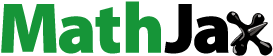
ABSTRACT
Aiming at the selective recovery of fission palladium(II) from high-level liquid waste (HLLW), the silica/polymer (SiO2-P)-based isoHex-BTP adsorbent (isoHex-BTP/SiO2-P) was synthesized by impregnating complexing agent isoHex-BTP into the multiporous SiO2-P inert support. The feasibility of separation of Pd(II) from HLLW by isoHex-BTP/SiO2-P was evaluated by batch experiment method. The results showed that isoHex-BTP/SiO2-P exhibited much higher adsorption selectivity for Pd(II) than the other fission products, even Am(III) and Pu(IV) presented in HLLW. The ideal nitric acid concentration for the adsorption of Pd(II) by the adsorbent was shown to be ≥2 mol dm–3. The adsorption of Pd(II) fits well to the pseudo-second-order kinetic model and Langmuir isotherm model. Quantitative Pd(II) desorption was achieved by using 0.5 mol dm−3 SC(NH2)2 - 0.1 mol dm−3 HNO3 solution.
Introduction
High-level liquid waste (HLLW) arising from PUREX process for the reprocessing of spent nuclear fuel contains significant quantities of valuable fission palladium, namely, a few kilograms per ton of spent fuel [Citation1]. Palladium usually interferes with vitrification of HLLW by formation of distinct phases during vitrificaiton process leading to a non-homogeneous glass matrix, and thereby destabilizing the vitrified product [Citation2,Citation3]. This problem is more serious and undesirable if HLLW is from fast breeder reactors (FBRs) [Citation4]. Therefore, there is a growing interest in the recovery of valuable palladium from HLLW prior to vitrification to eliminate complex problems and to improve the quality of final waste form of vitrified HLLW. In addition, the fission palladium comprises majority of stable isotopes 104Pd (17 wt. %), 105Pd (29 wt. %), 106Pd (21 wt. %), 108Pd (12 wt. %), 110Pd (4 wt. %) and small amount of a very weak radioactive isotope (107Pd, 17 wt. %, soft β-emitter with Emax of 35 keV) which has a half-life of 6.5 × 106 years [Citation4]. The recovered palladium from HLLW could find applications in various fields where the activity of 107Pd can be tolerated [Citation5], which can relieve the increasing pressure about the imbalance between supply and demand of this precious metal [Citation6].
Several separation methods such as solvent extraction [Citation3,Citation7,Citation8], ion exchange [Citation9,Citation10], electrochemical recovery [Citation11,Citation12], etc. have been reported for the recovery of palladium from HLLW in the last two decades. Compared to these methods, extraction chromatography method is particularly attractive as it involves the high selectivity, the simultaneous adsorption and different elution of several elements, low inventory of organic extractants and solvents leading to less waste accumulation and compact equipment [Citation6,Citation13,Citation14].
On the other hand, in P & T (partitioning and transmutation) strategy, the selective separation of trivalent minor actinides (MA(III): Am(III) and Cm(III)) from HLLW is one of the most important and challenging tasks that remains to be solved [Citation15,Citation16]. To solve this problem, we have reported a direct separation process of MA(III) from HLLW based on extraction chromatography () [Citation17]. The process uses a single-column packed with silica/polymer-based BTP (2,6-bis(5,6-dialkyl-1,2,4-triazin-3-yl)pyridine) adsorbent named as BTP/SiO2-P, e.g. isoHex-BTP/SiO2-P (isoHex-BTP: 2,6-bis(5,6-diisoHexyl)-1,2,4-triazin-3-yl)pyridine). The extraction chromatographic material, isoHex-BTP/SiO2-P adsorbent, was prepared using silica/polymer (SiO2-P) as the inert composite support and isoHex-BTP as the extracting agent. SiO2-P support contains a macroreticular styrene-divinylbenzene copolymer which is immobilized in porous silica particles with pore size of 0.6 μm and mean diameter of 60 μm. The content of extracting agent isoHex-BTP in isoHex-BTP/SiO2-P was relatively high (up to 33.3% of the total mass of the adsorbent) [Citation18]. Our research indicates that the method is very promising to perform the separation of MA(III) from HLLW due to the major merits of this process including BTPs exhibiting extraordinary selectivity for MA(III) in HLLW, BTP fulfilling the CHON principle (i.e. the species consist entirely of carbon, hydrogen, oxygen, and nitrogen atoms to make them combustible to safe gaseous products), the high content of extracting agent BTP in BTP/SiO2-P adsorbent leading to high loading capacity and small scale equipment [Citation17,Citation18].
Figure 1. Conceptual flowchart of the direct separation process of MA (III) from fission products present in HLLW by extraction chromatography.
