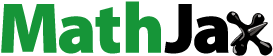
ABSTRACT
In the operation of the sodium-cooled fast reactor, the accident caused by the leakage and combustion of liquid sodium is common and frequent. In this paper, the combustion and spatial temperature distribution characteristics at the different distances from the center of droplet and the different angles (0–30°) were studied by carrying out the experiments of the oxidation and combustion under initial temperatures (200–350°C) of sodium droplet, oxygen concentrations (12–21%) and ambient atmosphere temperatures (80°C and 180°C). The experiment results show that the ambient atmosphere temperature cannot change the three stages (surface oxidation, preignition, and combustion) of sodium droplet combustion but it will change the characteristics of every stage. When the temperature of sodium droplet excesses the characteristic temperature (600°C), the temperatures of all spatial measuring points begin to rise noticeably. The peak temperature of the sodium droplet that completely burned could roughly reach 580–1000°C. The distributions of the spatial maximum temperature in three angle directions are in conformity with exponential function as the distance increases. The study provides the technical support on the evaluation and analysis of various forms of sodium fire accidents.
1. Introduction
The sodium-cooled fast reactor (SFR) is a more realistic type of reactor in the fourth-generation advanced reactors. Liquid sodium is used as a coolant in SFR for its more excellent thermodynamical properties than the water, including the higher thermal conductivity, higher boiling point, and smaller neutron absorption cross section that can improve the utilization of uranium [Citation1,Citation2]. However, the particular disadvantage of liquid sodium is its high chemical reactivity with the atmosphere [Citation3]. At present, the accidents caused by the leakage and combustion of liquid sodium are common and frequent in sodium-related facilities. Many serious sodium leakages and accidents have occurred and cause long-time shutdown, including British prototype reactor, the French Super-Phoenix, and Japan Monju reactor [Citation4,Citation5]. Typically, the sodium fire is the key and difficult research field in the international fast reactor safety analysis [Citation6]. It can be seen that the research on the sodium fire is the basis for research, design, and operation of SFR.
At present, some theoretical and experimental studies have been carried out on the sodium fire around the world. However, there are only a few studies on the experiments of the sodium droplet combustion. Richard and Tsai [Citation7,Citation8] have studied the combustion of static sodium droplet. They found that the sodium droplet combustion follows the D2-law and the combustion of sodium droplet could be divided into two stages: preignition stage and post-combustion stage. In 1983, Newman [Citation9] carried out some experiments on the sodium droplet combustion and found that the sodium combustion is gas-phase combustion. In 2003, Makino [Citation10] studied the relationship between the delay time, critical conditions of ignition, and the initial temperature, size of sodium droplet. In 2005, Makino and Fukada [Citation11] found that the obvious factors affecting the ignition temperature of sodium droplet are the initial temperature, the diameter of sodium droplet, oxygen concentration, and the relative speed by experimentally studying the combustion characteristics of free-fall sodium droplet. Nishimura [Citation12] conducted some preliminary studies on the oxidative combustion and recombustion of sodium droplet, and analyzed the effect of columnar oxides in combustion. Recently, Zhang et al. [Citation13–Citation15] carried out several experiments of sodium columnar fire to study the composition, combustion characteristics, and fire extinguishing measures of sodium columnar fire; and a series of experiments about sodium droplet in high ambient temperature to study its combustion characteristics, including the self-ignition and combustion of droplet under different oxygen concentration, droplet surface characteristics and distributions of spatial temperature. In the present study, the lumped parameter method is widely used for the treatment of ambient atmosphere temperature, and few experiments focus on the impact of ambient atmosphere temperature on the sodium droplet combustion and the distribution characteristics of spatial temperature. However, the existing research conditions are not systematic and lack of detailed experimental data. Therefore, it is great significance to study the combustion characteristics of sodium droplet.
