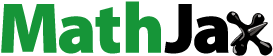
ABSTRACT
The adsorption properties of a porous silica-based adsorbent 2,6-bis(5,6,7,8-tetrahydro-5,8,9,9-tetramethyl-5,8-methano-1,2,4-benzotriazin-3-yl)pyridine (Me2-CA-BTP/SiO2-P) towards Pd(II) were investigated. The adsorption ability of Me2-CA-BTP/SiO2-P towards Pd(II) increased dramatically with the increase of HNO3 concentration and showed a saturated uptake of Pd(II) after the HNO3 concentration reached 3 mol/dm3. It was found that nitrate ion would participate in the adsorption reaction. Palladium adsorption kinetic and isotherm were in accordance with the pseudo-second-order rate law and Langmuir isotherm adsorption model, respectively. Thiourea could effectively elute the adsorbed palladium from Me2-CA-BTP/SiO2-P. Adsorption studies with high level liquid waste elements showed a high selectivity towards Pd(II) over other fission product elements in ≥3 mol/dm3 HNO3 solution. The stability of Me2-CA-BTP/SiO2-P against HNO3 was satisfied for the reason of the adsorption performance keeping for Pd(II) after a long-time contact with HNO3 solution. The adsorbent performed good γ-irradiation stability in dry state and low concentrations of HNO3. The presence of HNO3 enhanced the radiolysis and the adsorbent still reserved a presentable adsorption amount after γ-irradiation in 3 mol/dm3 HNO3.
1. Introduction
The platinum group metals (PGMs) play important roles in chemical, pharmaceutical, electronic and petroleum industries [Citation1,Citation2]. As an important member of PGMs, palladium has been widely used for its unique and useful properties, such as outstanding catalytic characteristics, good malleability, excellent electrical conductivity and high chemical inertness [Citation3]. Therefore, an increasing demand for palladium is followed by the numerous applications and the rapid development of related industries. However, the nature abundance of palladium is only about 10−6% in the earth's crust and would like to be consumed very soon [Citation2,Citation4]. The gap between demand and natural sources could be replenished by seeking alternate resources.
High level liquid waste (HLLW) originating from reprocessing of spent nuclear fuel by plutonium uranium reduction extraction process contains significant amount of fission produced palladium [Citation2,Citation5,Citation6]. According to calculation, spent fuel contains about 1.3 kg Pd per ton from light water reactor (LWR) at the burn-up of 33,000 MWd/t (1 MWd/t = 86.4 GJ/kg), and about 11.1 kg Pd per ton from fast breeder reactor at the burn-up of 150,000 MWd/t [Citation7]. Fission palladium is composed of stable isotopes 104Pd (17%), 105Pd (29%), 106Pd (21%), 108Pd (12%), 110Pd (4%) and a radioactive 107Pd (17%) isotope, which is a soft β-emitter with a half-life of 6.5 × 106 year and a maximum energy of 35 keV [Citation5]. On account of the weak intrinsic radioactivity of 107Pd, it can be tolerated for many industrial applications [Citation8,Citation9]. Furthermore, palladium would form separate phases during the vitrification process of HLLW and cause deteriorations in the stability of the glasses [Citation10,Citation11]. The separation of palladium from HLLW could exclude those problems. Therefore, the recovery of palladium from HLLW is of great significance.