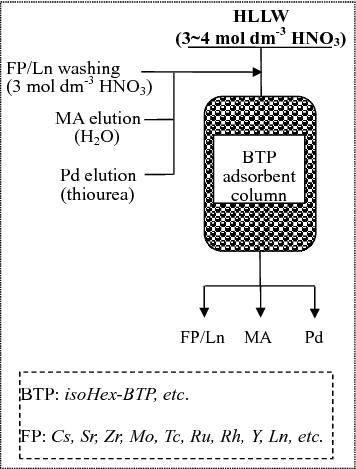
In the course of our research on the process, it was found that isoHex-BTP/SiO2-P exhibited extraordinary selectivity for Pd(II) over other fission products (FP) and even over MA(III). The adsorbed Pd(II) can be eluted by thiourea (SC(NH2)2), while MA(III) can be eluted by distilled water or diluent nitric acid solution [Citation18]. So the present work is aimed to study the feasibility of simultaneous separation of Pd(II) while MA(III) are separated from HLLW using isoHex-BTP/SiO2-P by extraction chromatography process. The study involves the adsorption and desorption behavior of isoHex-BTP adsorbent. The selective adsorption of Pd(II), effects of nitric acid concentration, contact time and concentration of Pd(II) on the adsorption of Pd(II) from nitric acid solution by isoHex-BTP/SiO2-P were studied. The experimental adsorption data depending on contact time were analyzed using the pseudo-first-order, pseudo-second-order, intra-particle diffusion, and Elovich kinetic models to interpret the adsorption kinetics. The equilibrium adsorption data were analyzed using the Freundlich and Langmuir isotherm models. Furthermore, the desorption conditions for Pd(II) recovery were also sought for.
Experimental
Reagent preparation
Nitrates of rare earth (RE) such as Y(III), La(III), Ce(III), Nd(III), Sm(III), Eu(III), Gd(III) (RE(NO3)3·xH2O, x = 5 or 6) and other FP elements (Sr(II), Zr(IV), Mo(VI) and Pd(II)) reagents were of commercial reagents of analytical grade. Ru(III) solution was obtained by diluting a commercially available nitrosyl nitrate solution containing 1.5% of Ru(III). 1 mmol dm–3 of depleted U(VI) and radioactive tracers of 241Am(III), 99Tc(VII) and 239Pu(IV) were prepared from their stocked solutions, respectively.
All the other reagents used were analytical grade chemicals.
Adsorbent
The purity of synthesized extracting agent, isoHex-BTP, was 99%. isoHex-BTP/SiO2-P adsorbent was prepared as described in the previous studies of Wei, et al. [Citation19–21] by impregnating isoHex-BTP molecules into the pores of the silica/polymer composite support (SiO2-P). isoHex-BTP/SiO2-P contained 0.5 g of isoHex-BTP in 1.0 g of SiO2-P, i.e. the content of extracting agent isoHex-BTP was up to 33.3% of the total mass of the adsorbent. The chemical structure of isoHex-BTP and scanning electron microscope (SEM) image of isoHex-BTP/SiO2-P are shown in [Citation18].
Batch adsorption experiment
Distribution coefficient (Kd) and adsorption capacity (q) of metal ions were measured by batch adsorption method. In a batch adsorption experiment, 0.1 g of isoHex-BTP/SiO2-P and 5 cm3 of aqueous solution containing metal ions and nitric acid were put into a 10 cm3 glass vial sealed with Teflon stopper and the vial was maintained at 298 K in a thermostatic water bath and shaken mechanically at 120 rpm for a certain contact time. Then isoHex-BTP/SiO2-P was separated from the aqueous phase through a membrane filter with 0.20 μm pore. The concentration of individual FPs such as Sr(II), Y(III), Zr(IV), Mo(VI), Ru(III), Pd(II), La(III), Ce(III), Nd(III), Sm(III), Eu(III), and Gd(III) in aqueous phase before and after adsorption was analyzed by inductively coupled plasma atomic emission spectroscopy (ICP-AES: Shimadzu ICPS-7510). The radioactivity of 241Am(III) was determined by HPGe-γ-detector (GEM70P-PLUS, Ortec). The individual radioactivity of 99Tc(VII) and 239Pu(IV) was measured by super low level liquid scintillation analyzer (PE Tri-Carb 3170). The concentration of U(VI) was measured by UV spectrophotometer (LabTech UV1000/1100).
The distribution coefficient (Kd, cm3 g–1) and the adsorption capacity (q, mmol g–1) were calculated by Equations Equation(1)–(3):
(1)
(1)
(2)
(2)
(3)
(3) where C0 (mmol dm–3) (A0 (Bq cm–3)) and Cf (mmol dm–3) (Af (Bq cm–3)) denote the metal ion concentration (radioactivity) in the aqueous phase before and after adsorption, respectively. V (cm3) and WR (g) indicate the volume of the aqueous phase and the mass of isoHex-BTP/SiO2-P, respectively.
Batch desorption experiment
In batch desorption experiments, the Pd(II) loaded isoHex-BTP/SiO2-P adsorbent was obtained by contacting isoHex-BTP/SiO2-P with a solution of Pd(II) in 3 mol dm–3 HNO3. Several eluents summarized in including water, diethylenetriaminepentaacetic acid (DTPA, HOOCCH2N[CH2CH2N(CH2COOH)2]2) and thiourea (SC(NH2)2) were employed for conducting the desorption experiments. An amount of 0.1 g loaded adsorbent was shaken with 5 cm3 of eluent solution with 120 rpm shaking speed at 298 K for 3 h. Then the adsorbent was separated from the aqueous solution. The concentration of Pd(II) in the solution was determined by ICP-AES. Desorption efficiency of Pd(II) was calculated by the following equation:
(4)
(4) where qd (mmol g–1) and qa (mmol g–1) are the desorption capacity from the loaded isoHex-BTP/SiO2-P adsorbent and the adsorption capacity of isoHex-BTP/SiO2-P adsorbent, respectively.