In this paper, the experiments were carried out under the conditions of different initial temperatures (200–350°C) of the sodium droplet, oxygen concentrations (12–21%), and initial ambient temperatures (80°C and 180°C) to investigate the sodium droplet combustion characteristics including the combustion mechanism and the distribution characheristics of spatial temperature at different angles (0°, 15°, and 30°) and different distances (10, 20, and 40 mm). These studies may improve the simulation accuracy of the sodium column fire and spray fire and provide technical support for the safety evaluation and prevention measures on the different forms of the sodium fire accidents.
2. Experiments
2.1. Experimental apparatus and procedure
The experimental apparatus consists of a droplet combustion device, a glove box, the equipment for photographing the combustion process, and a gas supply system. A schematic view of the experimental apparatus is shown in .
Figure 1. Experimental apparatus.
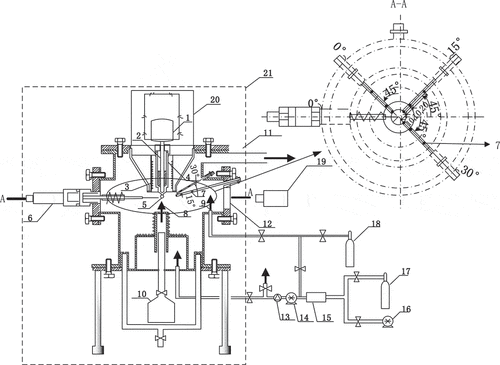
The combustion vessel consists of two dependent chamber components. In the upper chamber, there is a crucible with a nozzle to form the sodium droplet, and an electric wire incorporate around the crucible to preheat the sodium. A piston for extruding liquid sodium is above the crucible. In addition, a motor is mounted on the upper flange to move the piston up and down. A tube is set under the nozzle for the gas supply. The viewing port is positioned at the height of the nozzle for observing liquid sodium droplet. The organic glass container makes the crucible in the shielding gas to prevent the sodium from oxidizing. The lower chamber is the combustion chamber. A thermocouple (outside sheath diameter: 0.5 mm) is installed under the nozzle and could move in the horizontal direction to contact with the droplet to measure the initial temperature and the combustion temperature of the sodium droplet. At the same time, nine thermocouples are set for measuring the spatial temperature at the angle of 0°, 15°, and 30° and in the distance of 10, 20, and 40 mm from the center of the sodium droplet. All the thermocouples are connected to a data sampling board (HIOKI-LR8402 E.E). The precision of the data sampling board is ±0.8°C, and the minimum data acquisition interval is 10 ms. The measurement precision of the thermocouple is ±0.75°C between 0 and 800°C. Under the lower chamber, a heating wire is provided to preheat the ambient atmosphere and a thermocouple is set to measure the mixed ambient gas temperature. The mixed gas of arbitrary oxygen fraction could be supplied by mixing nitrogen with the air (oxygen: 21%). This combustion vessel is located in a glove box, where the oxygen and moisture fractions could be controlled accurately.
When the oxygen concentration in the glove box was lower than a predetermined value. The sodium was then heated to a prescribed temperature. A liquid sodium droplet was formed at the tip of the nozzle through the slow depression of the piston, and the horizontal diameter of the sodium droplet was controlled to be around 3–5 mm. The tip of the thermocouple was positioned at the edge of sodium droplet when the temperature of sodium was stabilized. The mixed gas was adjusted to the prescribed fraction of oxygen, and the flow rate was set to 650ml/min at the bypass line outlet. The mixed gas was supplied into the combustion chamber. The video images were recorded by the high-speed video camera at a frame rate of 100 fps.
2.2 Experimental conditions
This experiment was mainly to study the spatial temperature distribution characteristics and investigate the effect of ambient atmosphere temperature on the sodium droplet oxidation, ignition, and combustion. In order to study the droplet combustion characteristics at different temperatures, according to the environmental temperature of column flow fire and spray fire [Citation13,Citation15], the ambient atmosphere temperature was set to 80°C and 180°C, as shown in and . The moisture was maintained to be about 17% in all experiments in this paper. At 0 s, the examination gas was led into the combustion chamber.
