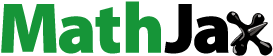
ABSTRACT
Neutron nuclear data on 10 isotopes of platinum have been evaluated for the next version of Japanese Evaluated Nuclear Data Library general-purpose file in the energy region from 10−5 eV to 20 MeV. Resolved resonance parameters of naturally occurring isotopes were taken from a compilation work, while unresolved resonance parameters were obtained by fitting to the total and capture cross sections calculated from nuclear models. A statistical model code was applied to evaluate cross sections above the resolved resonance region. Compound, pre-equilibrium and direct-reaction processes were considered for cross-section calculation. Coupled-channel optical model parameters were employed for the interaction between neutrons and nuclei. Giant-dipole and pygmy resonance parameters for E1 γ-ray transition from platinum isotopes were determined so as to reproduce measured γ-ray spectrum. The present results reproduce experimental data very well. The evaluated data are compiled into Evaluated Nuclear Data File formatted data files.
1. Introduction
Nuclear data are required for nuclear science and engineering. Especially, neutron-induced reaction data are important for nuclear energy applications. Japanese Evaluated Nuclear Data Libraries (JENDLs) have been developed by the Japan Atomic Energy Agency (JAEA) since 1977. The fourth version of the general-purpose file, JENDL-4.0, was released [Citation1] in 2010. JENDL-4.0 contains neutron-induced reaction data for 406 nuclides in the atomic number of Z = 1–100. However, the data for 11 elements have never been evaluated due to the low priority for nuclear energy applications. Platinum is an element of which data are missing in JENDL-4.0. Recently, activation cross sections of various elements have been needed for the decommissioning of nuclear facilities. In addition to major elements, we have to pay attention to minor elements which could be contained as impurities in structural materials, since their radioactivity might be high. Hence, the platinum data should be evaluated in order to make JENDL a complete library.
The present work was undertaken to newly evaluate the data on platinum isotopes by considering the latest knowledge on experimental and theoretical nuclear physics. Evaluated are the total, elastic and inelastic scattering, (n, γ), (n, p), (n, d), (n, t), (n, 3He), (n, α), (n, np), (n, nd), (n, nα), (n, 2n), (n, 3n), (n, 2np) reaction cross sections, the angular distributions of elastically and inelastically scattered neutrons and the energy distributions of emitted particles and γ-rays in the energy region from 10−5 eV to 20 MeV. The Q-values of the reactions were calculated from the mass table AME2012 [Citation2], and are listed in , together with abundances [Citation3] and half-lives [Citation4].
Table 1. Isotopic abundances and reaction Q-values of platinum isotopes.
The paper presents how the evaluation was performed. Section 2 deals with the resonance region. In Section 3, the computational methods and procedures above the resonance region are described. Comparisons of the evaluated results with the experimental and existing evaluated data are made in Section 4. Finally, Section 5 summarises the conclusion.
2. Resonance region
The resolved and unresolved resonance regions are listed in for each target nucleus. In principle, resolved resonance parameters (RRPs) are obtained from the analyses of experimental data. RRPs are available for 190, 192, 194, 195, 196, 198Pt, although the parameters were quite old and no new measurements have been reported. In the present work, the RRPs for these nuclei were taken from the compilation work of Mughabghab [Citation5]. The neutron widths for the negative resonances of 190, 192, 194Pt were adjusted so as to reproduce the thermal capture cross sections recommended by Mughabghab, while those of 195, 196, 198Pt were modified by considering the data measured by Massarczyk et al. [Citation6] for 195Pt and by Farina Arboccò et al. [Citation7] for 196, 198Pt. Concerning 189, 191, 193, 197Pt for which RRPs were unavailable, constant and 1/v energy dependencies were assumed for the elastic scattering and capture cross sections, respectively. Normalisation of these cross sections was done at thermal energy. The thermal scattering cross section was calculated as 4πR′ 2, where R′ expresses the scattering radius that can be estimated from nuclear model calculations in the higher energy region. On the other hand, the thermal capture cross sections of these nuclei were estimated from a simplified formula used in the preceding work on antimony [Citation8]. This formula gives rough estimates of thermal capture cross sections by using theoretical s-wave γ-ray strength functions. It is used only when no other options are available.