Recovery of palladium from HLLW has been extensively studied. Many methods have been developed, such as ion exchange [Citation12,Citation13], solvent extraction [Citation14,Citation15] and electrodeposition [Citation16,Citation17]. However, the industrialization of recovering palladium from HLLW has not been realized due to the complexity of HLLW. In recent years, there are growing interests in the extraction chromatography for its attractive advantages, such as no or very limited utilization of organic diluents, compact equipment and less waste accumulation [Citation18–20]. Extraction chromatography achieves a solid-adsorbent capable of selectively removing certain metal ions from liquid solution by using an inert support impregnated with an extractant. Especially in the field of dealing with HLLW, extraction chromatography has attracted much attention. Wei et al. [Citation21,Citation22] have proposed and designed some extraction chromatography processes for key elements separation from HLLW. In those processes, palladium could achieve separation by using a series of silica-based adsorbents such as CMPO/SiO2-P and R-BTP/SiO2 -P (BTP: 2,6-bis-(5,6-dialkyl-1,2,4-triazin-3-yl)-pyridines, R: alkyl group). According to the recent studies, Liu et al. [Citation23] achieved a good recovery of palladium by using isohexyl-BTP/SiO2-P; Ito et al. [Citation24] investigated the adsorption behaviors of PGMs in simulated HLLW by using MOTDGA-TOA/SiO2-P; Xu et al. [Citation20,Citation25] studied chromatographic separation of PGMs from simulated HLLW using (Crea + Dodec)/SiO2-P, (Crea + TOA)/SiO2-P and (MOTDGA + TOA)/SiO2-P, respectively; Bai et al. [Citation26] studied the recovery of palladium from simulated HLLW by a new silica-based adsorbent functionalized with macrocyclic ligand. Among them, R-BTP/SiO2-P could achieve the separation of Pd(II) from HLLW along with MA(III), which would greatly reduce the wastes produced in the partitioning process [Citation27]. Therefore, several process such as modified MAREC (minor actinides recovery from acidic high active liquid waste(HLW) by extraction chromatography) process [Citation23], MPS (the long-lived MA(III) and Pd(II) separation) process [Citation27] and SPMP (simultaneous partitioning of MA(III)/Pd(II) from HLW by extraction chromatography) process [Citation28] were proposed to separate MA(III) and Pd(II) simultaneously in a single extraction chromatography system. However, the traditional BTPs showed the weakness towards acidic hydrolysis and radiolysis [Citation29]. Chemical attack upon the benzylic hydrogens has been shown to be responsible for much of the degradation [Citation30]. To overcome such drawbacks, many attempts have been made to modify the BTPs in order to enhance its stability. In our previous works [Citation18,Citation31], a novel adsorbent, Me2-CA-BTP/SiO2-P was synthesized. In the structure of Me2-CA-BTP, all the benzylic hydrogens have been replaced with new-type cycle structures. Me2-CA-BTP/SiO2-P has exhibited a good performance and could be a potential candidate used in an advanced partitioning process for key elements separation from HLLW, but its adsorption behavior towards Pd(II) and its stability against nitric acid and γ-irradiation have not yet been studied in detail.
This work studied the adsorption behavior of Me2-CA-BTP/SiO2-P towards Pd(II). The adsorbent was synthesized by impregnating Me2-CA-BTP into a stable macroporous silica/polymer composite support (SiO2-P particles). The adsorption kinetics, isotherm and elution performance were examined. The adsorption behaviors of the adsorbent towards the typical fission products (FPs) contained in HLLW were also studied. In addition, the stability of Me2-CA-BTP/SiO2-P against nitric acid and γ-irradiation was investigated.
2. Experimental
2.1. Materials and characterization
All the reagents used in this work such as nitric acid, Pd(NO3)2•2H2O, thiourea (TU), etc. were of analytical grade. The solutions of Pd(II) were prepared by dissolving Pd(NO3)2•2H2O (ACROS, Belgium) into the appropriate concentration of nitric acid. The purity of extracting agent, Me2-CA-BTP, was 97%. The Me2-CA-BTP/SiO2-P adsorbent was prepared as described in the previous studies with Me2-CA-BTP/SiO2-P replacing nBu-BTP [Citation19]. The silica/polymer composite support (SiO2-P) with pore size of 0.6 m, pore fraction of 0.69 and mean diameter of 50 m was developed in the previous work [Citation32]. P refers to macroreticular styrene–divinylbenzene copolymer (SDB) and is immobilized in porous silica (SiO2) particles with the content of 17%–18% of the total mass of SiO2-P. Finally, 0.5g of Me2-CA-BTP was impregnated into 1.0 g silica/polymer composite support (SiO2-P). In other words, the content of extracting agent was 33.3% of the total mass of the adsorbent. (a,b) shows the chemical structure of Me2-CA-BTP and scanning electron microscope (SEM) image of Me2-CA-BTP/SiO2-P, respectively.
2.2. Batch adsorption and desorption experiments
The adsorption properties of Me2-CA-BTP/SiO2-P adsorbent towards Pd(II) were investigated by batch experiment. For each batch experiment, 0.1 g adsorbent was combined with 5 cm3 aqueous solution containing required concentration of metal ions in a 10 cm3 glass vial with screw cap. The mixture in the vial was shaken mechanically at 120 rpm at a constant temperature for determined time in a water bath. The aqueous phase was filtrated through a membrane filter with 0.45 m pore. The concentrations of metal ions in solutions before and after adsorption were measured by inductively coupled plasma atomic emission spectrometry instrument (ICP-7510, Shimadzu, Japan). The radioactivity of 241Am was measured by a NaI (Tl) γ-ray spectrometry (Seiko EG&G).