Table 1. Eluents used in batch desorption experiments.
Results and discussion
Adsorption studies
Selective adsorption of Pd(II)
HLLW contains various stable/radioactive FPs along with the actinides in appreciable quantities [Citation15]. And the nitric acid concentration in HLLW is about 3 mol dm−3. Therefore, to evaluate the efficacy of isoHex-BTP/SiO2-P in separation and recovery of Pd(II) from HLLW, batch adsorption experiment was carried out with 3 mol dm–3 nitric acid solution containing Pd(II) (1 mmol dm–3) and other FPs (individually 1 mmol dm–3), 241Am(III) (1000 Bq cm–3), 99TcO– 4 (1000 Bq cm–3), 239Pu(IV) (50 Bq cm–3) and UO2+ 2(1 mmol dm–3) for 24 h of contact. shows the distribution coefficients of various metal ions. The distribution coefficient of Pd(II) was more than Kd > 105 cm3 g−1 and almost 100% of Pd(II) was adsorbed from the solution. Sr(II), Y(III), Zr(IV), Mo(VI), Tc(VII), Ru(III), La(III), Ce(III), Nd(III), and U(VI) were hardly adsorbed by isoHex-BTP/SiO2-P, while Sm(III), Eu(III) (Kd Eu(III) = 78 cm3 g–1), and Gd(III) (Kd Gd(III) = 82 cm3 g–1) had fairly low distribution coefficients. The order of adsorbability was Pd(II) >> Pu(IV) > Am(III) >> Gd(III) > Eu(III) > Sm(III) and other FPs and U(VI). So isoHex-BTP/SiO2-P exhibited extremely higher selectivity and affinity for Pd(II) than other metal ions, even than Pu(IV) (Kd Pu(IV) = 20,483 cm3 g−1) and Am(III) (Kd Am(III) = 16,246 cm3 g−1). The high selectivity adsorption of Pd(II) is considered to result from the fact that Pd(II) being a well-known soft metal is strongly bound to ligands containing soft donor atoms like ‘N’ because the 4d orbitals of Pd(II) give rise to a good orbital overlap in bond formation with the soft ligands. isoHex-BTP as a soft ligand containing ‘N’, which coordinates with Pd(II) via N atom in the pyridine ring as well as via the triazinyl N atoms, shows the high affinity for Pd(II) [Citation22]. The distribution coefficient of Pd(II) is sufficiently high to enable the complete Pd(II) adsorption possible in the direct separation process as shown in . Furthermore, the adsorbed metal ions, i.e. Pu(IV), Am(III) and Ln(III) can be desorbed from loaded isoHex-BTP/SiO2-P with water or diluted nitric acid solution but Pd(II) could not [Citation18]. So the separation and recovery of Pd(II) could be achieved while Pu(IV) and Am(III) are being separated using isoHex-BTP/SiO2-P.
Effect of nitric acid concentration
The purpose of the present study is the application of isoHex-BTP/SiO2-P for the separation of Pd(II) from HLLW. As the nitric acid concentration in HLLW is about 3 mol dm−3, the effect of nitric acid concentration from 0.01 to 4 mol dm−3 on the adsorption was studied. An amount of 0.1 g isoHex-BTP/SiO2-P was equilibrated with 5 cm3 solution containing 20 mmol dm–3 Pd(II) at various initial nitric acid concentrations for 3 h of contact, and the results are shown in . isoHex-BTP/SiO2-P presented high adsorption performance for Pd(II) even at low nitric acid concentration. The adsorption capacity q and distribution coefficient Kd of Pd(II) increased with increasing nitric acid concentration. The acid dependence can be attributed to either the salting out effect due to increase in nitrate ion concentration or the participation of nitric acid molecule in the formation of metal complex [Citation23]. However, further studies are required to understand the reason for the increase adsorption with nitric acid concentration. The adsorption of Pd(II) almost reached a maximum and the adsorption capacity was 0.68 mmol g–1 at nitric acid concentration of 3 mol dm–3, which suggests a highly favored recovery of Pd(II) from HLLW.
Figure 4. Effect of initial nitric acid concentration on q and Kd of Pd(II) adsorbed by isoHex-BTP/SiO2-P (298 K, phase ratio: 0.1 g/5 cm3, initial Pd(II) concentration: 20 mmol dm-3, shaking speed: 120 rpm, contact time: 3 h).