Table 2. Resolved and unresolved resonance regions.
The unresolved resonance parameters (URPs) were determined with the ASREP code [Citation9] by fitting to the total and capture cross sections evaluated with the nuclear models which will be described in the succeeding section. The URPs obtained are used only for self-shielding calculations, since the pointwise cross sections are given in the evaluated data files. As an example, the average cross sections of 197Pt reconstructed from the URPs are compared in with the total and capture cross sections calculated from the nuclear models. In the parameter fitting with the ASREP code, 10% uncertainties in the nuclear model calculations were assumed. The reconstructed cross sections reproduce the nuclear model calculations very well.
3. Computational methods and procedures above resonance region
3.1. Nuclear models
The CCONE code (Version 0.8.4) [Citation10,Citation11] was used for calculating the neutron-induced reaction cross sections of platinum isotopes. The code is based on the spherical and coupled-channel optical models, the two-component exciton pre-equilibrium model, the distorted-wave Born approximation, and the multistep statistical model. In order to simulate the direct and semi-direct effects on the radiative capture reaction, the pre-equilibrium capture was considered by using the γ-ray emission rate of Akkermans and Gruppelaar [Citation12] extended to the two-component exciton pre-equilibrium model.
3.2. Parameter determination
3.2.1. Optical model potentials
As for neutrons, we employed the global optical model parameters obtained by Kunieda et al. [Citation13] using the coupled-channel method based on the rigid rotor model [Citation14]. The potential forms are the same as those used in the previous work [Citation15]. From the neutron potentials, transmission coefficients are obtained together with total cross sections, shape-elastic scattering cross sections, and the direct-reaction components of inelastic scattering cross sections for the CCONE calculation. The parameter values are listed in , and the coupling schemes and deformation parameters are given in . The deformation parameters for 194, 196, 198Pt were taken from the work of Deason et al. [Citation16], while those for the remaining nuclei were based on the finite-range droplet model (FRDM) [Citation17]. The calculated total cross section of elemental Pt is shown in together with experimental data and the spherical optical model calculations using the parameters of Koning and Delaroche [Citation18]. In order to give a better fit to the experimental data, the value of WDISPD was adjusted, while the rest of the parameters remain unchanged from the original ones [Citation13]. It is found from that the present calculations reproduce the measured data better than those using the parameters of Koning and Delaroche.
Table 3. Optical model parameters for neutrons.
Table 4. Coupling schemes used in the interaction between neutron and target.
Concerning charged particles, we utilised the spherical parameters of Koning and Delaroche [Citation18] for protons, those of Lohr and Haeberli [Citation19] for deuterons, those of Becchetti and Greenlees [Citation20] for tritons and 3He, and those of Avrigeanu et al. [Citation21] for α-particles. The transmission coefficients, which are required by CCONE to calculate charged-particle emission, were obtained from the charged-particle parameters.
3.2.2. Discrete levels and level density
In the calculation, it was necessary to input the discrete levels and level density parameters for 35 nuclei, i.e. 188 − 199Pt, 188 − 198Ir, and 185 − 196Os. The discrete levels were taken from the reference input parameter library RIPL-3 [Citation22].
Concerning the level density, the composite formula of Gilbert and Cameron [Citation23] was used in the present work. In the region of low excitation energy E, the level density is described by the constant temperature formula ρT, namely
(1)
(1)
where T and E0 are adjustable parameters to fit the formula in measured discrete levels. On the other hand, the a parameter, which characterises the Fermi-gas part of level density ρF, is defined as
(2)
(2)
where Esh is the shell correction energy and γ a damping factor. The damping factor is given by γ = 0.40A− 1/3MeV− 1. The energy U is expressed by E and the pairing energy Δ, i.e. U = E − Δ. In the above equations, the values of a(*) and Δ were taken from the work of Mengoni and Nakajima [Citation24]. The shell correction energy Esh was calculated as the difference between the experimental mass [Citation2] and the theoretical mass [Citation25]. The two parameters T and E0 were determined so as to connect ρF and ρT smoothly at an appropriate matching energy Em. The parameters used in this work are listed in for individual nuclei, together with the energies of the highest discrete levels Ehighestlevel.