Table 1. Experimental conditions (ambient atmosphere temperature 180°C)
Table 2. Experimental conditions (ambient atmosphere temperature 80°C)
3. Experimental results and analysis
3.1. The influence of the ambient atmosphere temperature on sodium droplet combustion
shows the combustion process of sodium droplet under different conditions (Case5c, Case1c [Citation14], Case7c, and Case3c [Citation14]). During sodium droplet combustion, several chemical reactions may take place according to the following equations. EquationEquations (1)(1)
(1) and (Equation2
(2)
(2) ) are the main reactions, and only if the sodium is excessive, EquationEquations (5)
(5)
(5) and (Equation6
(6)
(6) ) would take place.
It could be found that the process of sodium droplet combustion under different ambient atmosphere temperatures (80°C and 180°C) can be divided into three stages. Surface oxidation stage: the droplet surface was covered with a white oxide layer in 3–5 s after the droplet gets into contact with oxygen, and the temperature of droplet would rise slightly (about 40°C) because of oxidation; however, there was no deformation to be observed; preignition stage: because of the heat released by the oxidation reaction, the temperature of the droplet rose and the volume expanded. Affected by the surface tension and oxides, the droplet was out of shape and started to wrinkle and sink. Although the flame cannot be observed at this stage, the temperature of droplet would rise continually and approach the ignition point; combustion stage: the temperature of droplet rose rapidly and got the ignition point, after that, the droplet started burning severely and released bright orange flame. The peak of droplet temperature was also detected in this stage.
The ambient atmosphere temperature has effects on the combustion characteristics of each stage. As can be seen from , when the ambient atmosphere temperature was 80°C, the droplet surface was covered with a white oxide layer, accompanied by obvious dendritic oxide for a long time. The temperature of sodium droplet first increased slightly until reaching the ignition point, after that, sodium droplet started burning and the temperature rose rapidly. In this condition, both the preignition stage and the combustion stage lasted longer than these in higher ambient atmosphere temperature. When ambient atmosphere temperature was 180°C, the oxide layer formed on the surface of the sodium droplet was not obvious and not accompanied by dendritic oxide. At about 10 s after the oxidant supplied, the droplet started burning and giving off bright flame, but the time of combustion stage was much shorter.
shows the temperature distribution of sodium droplet under different ambient atmosphere temperatures (80°C and 180°C) but under the same initial sodium temperature and oxygen concentration. Four groups of experiment (Case6b and Case2b, Case6c and Case2c, Case8b and Case4b, and Case8c and Case4c) are selected to analyze the effect of the ambient atmosphere temperature on the sodium droplet combustion. It could be observed from (a–d) that when the ambient atmosphere temperature is 80°C, the sodium droplet temperature rises slightly at first, then a sudden rise can be observed in the preignition stage and the sodium begins to burn accompanied by a temperature peak. However, when the ambient atmosphere temperature is 180°C, the droplet temperature rises immediately and starts burning. This is probably because the droplet surface under low temperature is covered with a thick oxide layer that prevents further oxidation, and the ambient atmosphere temperature would take more heat from the surface of droplet. When the initial temperature is high (350°C), the temperature of sodium rises suddenly and rapidly. This is probably because that the sodium droplet reaches the ignition point immediately after the ambient atmosphere supplied.
Figure 3. Combustion temperature distributions under different the ambient atmosphere temperatures.