The distribution coefficient Kd (cm3/g) and adsorption amount per unit adsorbent Q (mmol/g) are calculated by following equations, respectively:
(1)
(1)
(2)
(2)
where C0, Ce (mmol/dm3) are the concentration of metal ions in the aqueous phase before and after adsorption, respectively. V (cm3) refers to the volume of aqueous phase and W (g) is the mass of dry Me2-CA-BTP/SiO2-P adsorbent.
In batch desorption experiments, Me2-CA-BTP/SiO2-P loaded by contacting with a solution containing Pd(II) in 3 mol/dm3 HNO3 solution was used to examine the desorption behavior. TU solution was used as eluting reagent, which was obtained by dissolving TU into H2O. The loaded adsorbent was contacted with TU solution in a 10 cm3 glass vial and separated from the aqueous solution after shaking for 24 h. Then, the metal ion concentrations in desorption solutions were measured as described earlier.
2.3. Hydrolytic and γ-irradiation stability experiment
To investigate the stability of Me2-CA-BTP/SiO2-P adsorbent against nitric acid, 0.1 g adsorbent and 5 cm3 nitric acid solutions were mixed into a 10 cm3 glass vial, which was shaken mechanically at 120 rpm at a designed contact time. Then the adsorbent was used for the adsorption of Pd(II) in batch experiments to exam the property changes after filtrated and dried.
In order to simulate the actual situation of HLLW, this work also investigated the adsorption behavior of Me2-CA-BTP/SiO2-P towards Pd(II) after γ-irradiation. 0.1 g adsorbent was kept into a 10 cm3 glass vial at a dry state or dipped into a 5 cm3 nitric acid solution. Then the adsorbent was irradiated under an absorbed dose rate of 1.0 kGy/h for a determined time at room temperature with Co-60 as the radioactive source (Shanghai Institute of Applied Physics, China). The vials were placed around the Co-60 source at an appropriate distance to receive the radiation dose. The dose rate was precisely calibrated for each vial's position in advance. After irradiation, the adsorbent was dried and then evaluated by batch adsorption experiment.
3. Results and discussion
3.1. Effect of HNO3 concentration
The effect of nitric acid on the adsorption ability of Me2-CA-BTP/SiO2-P towards Pd(II) was investigated. The results are plotted in . Pd(II) is a well-known soft metal ion containing an unsaturated d-orbital which has strong ability of electron acceptance, and thus forms stable complexes with the soft ligand, Me2-CA-BTP, which contains nitrogen atoms bearing lone-pair electrons [Citation21]. As seen in , the adsorption ability of Me2-CA-BTP/SiO2-P towards Pd(II) increased dramatically with the increase of HNO3 concentration. The results may be explained by the participation of nitrate ion in the adsorption reaction, which was similar to the adsorption behavior of BTPs towards An(III) [Citation33,Citation34]. The adsorbent showed a saturated uptake of Pd(II) after the HNO3 concentration reached to 3 mol/dm3. As the HNO3 concentration of HLLW is normally as high as 3--5 mol/dm3, the results indicate that the adsorbent would be suitable for potential application.
3.2. Adsorption kinetics
The influence of contact time on Pd(II) adsorption was studied in 3 mol/dm3 HNO3 at 298 K. The adsorption kinetic results are shown in . It is obvious that Me2-CA-BTP/SiO2-P adsorbed Pd(II) quickly in the first few hours. After 12 h, the adsorption slowed down and reached an equilibrium state gradually. Pseudo-first-order model and pseudo-second-order model [Citation35] were applied to analyze the experimental data in order to figure out the kinetic mechanism of the adsorption process. Pseudo-first-order model is represented by
(3)
(3)
where k1 (h−1) is the adsorption rate constant of pseudo-first-order model, Qt (mmol/g) denotes the adsorption capacity at time t (h) and Qe (mmol/g) is the equilibrium adsorption amount. Pseudo-second-order model can be written as
(4)
(4)
where k2 (g/(mmol h)) is the adsorption rate constant of pseudo-second-order model.
Figure 3. Adsorption kinetics and pseudo-first-order and pseudo-second-order models of Pd(II) on Me2-CA-BTP/SiO2-P in 3.0 mol/dm3 HNO3 solution (adsorbent: 0.1 g, solution: 5 cm3, [Pd]: 20 mmol/dm3, temperature: 298 K, shaking speed: 120 rpm).