Table 5. Level density parameters for each nucleus.
3.2.3. Gamma-ray transition
The γ-ray transmission coefficients are related to the photoabsorption cross sections by the detailed balance. The photoabsorption cross section is usually approximated by various Lorentzian shapes. In the present calculation, the shape was determined by comparing with the γ-ray spectra measured by Voignier et al. [Citation26] at 500 keV. As for the E1 radiation, three terms were needed to reproduce the spectra, i.e. a giant-dipole resonance (GDR), a pygmy resonance, and a correction term, as in the analysis performed by Kim et al. [Citation27]. An enhanced generalised Lorentzian (EGLO) [Citation28] was used for the GDR and the pygmy resonance terms, while the correction term was based on a standard Lorentzian (SLO). The resonance energy (E0), resonance width (Γ0), and peak cross section (σ0) were fixed for all platinum isotopes, and they are given in . The calculated γ-ray spectrum for 194Pt is compared with the data measured by Voignier et al. at an angle of 90° in , where the measurements are multiplied by 4π. Agreement between the calculated and measured shapes is satisfactory. In this comparison, much emphasis is placed on the spectral shape, since the 500-keV capture cross section of Voignier et al. is slightly larger than the present calculation, as seen in the succeeding section. For the other nuclei such as iridium and osmium isotopes, the GDR parameters for EGLO are given [Citation29] by
(3)
(3)
(4)
(4)
(5)
(5)
where Z, A, and N are the atomic number and mass number of a nucleus, and N = A − Z, respectively. The SLO form was used to describe the M1 and E2 radiations for which the parameters were taken from the work of Kopecky and Uhl [Citation30].
Table 6. E1 parameters for platinum isotopes.
In cases where measured capture cross sections are available in the keV region, the γ-ray strength functions are renormalised so that the calculated cross sections reproduce these data. The s-wave γ-ray strength functions are compared in with the values recommended by Mughabghab [Citation5]. In this work, the strength functions for 191, 196, 199Pt were renormalised, whereas those for the rest of nuclei remained unchanged.
Table 7. Gamma-ray strength functions for s-wave neutron in units of 10−4.
3.2.4. Pre-equilibrium parameters
The Version 0.8.4 of CCONE incorporates the pre-equilibrium processes for the (n, γ) and first-step binary reactions such as (n, n′), (n, p), (n, d), (n, t), (n, 3He), and (n, α). In the two-exciton pre-equilibrium formalism, the single-particle state densities for protons and neutrons in a residual nucleus are described [Citation31] by
(6)
(6)
(7)
(7)
respectively. The parameter C0, of which the default value is unity, is changed so that the calculations reproduce measured neutron and proton emission data such as (n, 2n) and (n, p) in the present work. The (n, 2n) reaction is a ternary reaction, which proceeds from (n, n′) by sequential decay in the framework of the multistep statistical model. Hence, the (n, 2n) cross section is sensitive to C0 for the residual nucleus in the (n, n′) reaction. More experimental data are available for (n, 2n) than for (n, n′) in the nuclides presently concerned. In addition to the C0's, the parameters for the knockout and pickup reactions [Citation32] were adjusted for α emission. The pre-equilibrium parameters used are listed in , where C0's are given only for the residual nuclei in the (n, n′) and (n, p) reactions, and are assumed to be unity for those in the other first-step binary reactions.
Table 8. Pre-equilibrium parameters.