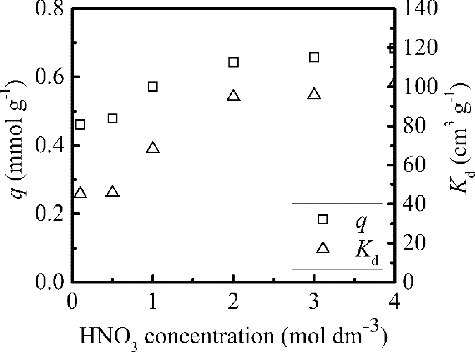
According to the nitric acid concentration in HLLW and the experimental data shown in , 3 mol dm–3 HNO3 was chosen to investigate the adsorption of Pd(II) at 298 K in the following study.
Adsorption kinetics
To determine the equilibrium time for the adsorption, Pd(II) (initial concentration: 20 mmol dm–3) adsorption from 3 mol dm–3 HNO3 as a function of contact time by isoHex-BTP/SiO2-P was studied at 298 K. The results are shown in . The adsorption capacity qt increased with increasing contact time t. It seems as if Pd(II) uptake occurred in two steps. Adsorption occurred rapidly within the initial 2 h, coming in a steady state after 24 h, after which no remarkable increase in qt was observed. And 57%, 71%, and 83% of the equilibrium adsorption capacity (qe,exp = 0.84 mmol g–1) of Pd(II) occurred within the first 0.5, 2 and 10 h, respectively. The sudden increase of qt at the very beginning of the process is attributed to an abundant availability of active sites on internal and external surface area of isoHex-BTP/SiO2-P/SiO2-P. With the progressive occupancy of these sites by Pd(II), the process comes into a period of slower adsorption, during which the less accessible sites can be occupied [Citation24]. However, the adsorption of Pd(II) by isoHex-BTP/SiO2-P was rather slow and reached almost an equilibrium state at 24 h.
Figure 5. Effect of contact time on the adsorption capacity (qt) of Pd(II) by isoHex-BTP/SiO2-P (298 K, initial HNO3 concentration: 3 mol dm–3, initial Pd concentration: 20 mmol dm–3, phase ratio: 0.1 g/5 cm3, shaking speed: 120 rpm).
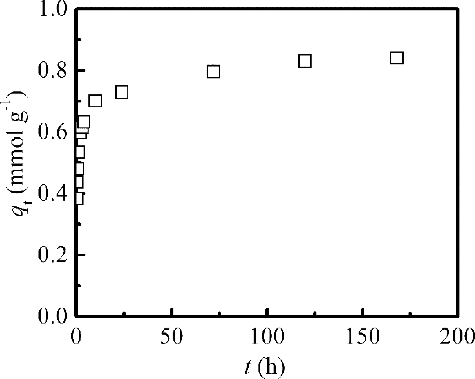
The experimental data were further analyzed using the pseudo-first-order, pseudo-second-order, intra-particle diffusion and Elovich kinetic models to examine the mechanism of adsorption potential rate controlling steps such as mass-transport, and chemical reaction processes of the adsorption of Pd(II) by isoHex-BTP/SiO2-P.
The pseudo-first-order kinetic model is based on the approximation that the adsorption rate relates to the number of the unoccupied, adsorptive sites. The linear form of the model can be expressed as Equation (Equation5(5)
(5) ) [Citation25]:
(5)
(5)
Experimental data were also applied to the pseudo-second-order kinetic model which is based on the assumption that chemisorption is the rate-determining step of adsorption process. The linear form of the model can be expressed as EquationEquation(6)(6)
(6) [Citation26,Citation27]:
(6)
(6)
The intra-particle diffusion model is also commonly used to characterize the adsorption data. In order to test the contribution of intra-particle diffusion on the adsorption process, the rate constant for intra-particle diffusion model is obtained by using Equation (Equation7(7)
(7) ) [Citation28]:
(7)
(7)
The equation defining the Elovich kinetic model is based on a kinetic principle assuming that the adsorption sites increase exponentially with adsorption, which implies a multilayer adsorption. The linear form of Elovich equation is given by the following Equation [Citation29]:
(8)
(8)
In Equations (Equation5(5)
(5) )–(Equation8
(8)
(8) ), qe (mmol g–1) and qt (mmol g–1) are the adsorption capacity at equilibrium and at any time t (h). k1 (h−1) is the adsorption rate constant of the pseudo-first-order kinetic model. k2 (g mmol–1 h–1) is the adsorption rate constant for the pseudo-second-order kinetic model. C is the intercept and ki (mmol g–1 h–1/2) is the intra-particle diffusion rate constant. α and β known as the Elovich coefficients, α (mmol g–1 h–1) represents the initial adsorption rate, and β (g mmol–1) is the desorption constant, respectively.
The linear plots of ln (qe-qt) versus t for the pseudo-first-order kinetic model, t/qt versus t for the pseudo-second-order kinetic model, qt versus t1/2 for the intra-particle diffusion model and qt versus lnt for the Elovich kinetic model for the adsorption of Pd(II) by isoHex-BTP/SiO2-P were drawn to obtain the adsorption kinetic parameters. The kinetic parameters of Pd(II) were calculated from these plots and are summarized in .
Table 2. The adsorption kinetic model parameters for Pd(II) adsorption by isoHex-BTP/SiO2-P at 298 K (initial HNO3 concentration: 3 mol dm–3, initial Pd(II) concentration: 20 mmol dm–3, phase ratio: 0.1 g/5 cm3, shaking speed: 120 rpm).