![Figure 3. Adsorption kinetics and pseudo-first-order and pseudo-second-order models of Pd(II) on Me2-CA-BTP/SiO2-P in 3.0 mol/dm3 HNO3 solution (adsorbent: 0.1 g, solution: 5 cm3, [Pd]: 20 mmol/dm3, temperature: 298 K, shaking speed: 120 rpm).](/cms/asset/c535ccfe-90bb-41a3-b704-a7aaca03cbb5/tnst_a_1298481_f0003_oc.jpg)
The fitted curves are plotted in and the fitted parameters were calculated and are listed in . The correlation coefficient R2 shows that the fitting results agree very well with pseudo-second-order model. The calculated equilibrium adsorption amount (Qe) is 0.78 mmol/g, which is well consistent with the experiment results (0.76 mmol/g). It can be suggested that the adsorption is controlled by a chemical adsorption process. In addition, as illustrated in , the adsorption spent a long time to reach an equilibrium state. It is known that the adsorption kinetics is controlled by the diffusion step inside the resin in many cases and a reduction particle size of resin would benefit a rapid kinetics. In consideration of the small size of SiO2-P, the slow adsorption kinetics is considered to result mainly from the complexation reaction between Pd(II) and Me2-CA-BTP/SiO2-P [Citation27].
Table 1. Fitted parameters of pseudo-first-order model and pseudo-second-order model for Pd(II) adsorption.
3.3. Adsorption isotherm
To obtain a further understanding of the adsorption behavior, the relationship between the concentration of Pd(II) in solution and the amount of Pd(II) adsorbed onto Me2-CA-BTP/SiO2-P adsorbent was examined. As shown in , the adsorption amount increased as the equilibrium concentration increased. The adsorption data were fitted with two basically theoretical isotherm models, i.e. the Langmuir equation and the Freundlich equation.
Figure 4. Adsorption isotherm of Pd(II) on Me2-CA-BTP/SiO2-P in 3.0 mol/dm3 HNO3 solution (adsorbent: 0.1 g, solution: 5 cm3, contact time: 24 h, temperature: 298 K, shaking speed: 120 rpm).
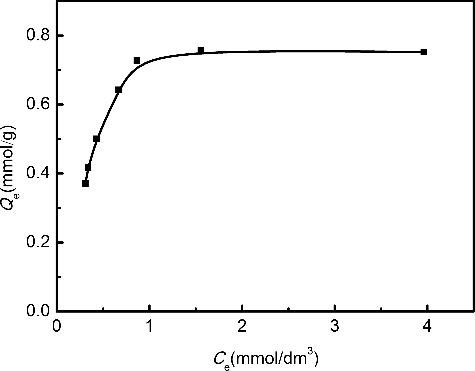
The Langmuir model assumes that the adsorption reaction of adsorbate is on a homogeneous, flat surface of an adsorbent with constant adsorption energy, and each adsorptive site can be occupied only once in a one-on-one monolayer adsorption manner [Citation36]. The linearized model can be written as
(5)
(5)
where Ce (mmol/dm3) is the equilibrium concentration, Qe (mmol/g) is the equilibrium absorption amount, Qmax (mmol/g) is the saturated adsorption amount and KL (dm3/mmol) is the Langmuir constant.
On the other hand, Freundlich model describes adsorption on a heterogeneous surface with uniform energy, which is represented by the following equation [Citation37]:
(6)
(6)
where KF (mmol/g) and n are the Freundlich constants.
The fitted parameters were calculated and are listed in . In comparison, the adsorption behavior of Pd(II) is better fitted by the Langmuir model with the coefficient (R2) of 0.99. The fitted curve of Langmuir model is plotted in . The apparent adsorption capacity Qmax was calculated to be 0.80 mmol/g from the slope of linear approximation, which is very close to the experimental data. The results suggest that the Pd(II) uptake on Me2-CA-BTP/SiO2-P could be more reasonably regarded as monolayer adsorption.
Table 2. Fitted parameters of Langmuir and Freundlich models for Pd (II) adsorption.
3.4. Desorption behavior
Me2-CA-BTP/SiO2-P has showed good adsorption behavior towards Pd(II), while its desorption performance is also an important property when evaluating the adsorbent for practical application. The desorption performance is evaluated by using desorption efficiency, which is calculated by EquationEquations (7)(7)
(7) and (Equation8
(8)
(8) ).
(7)
(7)
(8)
(8)
where Qd and Q are the desorption amount and adsorption amount per unit adsorbent in mmol/g, respectively. Cd denotes the desorbed metal ion concentration in eluent.
