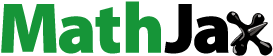
ABSTRACT
Neutron cross sections of 90,91,92,94,96Zr were calculated in the incident energy (En) range from 200 keV to 20 MeV for the revision of the 4th version of the Japanese Evaluated Nuclear Data Library (JENDL-4.0). The calculation was carried out by using conventional nuclear reaction models such as the spherical optical model, the distorted wave Born approximation, preequilibrium models, and the multi-step statistical model. Parameter values of these nuclear models were adjusted with the aid of experimental cross sections which were published after the JENDL-4.0 evaluation. Cross sections were computed for total, elastic and inelastic scattering, (n, γ), (n, 2n), (n, p), (n, α), (n, nα), and (n, x) = (n, d) + (n, np) reactions, and they were almost consistent with the experimental data. The cross sections were also estimated for the metastable states with the half-life larger than 1 sec. The obtained results well reproduced measured cross sections for the reactions 90Zr(n, 2n)89mZr, 91Zr(n, x)90mY and 91Zr(n, nα)87mSr.
1. Introduction
The fourth version of Japanese Evaluated Nuclear Data Library (JENDL-4.0 [Citation1]) was released in 2010. In this library, neutron nuclear data are available for 406 nuclides in the incident energy range from 10 eV to 20 MeV. In the JENDL-4.0 project, effort was made for improving the data of fission products and minor actinides, to contribute to innovative reactor designs and nuclear transmutation analyses. Moreover, covariance data were estimated mainly in actinides.
At present, we are engaged in the revision of JENDL-4.0. A part of our activity is being carried out for structural materials of nuclear power plants, and cross sections for metastable states will be supplemented substantially to the library for the evaluation of radioactivities.
Zirconium is an important structural material in nuclear reactors. Natural zirconium (Zr) is composed of five isotopes
Zr with the abundances 51.45% (
Zr), 11.22% (
Zr), 17.15% (
Zr), 17.38% (
Zr), and 2.80% (
Zr). Zirconium alloys are being employed in fuel rod cladding and channel boxes of fuel assemblies of light-water reactors, owing to the small absorption cross section for neutrons. Also, CuCrZr alloy is expected as a heat sink material of Tokamak fusion reactors because of its high thermal conductivity. Therefore, reliable nuclear data of Zr isotopes are required to investigate how these materials are affected in the reactors.
Under the current JENDL project,we have evaluated resonance parameters of Zr isotopes [Citation2] using recent experimental data. In the next version of JENDL-4.0, the resonance parameters of Zr will be updated on the basis of the measurements by Tagliente et al. [Citation3–Citation5] Also, above the resonance region, there are experimental cross sections [Citation6–Citation11] which were published after the JENDL-4.0 evaluation. Especially, Semkova et al. provided activation cross sections for thirteen reactions
Zr
Sr,
Zr
Y (
),
Zr
Y,
Zr
Zr,
Zr
Zr,
Zr
Sr,
Zr
Y,
Zr
Y,
Zr
Y,
Zr
Y,
Zr
Sr,
Zr
Y,
Zr
Y, including metastable state production [Citation6]. Frehaut revised the
cross section for natural zirconium
Zr [Citation10]. Filatenkov published activation cross sections in eight reactions
Zr
Sr,
Zr
Y,
Zr
Y,
Zr
Zr,
Zr
Zr,
Zr
Y,
Zr
Sr,
Zr
Zr [Citation11]. Regarding some reactions, theoretical approaches have been performed to explain the experimental data [Citation12,Citation13]. In the present study, we calculated cross sections in the incident energy
range between 0.2 MeV (above the resolved resonance region) and 20 MeV in order to improve the JENDL-4.0 data, with the aid of these measured data. We also attempted to evaluate cross sections for the reactions
Zr
Y,
Zr
Y,
Zr
Sr,
Zr
Y,
Zr
Y,
Zr
Y, which were not considered in our previous work [Citation14] and not included in JENDL-4.0.
This paper is structured as follows. The computational method and parameter values are mentioned in Section 2. Comparison of the obtained results with experimental data is presented in Section 3. Section 4 summarizes the conclusions of this study.
2. Computational method
Neutron cross sections were calculated by the CCONE code (Version 0.8.5.1) [Citation15,Citation16]. The cross sections were computed using (1) the spherical optical model, (2) the distorted-wave Born approximation (DWBA), (4) the two-component exciton preequilibrium model, and (3) the multi-step statistical model. The Kalbach particle-pickup and knockout processes [Citation17] and the reaction model of Akkermans and Gruppelaar [Citation18] were added to the preequilibrium calculation. In the statistical model calculation, the effect of width fluctuation was taken into account in
5 MeV [Citation19,Citation20].
2.1. Parameter determination
Parameters values were determined similarly with our previous work [Citation14]. Although some contents in [Citation14] are repeated, we mention the definition of major parameters for this paper.