As can be seen from , the correlation coefficient (R22 = 0.999) for the pseudo-second-order kinetic model was much higher than that for the pseudo-first-order kinetic model (R21 = 0.765), the intra-particle diffusion model (R2I = 0.778), and Elovich kinetic model (R2E = 0.987). So it can be inferred that adsorption of Pd(II) by isoHex-BTP/SiO2-P fits well the pseudo-second-order kinetic model as compared to the other three models. The calculated equilibrium adsorption capacity (qe,calc = 0.84 mmol g−1) from the pseudo-second-order kinetic model is equal to the experimental one qe,exp. The results support the assumption that the rate-limiting step of Pd(II) adsorption may be chemisorption process involving valence forces through electron sharing between N atom in the pyridine as well as N atoms in triazinyl of isoHex-BTP and 4d orbital of Pd(II).
Adsorption isotherms
The adsorption capacity is determined as a function of the equilibrium concentration at a constant temperature that could be explained in adsorption isotherms. Adsorption isotherm of Pd(II) in was determined by shaking a fixed mass of 0.1 g isoHex-BTP/SiO2-P with 5 cm3 Pd(II) solution with the initial Pd(II) concentration ranging from 5 to 50 mmol dm−3 for constant contact time (t = 72 h, to obtain the complete equilibrium state) at 298 K. As shown in , the adsorption capacity of Pd(II) increased as the equilibrium concentration of Pd(II) in solution increased, then reached a steady stage which finally reached the maximum adsorption capacity (qm,exp = 0.85 mmol g–1).
Figure 6. Adsorption isotherm of Pd(II) by isoHex-BTP/SiO2-P at 298 K (initial HNO3 concentration: 3 mol dm–3, phase ratio: 0.1 g/5 cm3, shaking speed: 120 rpm).
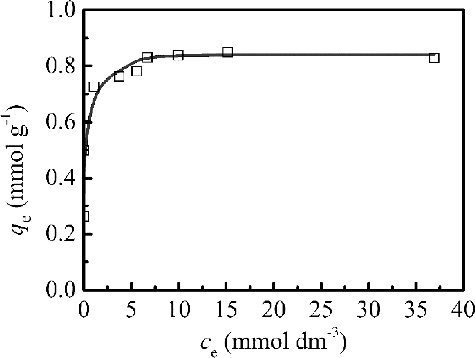
The equilibrium data for Pd(II) adsorption were analyzed with the most common adsorption isotherm models, the Freundlich and Langmuir isotherm model, to reveal the adsorption mechanism.
The Freundlich isotherm model is used to describe the adsorption of an adsorbate on a heterogeneous surface with adsorption sites that have different energies of that adsorption. The linear form of the model is given as Equation (Equation9(9)
(9) ) [Citation27,Citation30]:
(9)
(9) where KF (mmol g–1) is a constant related to the maximum adsorption capacity and nF (dimensionless) is the heterogeneity factor which has a lower value for more heterogeneous surfaces.
The Langmuir isotherm model is based on the assumption that adsorption on a homogeneous surface possessing identical sites equally available for adsorption and with equal energies of adsorption involves with the adsorbent being saturated after one layer of adsorbate molecules has formed on the surface [Citation27]. The linear form of the model is represented by Equation (Equation10(10)
(10) ) [Citation29]:
(10)
(10) where qe (mmol g–1) is the adsorption capacity at equilibrium, Ce (mmol dm–3) is the equilibrium concentration of an adsorbate in solution, qm (mmol g–1) is the maximum adsorption capacity to form a monolayer onto an adsorbent surface, KL (dm3 mmol–1) is Langmuir adsorption constant related to the free energy of adsorption. The fundamental characteristic of a Langmuir isotherm parameter (RL) can be expressed in terms of a dimensionless separation factor or an equilibrium parameter, which is defined by Equation (Equation11
(11)
(11) ) [Citation25]:
(11)
(11) where C0 (mmol dm−3) is the initial concentration of an adsorbate. According to the value of RL, the shape of the isotherm may be interpreted as follows: RL > 1: unfavorable adsorption, RL = 1: linear adsorption, 0 < RL < 1: favorable adsorption, and RL = 0: irreversible adsorption.
The linear plots of log qe versus log Ce for the Freundlich model, Ce/qe versus Ce for the Langmuir model for the adsorption of Pd(II) by isoHex-BTP/SiO2-P have been drawn to obtain the isotherm parameters from the slopes and the intercepts of the linear plots. And the isotherm parameters of Pd(II) are reported in . As can be seen, the correlation coefficient (R2 = 1.000) for the Langmuir model are much higher than that for the Freundlich model (R2 = 0.903) at 298 K. The calculated maximum adsorption capacity (qm,calc = 0.89 mmol g–1) from Langmuir model was very close to the experimental one qm,exp (qm,exp = 0.85 mmol g–1). The results indicate that the adsorption equilibrium data fit much better to the Langmuir model than the Freundlich model, which means that adsorption of Pd(II) occurs on a homogeneous surface by monolayer adsorption and each adsorptive site of isoHex-BTP/SiO2-P can be occupied by Pd(II) only once in a one-on-one manner. The value of RL (0 < RL < 1) indicates that the adsorption of Pd(II) by isoHex-BTP/SiO2-P is favorable.