4. Comparison with experimental data and other evaluated data in the fast-neutron region
The presently evaluated data are compared with experimental data and other evaluated data. The experimental data are taken from the EXFOR database [Citation33] that is compiled by the international data centres such as the IAEA Nuclear Data Section, the OECD NEA Data Bank, and the National Nuclear Data Center at the Brookhaven National Laboratory. As for the general-purpose evaluated nuclear data, there exist three major libraries, i.e. JENDL-4.0 [Citation1], ENDF/B-VII.1 [Citation34], and JEFF-3.2 [Citation35]. As for platinum, JEFF-3.2 contains the data on 190, 192, 194, 195, 196, 198Pt, while no platinum data are included in JENDL-4.0 and ENDF/B-VII.1. The activation cross-section files JENDL/A-96 [Citation36] and JEFF-3.1/A [Citation37] are also used for comparisons. We do not discuss most of the reactions for which experimental data are unavailable, since it is difficult to judge whether the present evaluation is reasonable without measurements.
The capture cross sections of 192, 194, 195, 196, 198Pt are illustrated in –. Except for 198Pt, the measurements of Koehler and Guber [Citation38] are useful for validating the evaluated data. In this work, the capture cross section of 195Pt was renormalised by considering their data, although those of 192, 194, 196Pt remained unchanged from the original calculations. The present evaluations well reproduce the data of Koehler and Guber for 192, 194, 195, 196Pt. Concerning 192Pt, the JENDL/A-96 evaluation is remarkably larger than the measurements and the other evaluations below 500 keV. As mentioned in Section 3, the 500-keV capture cross section of 194Pt measured by Voignier et al. [Citation26] (122 ± 9 mb) is slightly larger than the present evaluation (105.6 mb). This is why only the shape of their measured spectrum was considered for the determination of the γ-ray strength functions for the platinum isotopes. As for 196Pt, the other evaluations obviously put emphasis on the data measured by Herman et al. [Citation39] in the energy region from 0.5 to 1.3 MeV. An explanation is needed for the 198Pt(n, γ) reaction. Renormalisation of the γ-ray strength function for 199Pt was done so that the calculated Maxwellian-averaged cross section reproduced the cross section measured by Marganiec et al. [Citation40] at kT = 30 keV, as shown in . is illustrated in order to demonstrate that the Maxwellian averaging of 196Pt(n, γ)197Pt can also reproduce the data measured by the same authors. It should be noted that renormalisation of the γ-ray strength function was not done for the latter case. The capture cross section of 190Pt was renormalised so as to reproduce the Maxwellian-averaged cross section measured by Marganiec et al. [Citation41] at kT = 30 keV, as seen in .
– show the (n, 2n) reaction cross sections of the naturally occurring isotopes. Experimental data are available only for 190, 192, 198Pt. Concerning 190Pt, the present evaluation is smaller than the data measured by Luo et al. [Citation42]. The pre-equilibrium parameter C0 for (n, n′) would become very small, i.e. less than 0.5, in order to fit the data of Luo et al. Such a small value is unreasonable by comparing with the values for 192, 198Pt. In this work, further adjustment of the 190Pt(n, 2n) cross section was not performed by considering the facts that the abundance of 190Pt is extremely small and there exist no other measurements. The experimental data on the 192Pt(n, 2n) reaction are well reproduced by the present evaluation, while the JEFF-3.2 evaluation is slightly smaller than the measurements. As for 198Pt, all the evaluations are consistent with most of the measurements around 14 MeV except the one measured by Paul and Clarke [Citation43] (2.77 b ± 50%) which would be outside . However, the data of Bormann et al. [Citation44], which were obtained by the β− counting, are systematically larger than the evaluations in the region from 12 to 16 MeV. The presently evaluated 194, 195, 196Pt(n, 2n) cross sections are almost consistent with the JEFF-3.2 evaluations. The (n, 2n) cross section of elemental Pt is illustrated in , where the data of Frehaut et al. [Citation45] were renormalised by the factor of 1.078 that was derived by Vonach et al. [Citation46]. The present and JEFF-3.2 evaluations are in good agreement with the measurements, while the JEFF-3.1/A evaluation deviates from the former ones above 12 MeV.