The Pd(II) strongly adsorbed onto the resin could not be desorbed with H2O and any dilute HNO3 solution. Therefore, TU (CH4N2S) was attempted for the desorption, which was often used as a palladium eluent [Citation23]. The desorption behavior of Pd(II) from Me2-CA-BTP/SiO2-P by TU (CH4N2S) is presented in . TU shows an excellent desorption behavior. Pd(II) is a soft acid while TU contains ‘N’ and ‘S’ atoms, which are always likely to react with Pd(II) and form stable complexes [Citation38]. The desorption efficiency increases along with the increase of TU concentration and could reach 90% when the TU concentration was only 0.05 mol/dm3. After the TU concentration reached 0.3 mol/dm3, a very high desorption efficiency of 95% was obtained, revealing that TU is a promising eluent.
Figure 6. Effect of TU concentration on desorption of Pd(II) from Me2-CA-BTP/SiO2-P adsorbent (adsorption conditions: adsorbent: 0.1 g, solution: 5 cm3, [Pd]: 20 mmol/dm3, nitric acid: 3.0 mol/dm3 HNO3, temperature: 298 K, contact time: 24 h, shaking speed: 120 rpm; desorption conditions: adsorbent: 0.1 g, solution: 5 cm3, temperature: 298 K, contact time: 24 h, shaking speed: 120 rpm).
![Figure 6. Effect of TU concentration on desorption of Pd(II) from Me2-CA-BTP/SiO2-P adsorbent (adsorption conditions: adsorbent: 0.1 g, solution: 5 cm3, [Pd]: 20 mmol/dm3, nitric acid: 3.0 mol/dm3 HNO3, temperature: 298 K, contact time: 24 h, shaking speed: 120 rpm; desorption conditions: adsorbent: 0.1 g, solution: 5 cm3, temperature: 298 K, contact time: 24 h, shaking speed: 120 rpm).](/cms/asset/4c5ed2db-062b-4c5b-bda6-a1cd07d4e224/tnst_a_1298481_f0006_b.gif)
3.5. Adsorption behavior towards typical fission product elements
To evaluate the feasibility of Me2-CA-BTP/SiO2-P for palladium separation from HLLW, the adsorption behaviors of Me2-CA-BTP/SiO2-P towards the typical FP elements contained in HLLW were studied. shows the distribution ratio of these elements at different concentrations of HNO3. As can be seen, the adsorbent showed no or weak adsorption towards Ce(III), Zr(IV), Sr(II), Cs(I), Nd(III), Y(III) Sm(III) and La(III) at all the tested HNO3 concentrations. Eu(III), Gd(III) and Ru(III) exhibited a strong adsorption onto the adsorbent at about 1 mol/dm3 HNO3 and then decreased with increasing nitric acid concentration. The high selectivity of Me2-CA-BTP/SiO2-P towards Pd(II) was rather obvious in ≥3 mol/dm3 HNO3 solution. In 3 mol/dm3 HNO3, the Kd of Pd(II) could reach 65 cm3/g. shows the separation factor (SF) between Pd(II) and some typical FP ions, which was calculated as the ratio of respective Kd values. Notably, Me2-CA-BTP/SiO2-P still keeps adsorption abilities towards 241Am in 3 mol/dm3 HNO3. However, the adsorbed 241Am could be eluted from the adsorbent with water or diluted HNO3 according to our previous works [Citation18]. In consideration of the desorption behavior of Me2-CA-BTP/SiO2-P towards Pd(II) discussed earlier, Pd(II) and MA(III) could achieve separation from HLLW, respectively, by using TU and water as eluents.
Figure 7. Distribution ratios of typical HLLW elements at different concentrations of HNO3 (adsorption conditions: adsorbent: 0.1 g, solution: 5 cm3, 241Am(III): 800 Bq/L, metal: 1 mmol/dm3, temperature: 298 K, contact time: 24 h, shaking speed: 120 rpm).
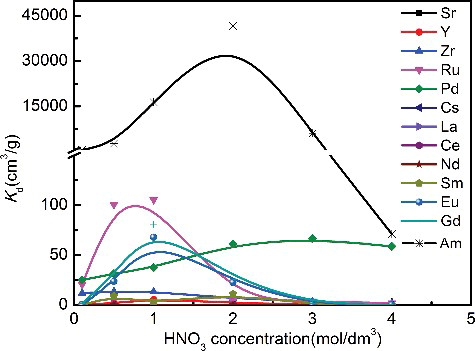
Table 3. Separation factor (SF) between Pd(II) and some typical FP ions.