Table 3. The Langmiur and Freundlich isotherm parameters for Pd(II) adsorption by isohexyl-BTP/SiO2-P at 298 K.
Desorption studies
After the process of adsorption, desorption of Pd(II) from loaded adsorbent is of crucial importance in assessing the potential of adsorbent for commercial applications. So the effects of different eluents, the concentration of thiourea (SC(NH2)2) as eluent, desorption time on the desorption efficiency of Pd(II) were examined by batch desorption method to optimize the desorption conditions. Several eluents including water, DTPA, and thiourea were employed for conducting the desorption experiments. shows Pd(II) desorption efficiency with various eluents. Significant desorption was not observed in water, DTPA solution with or without HNO3. Pd(II) can be efficiently eluted from loaded adsorbent by 0.5 mol dm–3 SC(NH2)2 - 0.1 mol dm–3 HNO3 mixed solution or 0.5 mol dm–3 SC(NH2)2. 0.5 mol dm–3 SC(NH2)2 - 0.1 mol dm–3 HNO3 mixed solution and 0.5 mol dm–3 SC(NH2)2 were made by dissolving SC(NH2)2 into 0.1 mol dm–3 HNO3 solution and the deionized water, respectively. And 0.5 mol dm–3 SC(NH2)2 - 0.1 mol dm–3 HNO3 is the most efficient eluent for Pd(II) desorption among these eluents by forming the complexe [Pd(SC(NH2)2)4](NO3)2 [Citation31], and more than 90% Pd(II) was desorbed. shows that increasing in the SC(NH2)2 concentration has significant effect on the desorption efficiency of Pd(II). The optimal SC(NH2)2 concentration in mixed solution with 0.1 mol dm–3 HNO3 is 0.5 mol dm–3 and the yield of Pd(II) to the eluate is 92%. In further series of experiments, the effect of desorption time was studied. shows that Pd(II) desorption efficiency increased with increasing desorption time. Hundred per cent Pd(II) was eluted at 6 h of desorption time. However, desorption kinetics was slow and further study is necessary for improving desorption rate.
Figure 7. Effect of different eluents on desorption efficiency from loaded isoHex-BTP/SiO2-P (adsorption capacity of loaded adsorbent: 0.65 mmol g–1 Pd(II), desorption conditions: phase ratio: 0.1 g/5 cm3, shaking speed: 120 rpm, 298 K, desorption time: 3 h) ( (a) H2O, (b) 0.01 mol dm–3 DTPA - 0.01 mol dm–3 HNO3, (c) 0.01 mol dm–3 DTPA, (d) 0.5 mol dm–3 SC(NH2)2 - 0.1 mol dm–3 HNO3, and (e) 0.5 mol dm–3 SC(NH2)2 ).
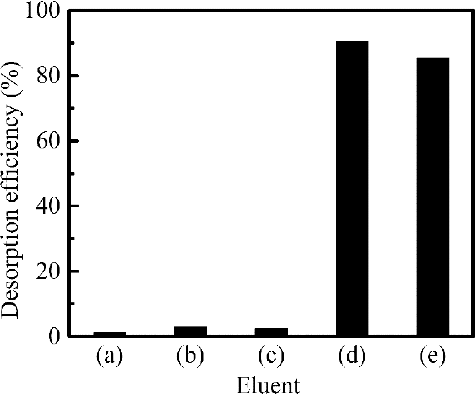
Figure 8. Effect of the concentration of SC(NH2)2 on the desorption efficiency from loaded isoHex-BTP/SiO2-P (adsorption capacity of loaded adsorbent: 0.65 mmol g–1 Pd(II), desorption conditions: initial HNO3 concentration: 0.1 mol dm–3, phase ratio: 0.1 g/5 cm3, 298 K, desorption time: 3 h, shaking speed: 120 rpm).
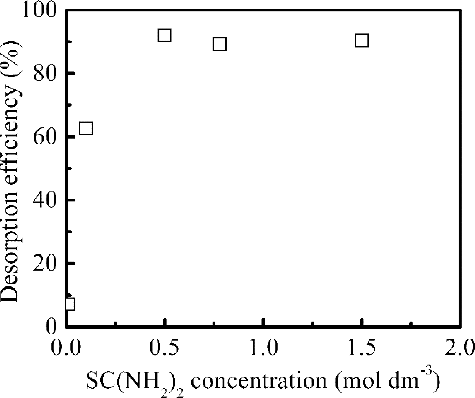
Figure 9. Effect of the desorption time on the Pd(II) desorption efficiency from loaded isoHex-BTP/SiO2-P (adsorption capacity of loaded adsorbent: 0.65 mmol g–1 Pd(II), desorption conditions: eluent: 0.5 mol dm–3 SC(NH2)2 - 0.1 mol dm–3 HNO3, phase ratio: 0.1 g/5 cm3, 298 K, shaking speed: 120 rpm).