The charged-particle emission cross sections are depicted in –. As for the partial 194Pt(n, p) reactions, the present and JEFF-3.1/A evaluations are consistent with the measurements. On the other hand, four evaluations of 195Pt(n, p) are somewhat different from one another, although the measured data are reproduced by the present evaluation. Concerning the 196Pt(n, p)196mIr reaction, the pre-equilibrium parameter C0 for (n, p) was adjusted so as to reproduce the cross sections measured by Filatenkov and Chuvaev [Citation47]. They deduced the cross sections by measuring four γ-rays, i.e. 393.5, 447.1, 521.4, and 647.3 keV with high-energy resolution, while only the 355.9-keV γ-line was used for the determination of the cross section in the other experiments. The data of Filatenkov and Chuvaev were judged most reliable in this work, since it was indicated [Citation47] that part of the 355.9-keV γ-ray peak could come from other reactions. The (n, α) cross sections of 194, 196Pt, which were measured by Filatenkov and Chuvaev [Citation47] and Coleman et al. [Citation48], are reproduced by the present and JEFF-3.1/A evaluations.
5. Concluding remarks
Platinum data had never been considered in the JENDL general-purpose files due to the low priority. The present work was undertaken to make JENDL complete for various applications. The neutron nuclear data on platinum isotopes were evaluated in the energy region from 10−5 eV to 20 MeV. The RRPs of 190, 192, 194, 195, 196, 198Pt were taken from the existing compilation work, since no new measurements were available. In the low-energy region, constant and 1/v energy dependencies were assumed for the elastic scattering and capture cross sections of 189, 191, 193, 197Pt, since there exist no RRPs. The thermal capture cross sections of these nuclei were renormalised by using the simplified formula. The URPs were obtained for self-shielding calculations by fitting to the total and capture cross sections calculated from the nuclear models.
In this work, the statistical model code CCONE was applied to the calculation of fast-neutron cross sections. The neutron transmission coefficients were obtained by the coupled-channel method, together with the total cross sections, the shape-elastic scattering cross sections and the direct-reaction components of the inelastic scattering cross sections. The neutron potentials were found to be reliable by comparing with the measured total cross sections. The GDR and pygmy resonance parameters for platinum isotopes, which were needed to calculate γ-ray emission, were determined so as to reproduce the shape of the γ-ray spectrum for 194Pt measured at 500 keV. The presently evaluated cross sections are in good agreement with measurements, and are much better than the other evaluations such as JEFF-3.2, JEFF-3.1/A, and JENDL/A-96. The evaluated data are compiled into ENDF-formatted data files for the next release of the JENDL general-purpose file.
Acknowledgments
The author would like to thank the members of the Nuclear Data Center, JAEA, for their helpful comments on this work. He is also grateful to Dr M. Ishikawa for reviewing the manuscript.
Disclosure statement
No potential conflict of interest was reported by the author.
References
- Shibata K, Iwamoto O, Nakagawa T, et al. JENDL-4.0: a new library for nuclear science and engineering. J Nucl Sci Technol. 2011;48:1–30.
- Wang M, Audi G, Wapstra AH, et al. The AME2012 atomic mass evaluation (II). Tables, graphs and references. Chinese Phys C. 2012;36:1603–2014.
- Berglund M, Wieser ME. Isotopic compositions of the elements 2009. Pure Appl Chem. 2011;83:397–410.
- Tuli JK. Nuclear wallet cards. 8th ed. Upton (NY): Brookhaven National Laboratory; 2011.
- Mughabghab SF. Atlas of neutron resonances, resonance parameters and thermal cross sections Z = 1–100. Amsterdam: Elsevier; 2006.
- Massarczyk R, Schramm G, Junghans AR et al. Electromagnetic dipole strength up to the neutron separation energy from 196Pt(γ, γ′) and 195Pt(n, γ) reactions. Phys Rev C. 2013;87:044306.
- Farina Arboccò F, Vermaercke P, Smits K et al. Experimental determination of k0, Q0 factors, effective resonance energies and neutron cross-sections for 37 isotopes of interest in NAA. J Radioanal Nucl Chem. 2014;302:655–672.
- Shibata K. Evaluation of neutron nuclear data on antimony isotopes. J Nucl Sci Technol. 2014;51:425–436.