3.6. Hydrolytic stability studies
The chemical stability of the adsorbent was investigated by a set of experiments performed in 0.5, 1 and 3 mol/dm3 HNO3. shows the effect of contact time with nitric acid solution on the adsorption of Pd(II) onto Me2-CA-BTP/SiO2-P. As can be seen, the adsorption amount of Pd(II) remained virtually unchanged during the experimental contact time in 0.5 and 1 mol/dm3 HNO3, while a decrease occurred in 3 mol/dm3 HNO3. The results indicate that the adsorbent was affected by high concentrations of nitric acid and a limited quantity of extractant would be leaked into aqueous solution from the adsorbent. However, the adsorbent still reserved an adsorption amount of about 0.63 mmol/g and it showed almost no change as the contact time increased. In consideration of the adsorption performance in a long-time contact with 3 mol/dm3 HNO3 solution, Me2-CA-BTP/SiO2-P could be used a few more times in column chromatographic process.
3.7. Irradiation stability studies
Due to the strong irradiation condition of HLLW, the irradiation stability is a key property when evaluating the adsorbent for Pd recovery. At first, Me2-CA-BTP/SiO2-P was irradiated in dry state for 23, 46, 69 and 92 h with the absorbed radiation dose rate of 1 kGy/h. After irradiation, the adsorbent was used to study the adsorption behavior towards Pd(II) and the results are illustrated in . As shown in this figure, it is obvious that Me2-CA-BTP/SiO2-P has presented a good stability under γ-irradiation in dry state. This behavior suggests that there is no direct and exclusive degradation of the ligand by direct gamma irradiation.
Figure 9. Effect of absorbed dose on Me2-CA-BTP/SiO2-P(dry state) adsorption towards Pd(II) (adsorption conditions: adsorbent: 0.1 g, solution: 5 cm3, [Pd]: 20 mmol/dm3, nitric acid: 3.0 mol/dm3 HNO3, temperature: 298 K, contact time: 24 h, shaking speed: 120 rpm).
![Figure 9. Effect of absorbed dose on Me2-CA-BTP/SiO2-P(dry state) adsorption towards Pd(II) (adsorption conditions: adsorbent: 0.1 g, solution: 5 cm3, [Pd]: 20 mmol/dm3, nitric acid: 3.0 mol/dm3 HNO3, temperature: 298 K, contact time: 24 h, shaking speed: 120 rpm).](/cms/asset/5ea2a04f-7d37-49fa-9a46-64d145edfeb4/tnst_a_1298481_f0009_b.gif)
Then the adsorbent was irradiated in nitric acid solution and accomplished adsorption at the same nitric acid concentration accorded with irradiation condition. shows the results. It is clear that Me2-CA-BTP/SiO2-P still keeps good adsorption ability in nitric acid under irradiation. Compared with the results in , γ-irradiation has certainly had an adverse effect on the adsorption, which may owe to the radiolysis and the change of the structure of Me2-CA-BTP/SiO2-P brought by γ-irradiation. It is well known that during irradiation, reactive radicals are formed mainly by radiolysis, which can react with the extractant molecules and lead to the degradation of the ligand [Citation39]. However, Me2-CA-BTP/SiO2-P still reserved an adsorption amount of about 0.5 mmol/g after a long-time irradiation in 3 mol/dm3 HNO3. Compared with some other R-BTP extractants such as isoHex-BTP/SiO2-P, which completely lost its efficacy at an absorbed dose of 46 kGy with the absorbed dose 1 kGy/h, the stability of Me2-CA-BTP/SiO2-P has enhanced obviously [Citation40]. Moreover, the dose rates to the solvent in the reprocessing of standard spent LWR fuels are burn-up dependent and range from 0.03 to 0.2 kGy/h for UO2 fuels and from 0.4 to 0.8 kGy/h for mixed oxide (MOX) fuels [Citation39], which is smaller than the experimental dose in this study. Therefore, Me2-CA-BTP/SiO2-P is a potential candidate for separating Pd(II) from HLLW.
4. Conclusions
In this study, the adsorption properties of a novel silica-based adsorbent, Me2-CA-BTP/SiO2-P towards Pd(II) were investigated. Me2-CA-BTP/SiO2-P showed strong adsorption towards Pd(II). The adsorption ability increased dramatically with the increase of HNO3 concentration. The adsorption kinetics matched well with pseudo-second-order rate law while the adsorption isotherm was in accordance with Langmuir isotherm adsorption model with the maximum adsorption capacity of about 0.80 mmol/g. The adsorbed palladium could be effectively eluted by TU from Me2-CA-BTP/SiO2-P with a high desorption rate of 95%. Me2-CA-BTP/SiO2-P showed a high selectivity towards Pd(II) in ≥3 mol/dm3 HNO3 solution over the typical FP elements. The adsorbent performed a good behavior against nitric acid and γ-irradiation. The presence of HNO3 enhanced the radiolysis, but it still reserved an adsorption amount of about 0.5 mmol/g when the radiation dose was up to 92 kGy in 3 mol/dm3 HNO3. The results demonstrated that Me2-CA-BTP/SiO2-P is a promising adsorbents for the recovery of Pd(II) along with the separation of MA(III) from HLLW.