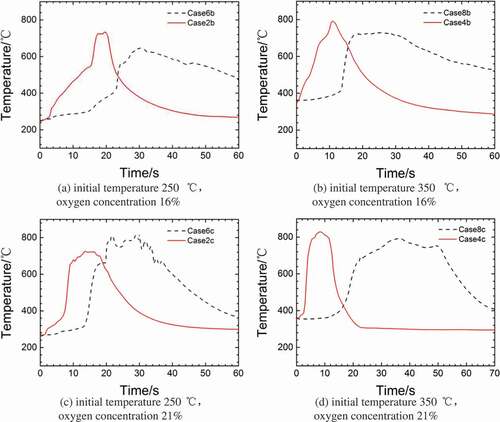
It can be observed from that the peak temperatures of sodium droplet are all relatively close and the temperature difference is in 100°C, even though under different ambient atmosphere temperatures. It illustrates that the ambient atmosphere temperature has little effect on the peak temperature in combustion. Moreover, it can be obviously observed from (a–d) that when the ambient atmosphere temperature is lower, the process of combustion would last longer. This is because sodium droplet would burn much severely in high ambient atmosphere temperature, which could be observed clearly from . On the other hand, shows that the oxide layer in Case5c and Case7c was thicker compared with Case1c [Citation14] and Case3c [Citation14]. The combustion was less severe in the low ambient atmosphere temperature; this is probably because the thicker oxide layer would reduce the contact area between the droplet and ambient atmosphere. In addition, when the ambient atmosphere temperature is higher, more sodium would gasify in the surface oxidation stage and preignition stage. However when the ambient gas temperature is lower, the thicker oxide layer would slow down the gasification, and the combustion stage would last longer because the quality of sodium droplet is larger.
3.2. The spatial temperature distribution in oxidation and combustion of sodium droplet
3.2.1. Spatial temperature distribution with the combustion process
shows the spatial temperature distribution when oxygen concentration is 21% and initial temperature is 250°C. It could be observed from that the temperature of droplet rises gradually at about 6 s after the droplet gets contact with gas because of oxidation. The flame could be observed at 10 s and the temperature of droplet rises rapidly. The peak of temperature is about 730°C at 18 s, and the combustion lasts for 10 s. However, the spatial temperature begins to rise at about 12 s. Comparing the temperature of the sodium droplet and the spatial measuring points, we found that the latter changes much later, but they reach the peak almost at the same time. It could be observed from that the spatial temperature begins to change observably after the droplet temperature excesses 600°C. When the droplet temperature excesses 600°C, the temperature of measuring point begins to rise observably; this is probably because the heat of measuring point that absorbs from radiation of sodium droplet is more than that taken away to the ambient by convection. It could be observed from that the temperature of the droplet and the spatial measuring points drop rapidly after burning. When the droplet temperature drops down to below 600°C, the heat received is less than that taken away and the measuring point temperature tends to be steady. We could observe these phenomena in most of other experiments. Therefore, the the droplet temperature of 600°C is very important for the change of spatial temperature under the experimental conditions. When droplet temperature is under 600°C, the spatial temperature changes slightly or remains stable, and when droplet temperature exceed 600°C, the spatial temperature changes obviously. It could be speculated that this characteristic temperature could be observed in the practical column flow fire and spray fire accidents, but its numerical value would change with the flow velocity and temperature of the airflow in the space. As shown in , the change of spatial temperature is very severe at 10 mm from the center of droplet, but the change at 20 mm is only about 20°C. However, the spatial temperatures almost have no change at 40 mm. This is because the solid angle of the measuring point at 40 mm from droplet is only one-fourth of that at 20 mm, and one-sixth of that at 10 mm. The bigger the distance from the droplet is, the smaller the solid angle of the measuring points is, and the less the radioactive heat transfer from droplet.
shows the spatial temperature field obtained through linear interpolation from the data in under different moments. The spatial temperature would gradually change as the sodium droplet burned. The spatial temperature changes greatly at 0–10 mm from the center of droplet, but the change is relatively flat at 10–40 mm. It can be observed from and that the spatial temperature at the angle of 0° is higher than those at the angle of 15° and 30°. This is probably because the ambient atmosphere first contacts with the bottom surface of the droplet after the ambient atmosphere supplied into the combustion chamber and the oxygen concentration is highest there. On the other hand, the contact area between droplet and ambient atmosphere also increases because of their relative velocity. The combustion in upper part of droplet is not violent because the amount of oxygen is consumed in the updraft and mixed with the gaseous combustion products.