- Kikuchi Y, Nakagawa T, Nakajima Y. ASREP: a computer program for automatic search of unresolved resonance parameters. Tokai: Japan Atomic Energy Research Institute; 1999. Japanese. ( JAERI-Data/Code 99-025).
- Iwamoto O. Development of a comprehensive code for nuclear data evaluation, CCONE, and validation using neutron-induced cross sections for uranium isotopes. J Nucl Sci Technol. 2007;44:687–697.
- Iwamoto O, Iwamoto N, Kunieda S, et al. The CCONE code system and its application to nuclear data evaluation for fission and other reactions. Nucl Data Sheets. 2016;141:259-288.
- Akkermans JM, Gruppelaar H. Analysis of continuum gamma-ray emission in precompound-decay reactions. Phys Lett B. 1985;157:95–100.
- Kunieda S, Chiba S, Shibata K, et al. Coupled-channels optical model analyses of nucleon-induced reactions for medium and heavy nuclei in the energy region from 1 keV to 200 MeV. J Nucl Sci Technol. 2007;44:838–852.
- Tamura T. Analyses of the scattering of nuclear particles by collective nuclei in terms of the coupled-channel calculation. Rev Mod Phys. 1965;37:679–708.
- Shibata K. Evaluation of neutron nuclear data on tantalum isotopes. J Nucl Sci Technol. 2016;53:957–967.
- Deason PT, King CH, Ronningen RM, et al. 194, 196, 198Pt(p, p′) reactions at 35 MeV. Phys Rev C. 1981;23:1414–1433.
- Möller P, Nix JR, Myers WD, et al. Nuclear ground-state masses and deformations. Atom Data Nucl Data Tables 1995;59:185–381.
- Koning AJ, Delaroche JP. Local and global nucleon optical models from 1 keV to 200 MeV. Nucl Phys A. 2003;713:231–310.
- Lohr JM, Haeberli W. Elastic scattering of 9–13 MeV vector polarized deuterons. Nucl Phys A. 1974;232:381–397.
- Becchetti FD, Greenlees GW. A general set of 3He and triton optical-model potentials for A > 40, E < 40 MeV. In: Barshall HH, Haeberli W, editors. Proceedings of the Third International Symposium on Polarization Phenomena in Nuclear Reactions; 1970 August 31–September 4; Madison (WI): University of Wisconsin Press; 1971. p. 682–683.
- Avrigeanu V, Hodgson PE, Avrigeanu M. Global optical potentials for emitted alpha particles. Phys Rev C. 1994;49:2136–2141.
- Capote R, Herman M, Obložinský P, et al. RIPL–reference input parameter library for calculations of nuclear reactions and nuclear data evaluations. Nucl Data Sheets. 2009;110:3107–3214.
- Gilbert A, Cameron AGW. A composite nuclear-level density formula with shell corrections. Can J Phys. 1965;43:1446–1496.
- Mengoni A, Nakajima Y. Fermi-gas model parametrization of nuclear level density. J Nucl Sci Technol. 1994;31:151–162.
- Myers WD, Swiatecki WJ. Nuclear masses and deformations. Nucl Phys. 1966;81:1–60.
- Voignier J, Joly S, Greiner G. Capture cross sections and gamma-ray spectra from the interaction of 0.5- to 3.0-MeV neutrons with nuclei in the mass range A = 45 to 238, Nucl Sci Eng. 1992;112:87–94.
- Kim HI, Yi MJ, Lee YO. Evaluation of neutron capture gamma-ray spectra for 89Y, 93Nb, 127I, 133Cs, 141Pr, 197Au, natTl, and 209Bi, J Nucl Sci Technol. 2007;44:1117–1125.
- Kopecky J, Uhl M, Chrien RE. Radiative strength in the compound nucleus 157Gd. Phys Rev C. 1993;47:312–322.
- Kopecky J. Gamma-ray strength functions. Vienna: International Atomic Energy Agency; 1998. ( IAEA-TECDOC-1034).