Acknowledgments
This work was supported by the National Natural Science Foundation [grant number 11305102], [grant number 91126006], [grant number 21261140335].
Disclosure statement
No potential conflict of interest was reported by the authors.
Additional information
Funding
References
- Acres GJK. Platinum group metal catalysis at the end of this century. Platinum Met Rev. 1984;28:150–157.
- Ruhela R, Sharma JN, Tomar BS , et al. N, N, N′, N′-tetra(2-ethylhexyl) thiodiglycolamide T(2EH)TDGA: a novel ligand for the extraction of palladium from high level liquid waste (HLLW). Radiochim Acta. 2010;98:209–214.
- Mudd GM. Sustainability reporting and the platinum group metals: a global mining industry leader? Platinum Met Rev. 2012;56:2–19.
- Ruhela R, Singh AK, Tomar BS , et al. Separation of palladium from high level liquid waste – a review. RSC Adv. 2014;4:24344–24350.
- Kolarik Z, Renard EV. Recovery of value fission platinoids from spent nuclear fuel part I: general considerations and basic chemistry. Platinum Met Rev. 2003;47:74–87.
- Kolarik Z, Renard EV. Recovery of value fission platinoids from spent nuclear fuel part II: separation processes. Platinum Met Rev. 2003;47:123–131.
- Venkatesan KA, Selvan BR, Antony MP , et al. Extraction of palladium from nitric acid medium by commercial resins with phosphinic acid, methylene thiol and isothiouronium moieties attached to polystyrene-divinylbenzene. J Radioanal Nucl Chem. 2005;266:431–440.
- Jayakumar M, Venkatesan KA, Srinivasan TG , et al. Extraction–electrodeposition (EX–EL) process for the recovery of palladium from high-level liquid waste. J Appl Electrochem. 2009;39:1955–1962.
- Ruhela R, Sharma JN, Tomar BS , et al. N,N,N′,N′-Tetra(2-Ethylhexyl) Thiodiglycolamide T(2EH)TDGA: a promising ligand for separation and recovery of palladium from high level liquid waste (HLLW) solutions. Sep Sci Technol. 2011;46:965–971.
- Grünewald W, Roth G, Tobie W , et al. The role of the platinum group elements ruthenium, rhodium and palladium in the vitrification of radioactive high level liquid waste using joule heated ceramic lined waste glass melters. Glass Technol-Part A. 2008;49:266–278.
- Sundaram SK, Perez JM Jr. Noble metals and spinel settlings in high level waste melters. PNNL-13347. Richland (WA): Pacific Northwest National Laboratory; 2000.
- Lee SH, Chung H. Ion exchange characteristics of palladium and ruthenium from a simulated radioactive liquid waste. Sep Sci Technol. 2003;38:3459–3472.
- Fujiwara K, Ramesh A, Maki T , et al. Adsorption of platinum (IV), palladium (II) and gold (III) from aqueous solutions onto l-lysine modified crosslinked chitosan resin. J Hazard Mater. 2007;146:39–50.
- Giridhar P, Venkatesan KA, Srinivasan TG , et al. Extraction of fission palladium by Aliquat 336 and electrochemical studies on direct recovery from ionic liquid phase. Hydrometallurgy. 2006;81:30–39.
- Parajuli D, Hirota K. Recovery of palladium using chemically modified cedar wood powder. J Colloid Interface Sci. 2009;338:371–375.
- Liu SC, Wei YZ, Liu RQ , et al. Electrochemical behavior and electrowinning of palladium in nitric acid media. Sci China Chem. 2013;56:1743–1748.
- Koizumi K, Ozawa M, Kawata T. Electrolytic extraction of platinum group metals from dissolver solution of purex process. J Nucl Sci Technol. 1993;30:1195–1197.
- Ning SY, Wang XP, Liu RQ , et al. Evaluation of Me2-CA-BTP/SiO2-P adsorbent for the separation of minor actinides from simulated HLLW. J Radioanal Nucl Chem. 2015;303:2011–2017.