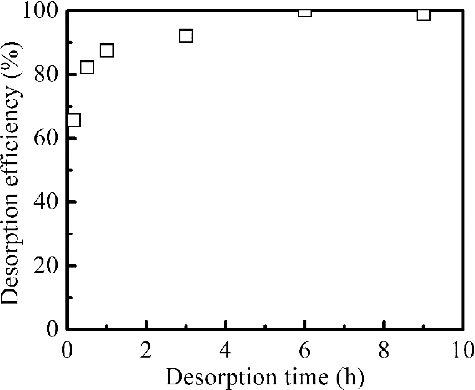
Conclusions
Within the scope of this work, isoHex-BTP/SiO2-P adsorbent exhibited remarkable selectivity and affinity for Pd(II) over other typical FPs and even over Am(III) and Pu(IV) present in HLLW. Many factors such as nitric acid concentration, contact time, and the concentration of Pd(II) had strong impacts on adsorption of Pd(II) by isoHex-BTP/SiO2-P from nitric acid solution at 298 K. Pd(II) adsorption from 3 mol dm–3 HNO3 depending on contact time fits much better to the pseudo-second-order kinetic model with high correlation coefficient (R2 = 0.999) than the pseudo-first-order, intra-particle diffusion and Elovich kinetic models, indicating that Pd(II) adsorption by isoHex-BTP/SiO2-P occurs by chemisorption mechanism and the coordination reaction between isoHex-BTP/SiO2-P and Pd(II) is the rate-controlling step of the adsorption process. The equilibrium adsorption data follow much better Langmuir model than Freundlich model, which means that the adsorption of Pd(II) occurs on a homogeneous surface of isoHex-BTP/SiO2-P and each adsorptive site of isoHex-BTP/SiO2-P can be occupied by Pd(II) only once in a one-on-one manner. Desorption studies showed that a quantitative recovery of Pd(II) was possible with 0.5 mol dm–3 SC(NH2)2 - 0.1 mol dm–3 HNO3 as eluent, but the desorption rate was slow.
These results demonstrate that isoHex-BTP/SiO2-P is a promising adsorbent for the direct separation of Pd(II), meanwhile Am(III) and residual Pu(IV) are separated using isoHex-BTP/SiO2-P from other typical elements present in HLLW. Through this study, essential adsorption kinetics, adsorption isotherm and desorption data were collected for an engineering design of the column separation of Pd(II) from HLLW by isoHex-BTP/SiO2-P. In addition, more investigations regarding the adsorption mechanism desorption kinetics, column separation experiments, and the reuse of a series of BTP/SiO2-P adsorbents are under the way.
Acknowledgments
This work was supported by National Natural Science Foundation of China under grant number [11305102 ] and [11675103].
Disclosure statement
No potential conflict of interest was reported by the authors.
Additional information
Funding
References
- Pokhitonov YA, Romanovskii VN. Palladium in irradiated fuel. Are there any prospects for recovery and application? Radiochemistry 2005;47(1):1–13.
- Giridhar P, Venkatesan KA, Srinivasan TG, et al. Extraction of fission palladium by Aliquat 336 and electrochemical studies on direct recovery from ionic liquid phase. Hydrometallurgy 2006;1:30–39.
- Raj MM, Dharmaraja A, Panchanatheswaran K, et al. Extraction of fission palladium(II) from nitric acid by benzoylmethylenetriphenylphosphorane (BMTPP). Hydrometallurgy 2006;1–2:118–124
- Venkatesan KA, Selvan BR, Antony MP, et al. Extraction of palladium from nitric acid medium by commercial resins with phosphinic acid, methylene thiol and isothiouronium moieties attached to polystyrene-divinylbenzene. J Radioanal Nucl Chem. 2005;3:431–440.
- Ruhela R, Singh KK, Tomar BS, et al. Amber lite XAD-16 functionalized with 2-acetyl pyridine group for the solid phase extraction and recovery of palladium from high level waste solution. Sep Purif Technol. 2012;99:36–43.
- Leng YX, Xu J, Wei JC, et al. Amino-bearing calixcrown receptor grafted to micro-sized silica particles for highly selective enrichment of palladium in HNO3 media. Biochem Eng J. 2013;232:319–326.
- Kolarik Z, Renard EV. Recovery of value fission platinoids from spent nuclear fuel PART II: SEPARATION PROCESSES. Platin Met Rev. 2003;3:123–131.
- Ruhela R, Sharma JN, Tomar BS, et al. N,N,N ',N'-Tetra(2-Ethylhexyl) thiodiglycolamide T(2EH)TDGA: a promising ligand for separation and recovery of palladium from high level liquid waste (HLLW) solutions. Sep Sci Technol. 2011;6:965–971
- Lee SH, Chung H. Ion exchange characteristics of palladium and ruthenium from a simulated radioactive liquid waste. Sep Sci Technol. 2003;14:3459–3472.
- Korolev VA, Pokhitonov YA, Gelis VM, et al. Recovery of Pd from spent fuel: 2. Sorption recovery of Pd from nitric acid solutions on anion-exchange resins. Radiochemistry 2005;4:370–373.