- Kopecky J, Uhl M. Test of gamma-ray strength functions in nuclear model calculations. Phys Rev C. 1990;41:1941–1955.
- Koning AJ, Duijvestijn MC. A global pre-equilibrium analysis from 7 to 200 MeV based on the optical model potential. Nucl Phys A. 2004;744:15–76.
- Kalbach C. The Griffin model, complex particles and direct reactions. Z Phys A. 1977;283:401–411.
- Otuka N, Dupont E, Semkova V, et al. Towards a more complete and accurate experimental nuclear reaction data library (EXFOR): international collaboration between nuclear reaction data centres (NRDC). Nucl Data Sheets. 2014;120:272–276.
- Chadwick MB, Herman M, Obložinský P, et al. ENDF/B-VII.1 nuclear data for science and technology: cross sections, covariances, fission product yields and decay data. Nucl Data Sheets. 2011;112:2887–2996.
- OECD Nuclear Energy Agency Data Bank. Available from http://www.oecd-nea.org/dbforms/data/eva/ evatapes/jeff_32/
- Nakajima Y. JENDL activation cross section file. In: Igashira M, Nakagawa T, editors. Proceedings of the 1990 Symposium on Nuclear Data; 1990 November 29–30; Tokai; Japan Atomic Energy Research Institute; 1991. p.43–57. ( JAERI-M 91-032).
- Koning A, Forrest R, Kellet M, et al., editors. The JEFF-3.1 nuclear data library. Paris: OECD Nuclear Energy Agency; 2006. ( JEFF Report 21).
- Koehler PE, Guber KH. Improved 192, 194, 195, 196Pt(n, γ) and 192Ir(n, γ) astrophysical reaction rates. Phys Rev C. 2013;88:035802.
- Herman M, Marcinkowski A. Cross sections for fast neutron capture on the Se, Pd, Cd, Os and Pt isotopes. Nucl Phys A. 1981;357:1–8.
- Marganiec J, Dillmann I, Domingo-Pardo C, et al. Stellar (n, γ) cross sections of neutron-rich nuclei: Completing the isotope chains of Yb, Os, Pt, and Hg. Phys Rev C. 2014;90:065801.
- Marganiec J, Dillmann I, Domingo Pardo C, et al. Stellar (n, γ) cross sections of p-process isotopes. II. 168Yb, 180W, 184Os, 190Pt, and 196Hg. Phys Rev C. 2010;82:035806.
- Luo J, He G, Liu Z, Kong X. Cross-section measurements for (n, 2n) and (n, p) reactions on platinum isotopes in the neutron energy range of 13.5 to 14.6 MeV. Radiochim Acta. 2005;93:381–383.
- Paul EB, Clarke RL. Cross-section measurements of reactions induced by neutrons of 14.5 MeV energy. Can J Phys. 1953;31:267–277.
- Bormann M, Bissem HH, Magiera E, et al. Total cross sections and isomeric cross-section ratios for (n, 2n) reactions in the energy region 12–18 MeV. Nucl Phys A. 1970;157:481–496.
- Frehaut J, Bertin A, Bois R, et al. Status of (n, 2n) cross section measurements at Bruyeres-le-Chatel. In: Bhat MR, Pearlstein S, editors. Proceedings of Symposium on Neutron Cross-sections from 10 to 50 MeV; 1980 May 12–14. Upton (NY): Brookhaven National Laboratory; 1980. p. 399–411.
- Vonach H, Pavlik A, Strohmaier B. Accurate determination of (n, 2n) cross sections for heavy nuclei from neutron production spectra. Nucl Sci Eng. 1990;106:409–414.
- Filatenkov AA, Chuvaev SV. Experimental determination of cross sections for several insufficiently known neutron induced reactions on heavy nuclei (Z = 74–79). Moscow: Khlopin Radium Institute; 2003. ( KRI-259).
- Coleman RF, Hawker BE, O'connor LP, et al. Cross sections for (n, p) and (n, α) reactions with 14.5 MeV neutrons. Proc Phys Soc (Lond). 1959;73:215–220.