3.2.2. Spatial temperature distribution in different angle directions
shows the distribution of the maximum spatial temperatures and fitting curves at different distances from the center of droplet and different angles (Case1a–Case4a, Case1b–Case4b, and Case1c-Case4c). The temperatures at 0 mm represent the temperature of the sodium droplet. It can be observed from that the peak temperature of sodium droplet is between 560°C and 1000°C when the sodium droplet burns completely. The distribution of the spatial maximum temperatures at 10 mm from droplet is scattered but doesn’t exceed 400°C. The spatial maximum temperatures at 20 mm is basically between 100°C and 300°C. The spatial maximum temperatures change slowly after at 20 mm away and tend to be a stable value. The spatial maximum temperature at 40 mm at different angles is relatively close and almost between 100°C and 200°C. We found the exponential correlation fits well in the distribution of spatial temperature. It can be clearly seen from the fitting curves that the spatial maximum temperatures at different angles change greatly at 0–10 mm from the center of droplet and the spatial fitting temperature at 10 mm is 450°C less than droplet. However, the spatial fitting temperature decreases relatively gently from 10 to 20 mm and the temperature difference between them is only about 100°C. The spatial fitting temperature is almost unchanged at 20 mm away, and basically stable at 150–170°C. This is because the effects of the sodium droplet on spatial temperature decrease rapidly with increasing of the distance from droplet. It can also be observed form the figure that the spatial fitting temperature at an angle of 0° is highest at 0–20 mm from droplet. The fitting formula of the space maximum temperature at three angles is embedded below.
Figure 6. Spatial maximum temperature distributions in different directions under different conditions.
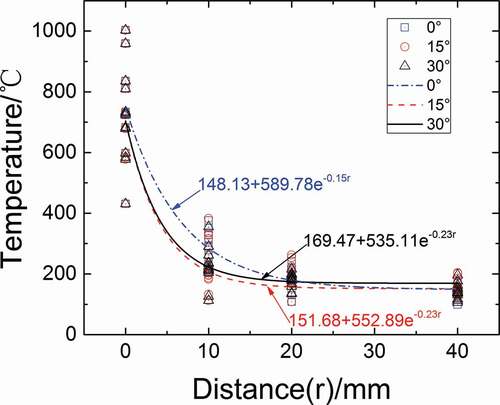
0°:T(r) = 148.13 + 589.78e−0.15r (0 mm ≤ r ≤ 40 mm, 200°C ≤T0 ≤ 350°C,12%≤C0 ≤ 21%);
15°:T(r) = 151.68 + 552.89e−0.23r (0 mm ≤r ≤ 40 mm, 200°C ≤T0 ≤ 350°C,12%≤C0 ≤ 21%);
30°:T(r)=169.47+535.11e−0.23r (0 mm ≤r ≤ 40 mm,200°C ≤T0 ≤ 350°C,12%≤C0 ≤ 21%);
where r is the distance from droplet center, mm; T(r) is the maximum space temperature, °C; T0 is the initial sodium temperature, °C; and C0 is the oxygen concentration.
The spatial maximum temperature distribution of sodium droplet could be helpful to the simulation of the sodium droplet, columnar flow and sodium spray combustion, and space temperature field more accurately.
4. Conclusion
The oxidation and combustion behaviors of liquid sodium droplet were investigated through experiments. The combustion process was visualized by a high-speed camera, and sodium temperature and spatial temperature were measured. The conclusion is summarized as follows:
(1) The ambient atmosphere temperature (80°C and 180°C) will not change the three stages of the sodium droplet combustion (surface oxidation, preignition, and combustion) but will change the characteristics in every stage. When the ambient atmosphere temperature is low (80°C), the columnar oxides are relatively obvious and the temperature of sodium changes slowly, then the temperature rises suddenly after exceeding the ignition point. When the ambient atmosphere temperature is high (180°C), the columnar oxides are not obvious and the time of combustion is shorter. The peak temperatures are all relatively close.