- Jayakumar M, Venkatesan KA, Srinivasan TG, et al. Electrochemical recovery of fission platinoids (Ru, Rh, Pd) from simulated high-level liquid waste. Desalination Water Treat. 2012;1–3:73–78.
- Jayakumar M, Venkatesan KA, Srinivasan TG, et al. Feasibility studies on the electrochemical recovery of fission platinoids from high-level liquid waste. J Radioanal Nucl Chem. 2010;1:79–85.
- Xu YL, Kim SY, Ito T, et al. Adsorption properties and behavior of the platinum group metals onto a silica-based (Crea + TOA)/SiO2-P adsorbent from simulated high level liquid waste of PUREX reprocessing. J Radioanal Nucl Chem. 2013;1:41–48.
- Parajuli D, Hirota K. Recovery of palladium using chemically modified cedar wood powder. J Colloid Interface Sci. 2009;2:371–375.
- Wilden A, Modolo G, Schreinemachers C, et al. Direct selective extraction of actinides (III) from PUREX Raffinate using a mixture of CyMe4BTBP and TODGA as 1-cycle SANEX Solvent Part III: demonstration of a laboratory-scale counter-current centrifugal contactor process. Solvent Extr Ion Exch. 2013;5:519–537.
- Panak PJ, Geist A. Complexation and extraction of trivalent actinides and lanthanides by triazinylpyridine N-Donor Ligands. Chem Rev. 2013;2:1199–1236.
- Liu RQ, Ning SY, Wang XP, et al. Adsorption behavior of actinides and some typical fission products by silica/polymer-based isoHex-BTP adsorbent from nitric acid solution. J Radioanal Nucl Chem. 2014;1:681–691.
- Liu RQ, Wei YZ, Xu YL, et al. Evaluation study on properties of isohexyl-BTP/SiO2-P resin for direct separation of trivalent minor actinides from HLLW. J Radioanal Nucl Chem. 2012;2:537–544.
- Wei YZ, Zhang AY, Kumagai M, et al. Development of the MAREC process for HLLW partitioning using a novel silica-based CMPO extraction resin. J Nucl Sci Technol. 2004;3:315–322.
- Wei YZ, Sabharwal KN, Kumagai M, et al. Preparation of novel silica-based nitrogen donor extraction resins and their adsorption performance for trivalent americium and lanthanides. J Nucl Sci Technol. 2000;12:1108–1110.
- Wei YZ, Hoshi H, Kumagai M, et al. Separation of Am(III) and Cm(III) from trivalent lanthanides by 2,6-bistriazinylpyridine extraction chromatography for radioactive waste management. J Alloys Compd. 2004;1–2:447–450.
- Guibal E, Sweeney NV, Vincent T, et al. Sulfur derivatives of chitosan for palladium sorption. React Funct Polym. 2002;2:149–163.
- Ruhela R, Tomar BS, Sharma JN, et al. Studies on the separation and recovery of palladium from simulated high level liquid waste (SHLW) solution with novel extractant N,N,N',N'-tetra(2-ethylhexyl) dithiodiglycolamide DTDGA. Sep Sci Technol. 2013;7:1049–1055.
- Bozic D, Stankovic V, Gorgievski M, et al. Adsorption of heavy metal ions by sawdust of deciduous trees. J Hazard Mater. 2009;1–3:684–692.
- Javadian H, Ghaemy M, Taghavi M. Adsorption kinetics, isotherm, and thermodynamics of Hg2+ to polyaniline/hexagonal mesoporous silica nanocomposite in water/wastewater. J Mater Sci. 2014;1:232–242.
- Hanafiah M, Zakaria H, Ngah WSW. Preparation, characterization, and adsorption behavior of Cu(II) ions onto alkali-treated weed (imperata cylindrica) leaf powder. Water Air Soil Pollut. 2009;1–4:43–53.
- EMÖ Kaya, Özcan AS, Ö Gök, et al. Adsorption kinetics and isotherm parameters of naphthalene onto natural- and chemically modified bentonite from aqueous solutions. Adsorption 2013;2–4:879–888.
- Salam MA. Removal of heavy metal ions from aqueous solutions with multi-walled carbon nanotubes: kinetic and thermodynamic studies. Int J Environ Sci Technol. 2013;4:677–688.
- Kaya EMO, Ozcan AS, Gok O, et al. Adsorption kinetics and isotherm parameters of naphthalene onto natural- and chemically modified bentonite from aqueous solutions. Adsorpt-J Int Adsorpt Soc. 2013;2–4:879–888.
- Dikici H, Saltali K, Bingolbali S. Equilibrium and kinetics characteristics of copper (II) sorption onto gyttja. Bull Environ Contam Toxicol. 2010;1:147–151.
- Shusharina EA, Druzhinina IA, Tatarchuk VV, et al. (2010) Crystal structure of [Rh(Thio)6](NO3)3·3H2O and [Pd(Thio)4](NO3)3·3H2O thiourea complexes - products of extraction tretament of rhodium(III) and palladium(II) nitrate-nitrite solutions. J Struct Chem. 2010;51(5):987–990.