- Wei YZ, Yamaguchi M, Kumagai M , et al. Separation of actinides from simulated spent fuel solutions by an advanced ion-exchange process. J Alloy Compd. 1998;271:693–696.
- Xu YL, Kim SY, Ito T , et al. Chromatographic separation of platinum group metals from simulated high level liquid waste using macroporous silica-based adsorbents. J Chromatogr A. 2013;1312:37–41.
- Wei YZ, Wang XP, Liu RQ , et al. An advanced partitioning process for key elements separation from high level liquid waste. Sci China Chem. 2012;55:1726–1731.
- Wei YZ, Zhang AY, Kumagai M , et al. Development of the MAREC process for HLLW partitioning using a novel silica-based CMPO extraction resin. J Nucl Sci Technol. 2004;41:315–322.
- Liu RQ, Wei YZ, Xu YL , et al. Evaluation study on properties of isohexyl-BTP/SiO2-P resin for direct separation of trivalent minor actinides from HLLW. J Radioanal Nucl Chem. 2012;292:537–544.
- Ito T, Kim SY, Xu YL , et al. Adsorption behaviors of platinum group metals in simulated high level liquid waste using macroporous (MOTDGA-TOA)/SiO2-P silica-based absorbent. Sep Sci Technol. 2013;48:2616–2625.
- Xu YL, Kim SY, Ito T , et al. Adsorption properties and behavior of the platinum group metals onto a silica-based (Crea + TOA)/SiO2–P adsorbent from simulated high level liquid waste of PUREX reprocessing. J Radioanal Nucl Chem. 2012;297:41–48.
- Bai FF, Ye G, Chen GJ , et al. Highly selective recovery of palladium by a new silica-based adsorbent functionalized with macrocyclic ligand. Sep Purif Technol. 2013;106:38–46.
- Zhang AY, Jiang JL, Chai ZF. Preparation of a macroporous silica-based multidentate soft-ligand material and its application in the adsorption of palladium and the others. Sep Sci Technol. 2013;48:1500–1509.
- Zhang AY, Xue WJ, Dong XC. Minor actinides partitioning: uptake of palladium with triazine-containing adsorbent under the framework of SPMP process. J Chem Eng Data. 2016;61:3303–3310.
- Mincher BJ, Modolo G, Mezyk SP. Review: the effects of radiation chemistry on solvent extraction 4: separation of the trivalent actinides and considerations for radiation-resistant solvent systems. Solvent Extr Ion Exch. 2010;28:415–436.
- Hudson MJ, Boucher CE, Braekers D , et al. New bis(triazinyl) pyridines for selective extraction of americium(III). New J Chem. 2006;30:1171–1183.
- Ning SY, Zou Q, Wang XP , et al. Adsorption behavior of Me2-CA-BTP/SiO2-P adsorbent toward MA(III) and Ln(III) in nitrate solution. Sci China Chem. 2016;59:862–868.
- Wei YZ, Kumagai M, Takashima Y , et al. Studies on the separation of minor actinides from high-level wastes by extraction chromatography using novel silica-based extraction resins. Nucl Technol. 2000;132:413–423.
- Ning SY, Zou Q, Wang XP , et al. Adsorption mechanism of silica/polymer-based 2,6-bis(5,6-diisohexyl-1,2,4-triazin-3-yl)pyridine adsorbent towards Ln(III) from nitric acid solution. J Nucl Sci Technol. 2016;53:1417–1425.
- Madic C, Lecomte M, Baron P , et al. Separation of long-lived radionuclides from high active nuclear waste. CR Phys. 2002;3:797–811.
- Ho YS, McKay G. A comparison of chemisorption kinetic models applied to pollutant removal on various sorbents. Process Saf Environ. 1998;76:332–340.
- Langmuir I. The constitution and fundamental properties of solids and liquids. II. Liquids. J Am Chem Soc. 1917;39:1848–1906.
- Freundlich H. Kapillarchemie. Leipzig: Akademische Verlagsgesellschaft; 1930. p. 72–88.
- Wei YZ, Liu RQ, Wu Y , et al. Chromatographic separation of actinides and fission products. Energy Procedia. 2013;39:110–119.
- Magnusson D, Christiansen B, Malmbeck R , et al. Investigation of the radiolytic stability of a CyMe4-BTBP based SANEX solvent. Radiochim Acta. 2009;97:497–502.
- Wang XP, Ning SY, Liu RQ , et al. Stability of isoHex-BTP/SiO2-P adsorbent against acidic hydrolysis and γ-irradiation. Sci China Chem. 2014;57:1464–1469.