(2) The temperature of sodium droplet begins to increase after the gas supplied, and the spatial temperature begins to increase substantially after the sodium temperature exceeds the characteristic temperature 600°C. The temperatures of the sodium droplet and spatial measuring points reach the maximum almost at the same time. After combustion finished, the spatial temperature began to drop gradually when the temperature of droplet drops down to below 600°C. This characteristic temperature would change with the flow velocity and temperature of the airflow in the practical column flow fire and spray fire accidents. The peak temperature of sodium droplet sufficient combustion is between 560°C and 1000°C.
(3) The peak temperature of sodium droplet combustion is higher than the spatial temperature. The longer the distance from droplet is, the smaller the solid angle have, the less the radioactive heat receive, and the lower the spatial temperature is. The exponential correlation fits well in the distribution of spatial temperature at different angles. The spatial temperatures change greatly at 10 mm from droplet, and because of the relative velocity, the temperatures in 0° direction is highest at 10 mm from droplet. In the distance of 10–20 mm, the spatial temperatures change gradually, and at 20 mm away from droplet, the spatial fitting temperatures at different angles almost level off to 150–170°C.
Acknowledgments
The authors thank the National Natural Science Foundation of China [grant number 51476040] and Project of Nuclear Energy Development, for the financial support.
Disclosure statement
No potential conflict of interest was reported by the authors.
References
- Xu M. Safety charactistic on fast reactor. Nucl Sci Eng. 2011;31(2):116–126. [in Chinese].
- Sathiah P, Roelofs F. Numerical modeling of sodium fire—part I: pool combustion and combined spray and pool combustion. Nucl Eng Des. 2014;278:739–752.
- Morewitz HA. Sodium spray fires. Nucl Eng Des. 1979;55(2):275–281.
- IAEA. Liquid metal cooled reactors: experience in design and operation. Vienna: IAEA; 2007. (IAEA-TECDOC-1569).
- Olivier TJ, Radel RF, Nowlen SP, et al. Metal fire implications for advanced reactors, part 1: literature review. Livermore (CA): Sandia National Laboratories; 2007. (SAND2007-6332).
- Rao PM, Raghavan V, Velusamy K, et al. Modeling of quasi-steady sodium droplet combustion in convective environment. Int J Heat Mass Transfer. 2012;55(4):734–743.
- Richard JR, Delbourgo R, Laffitte P. Spontaneous ignition and combustion of sodium droplets in various oxidizing atmospheres at atmospheric pressure. Symp Combust. 1969;12(1):39–48.
- Tsai SS. NACOM code for analysis of postulated sodium spray fires in LMFBRs. J Educ Gifted. 1980;34(1):156–176.
- Newman RN. Ignition and burning behaviour of sodium metal in air. Prog Nucl Energy. 1983;12(2):119–147.
- Makino A. Ignition delay and limit of ignitability of a single sodium droplet: theory and experimental comparisons. Combust Flame. 2003;134(1–2):149–152.
- Makino A, Fukada H. Ignition and combustion of a falling single sodium droplet. Proc Combust Inst. 2005;30(2):2047–2054.
- Nishimura M, Kamide H, Otake S, et al. Features of dendritic oxide during sodium combustion. Nucl Sci Technol. 2011;48(12):1420–1427.
- Zhang ZG, Peng KW, Huo Y, et al. Experimental study on combustion characteristics of sodium fire in a columnar flow. J Nucl Sci Technol. 2014;51(2):166–174.
- Zhang ZG, Sun SB, Liu CC, et al. Experiment study on oxidation and combustion characteristics of sodium droplets. At Energy Sci Technol. 2015;49(4):667–673. [in Chinese].
- Huo Y, Zhang ZG, Li JK, et al. Experimental study on combustion and suppression characteristics of sodium fire in a columnar flow using extinguishing powder. J Nucl Sci Technol. 2017;54(6):716–723.