No. 20130073110046. The authors wish to thank the beam line BL14W1 of the Shanghai Synchrotron Radiation Facility (SSRF), China where the EXAFS experiments were carried out and Bruce Ravel et al. for free offering the ifeffit 8.5 software code.
Disclosure statement
No potential conflict of interest was reported by the authors.
Additional information
Funding
References
- Magill J, Berthou V, Haas D, et al. Impact limits of partitioning and transmutation scenarios on the radiotoxicity of actinides in radioactive waste. Nucl Energy –Lond. 2003;42(5):263–278.
- Malmbeck R, Nourry C, Ougier M, et al. Advanced fuel cycle options. Energy Procedia. 2011;7:93–102.
- Dudwadkar NL, Tripathi SC, Gandhi PM. Studies on the partitioning of actinides from high level liquid waste solution employing supported liquid membrane with Cyanex-923 as carrier. J Radioanal Nucl Chem. 2012;295(2):1009–1014.
- Lan JH, Shi WQ, Yuan LY, et al. Thermodynamic study on the complexation of Am(III) and Eu(III) with tetradentate nitrogen ligands: a probe of complex species and reactions in aqueous solution. J Phys Chem A. 2012;116(1):504–511.
- Panak P J, Geist A. Complexation and extraction of trivalent actinides and lanthanides by triazinylpyridine N-donor ligands. Chem Rev. 2013;113(2):1199–1236.
- Clément H, Guillaneux D, Berthon L, et al. SANEX-BTP process development studies. J Nucl Sci Technol Sup. 2002;3:309–312.
- Kolarik Z, Müllich U, Gassner F. Selective extraction of Am(III) Over Eu(III) By 2,6-Ditriazolyl- and 2,6-Ditriazinylpyridines. Solv Extr Ion Exch. 1999;17(1):23–32.
- Wei YZ, Sabharwal KN, Kumagai M, et al. Preparation of novel silica-based nitrogen donor extraction resins and their adsorption performance for trivalent americium and lanthanide. J Nucl Sci Technol. 2000;37:1108–1110.
- Ning SY, Wang XP, Liu RQ, et al. Evaluation of Me2-CA-BTP/SiO2-P adsorbent for the separation of minor actinides from simulated HLLW. J Radioanal Nucl Chem. 2015;303(3):2011–2017.
- Liu RQ, Ning SY, Wang XP, et al. Adsorption behavior of actinides and some typical fission products by silica/polymer-based isoHex-BTP adsorbent from nitric acid solution. J Radioanal Nucl Chem. 2015;303(1):681–691.
- Wang XP, Ning SY, Liu RQ, et al. Stability of isoHex-BTP SiO2-P adsorbent against acidic hydrolysis and γ-irradiation. Sci China Chem. 2014;57(11):1464–1469.
- Wei YZ, Liu RQ, Wu Y, et al. Chromatographic separation of actinides and fission products. Energy Procedia. 2013;39:110–119.
- Liu RQ, Wei YZ, Xu YL, et al. Evaluation study on properties of isohexyl-BTP/SiO2-P resin for direct separation of trivalent minor actinides from HLLW. J Radioanal Nucl Chem. 2012;292(2):537–544.
- Matsumura T, Matsumura K, Morita Y, et al. Separation of trivalent minor actinides from fission products using single R-BTP column extraction chromatography. J Nucl Sci Technol. 2011;48:855–858.
- Usuda S, Wei YZ, Xu YL, et al. Development of a simplified separation process of trivalent minor actinides from fission products using novel R-BTP/SiO2-P adsorbents. J Nucl Sci Technol. 2012;49:334–342.
- Xu YL, Kim SY, Ito T, et al. Adsorption behavior of trivalent americium and rare earth ions onto a macroporous silica-based isobutyl-BTP/SiO2-P adsorbent in nitric acid solution. J Radioanal Nucl Chem. 2014;299:149–145.
- Trumm S, Geist A, Panak PJ, et al. An improved hydrolytically-stable bis-triazinyl-pyridine (BTP) for selective actinide extraction. Solv Extr Ion Exch. 2011;29(2):213–229.
- Ikeda A, Yaita T, Okamoto Y, et al. Extended X-ray absorption fine structure investigation of adsorption and separation phenomena of metal ions in organic resin. Anal Chem. 2007;79:8016–8023.
- Banik NL, Denecke MA, Geist A, et al. 2,6-Bis(5,6-dipropyl-1,2,4-triazin-3-yl)-pyridine: Structures of An(III) and Ln(III) 1:3 complexes and selectivity. Inorg Chem Commun. 2013;29:172–174.
- Denecke MA, Panak PJ, Burdet F, et al. A comparative spectroscopic study of U(III)/Am(III) and Ln(III) complexed with N-donor ligands. Cr Chim. 2007;10(10–11):872–882.
- Denecke M. Actinide speciation using X-ray absorption fine structure spectroscopy. Coordin Chem Rev. 2006;250(7–8):730–754.
- Denecke MA, Rossberg A, Panak PJ, et al. Characterization and comparison of Cm (III) and Eu (III) complexed with 2, 6-di (5, 6-dipropyl-1, 2, 4-triazin-3-yl) pyridine using EXAFS, TRFLS, and quantum-chemical Methods. Inorg Chem. 2005;44:8418–8425.
- Yaita T, Ito D, Tachimori S. La NMR relaxation and chemical shift studies in the aqueous nitrate and chloride solutions. J Phys Chem B. 1998;102:3886–3891.
- Patrick JB, William DHJ. Europium(III) luminescence excitation spectroscopy: inner-sphere complexation of europium(III) by chloride, thiocyanate, and nitrate ions. Inorg Chem. 1983;22:536–540.
- Boucher C, Drew MGB, Giddings P, et al. 12-coordinate complexes formed by the early lanthanide metals with 2,6-bis(-1,2,4-triazin-3-yl)-pyridine. Inorg Chem Commun. 2002;5:596–599.
- Iveson PB, Rivière C, Nierlich M, et al. Selective complexation of uranium(III) over cerium(III) by 2,6-bis(5,6-dialkyl-1,2,4-triazin-3-yl)pyridines: 1H NMR and X-ray crystallography studies. Chem Commun. 2001;16:1512–1513.
- Ning SY, Zou Q, Wang XP, et al. Evaluation study on silica/polymer-based CA-BTP adsorbent for the separation of minor actinides from simulated high-level liquid wastes. J Radioanal Nucl Chem. 2016;307(2):993–999.
- Wei YZ, Hoshi H, Kumagai M, et al. Separation of Am(III) and Cm(III) from trivalent lanthanides by 2,6-bistriazinylpyridine extraction chromatography for radioactive waste management. J Alloys Comp. 2004;374(1–2):447–450.
- Bruce R. ATHENA User's Guide. Available from: http://cars9.uchicago.edu/
- Gao SL, Ma HR, Yang ZP. Crystal structure of dysprosium(III) nitrate hexahydrate. J. Northwest Univ. 1990;20(2):53–58.