2.1.1. Optical model
The Zr isotopes, which appear near the double magic numbers, were assumed to be spherical. The global optical model potential (OMP) proposed by Kunieda et al. [Citation21] were employed for neutrons and protons. In this study, radius parameters for incident neutrons have been tuned slightly from 1.21 to 1.215 fm in the Zr target nuclei. and show the total and elastic scattering cross sections, respectively. The compound elastic scattering component is included in the cross section. In and , the cross sections obtained from the original global spherical OMPs (Global) and the coupled-channels computations based on the rigid-rotor model (RRM-CC) by Kunieda et al. [Citation21] are also shown. The OMPs of Kunieda et al. have been developed taking into account the measured neutron strength functions related to average neutron widths in the resolved resonance region. With the modified global OMP, experimental cross sections for
Zr can be reproduced as with the RRM-CC computations by Kunieda et al. [Citation21].
Transmission coefficients for particles were computed using the global OMP proposed by Avrigeanu et al. [Citation22]. The global OMP of Lohr and Haeberli [Citation23,Citation24] was adopted for deuterons. The global OMPs of Becchetti and Greenlees [Citation24,Citation25] were used for tritons and
He.
2.1.2. DWBA
Direct inelastic scattering cross sections were computed on the basis of the experimental data of Wang and Rapaport [Citation26], the JENDL-3.2 [Citation27] and JENDL-3.3 [Citation28] evaluations. shows the values of the deformation parameter () accompanied by the excitation energy (
), spin (
), parity (
), and the angular momentum transfer quantum number (
). In , the deformation parameters derived by Wang and Rapaport have been adjusted for reproducing the measured angular distributions with the present OMPs. shows several angular distributions for
= 8 MeV. In , pseudo states have been introduced in
Zr. The parameter values were taken from JENDL-3.2 [Citation14,Citation27]. Cross sections for the pseudo states were added to the calculated neutron continuum spectra using the Gaussian distribution with the standard deviation of 0.6 MeV in order to reproduce the measured continuum spectra. The deformation parameters for
Zr were based on the JENDL-3.3 evaluation [Citation14,Citation28].
Table 1. DWBA parameters for Zr
2.2. Preequilibrium model
In the two-component exciton model calculation, the single-particle state density parameter was initially set to =
/15 for neutrons and
=
/15 for protons, where
and
are the neutron and proton numbers of the compound nucleus. Some density parameters were modified by employing the correction factor
, in order to improve the agreement with measured data. The
values are shown in the eighth column of . Both the
and
parameters were multiplied by
in the calculation.
Table 2. Nuclear-level parameters and correction factors
In evaluating the particle emission, the pickup and knockout components were modified by multiplying the correction factor
. The same factor
was applied to the pickup and knockout components. In this study,
was selected to 3.0, 0.6, 2.7, 5.0 and 5.0 for
Zr, respectively.
2.3. Statistical model
2.3.1. Level data
Nuclear discrete level data were taken from the Reference Input Parameter Library, version 3, RIPL-3 [Citation29]. Nuclear-level densities were applied above the highest discrete state to describe continuum states. On the basis of the Gilbert-Cameron formulation [Citation30], the level density of the constant temperature form () was used for lower excitation energies, and the Fermi-gas form (
) was employed for higher energies. The constant temperature density
is given in the form
=exp
as a function of excitation energy
, where the parameters
and
were determined to connect
and
smoothly at an appropriate matching energy
. In the Fermi-gas density, the
dependent Mengoni-Nakajima density parameter [Citation31] was employed together with their pairing energy and spin cutoff function. shows the highest discrete energy
applied in the calculation, the values of
,
,
and the asymptotic level density parameter
in the Mengoni-Nakajima density, for each nucleus. In , most
values were taken from [Citation31], while the values in
Y were altered to reproduce the experimental cross sections. Moreover, the correction factor
has been introduced to adjust the spin cutoff function by multiplying
. The
values are shown in the seventh column of .
2.3.2. 
-ray strength function
The standard Lorentzian (SLO) form was adopted for the -ray strength functions in the E1, M1 and E2 radiations, since the
dependence of measured capture cross sections was better reproduced by SLO than the generalized Lorentzian (GLO) [Citation32]. The values of peak cross section, position and width parameters in the Lorentzian functions have been estimated using the systematic formulas built in the CCONE code [Citation16] on the basis of RIPL-1 [Citation33] and RIPL-2 [Citation34]. The Lorentzian functions for
Zr targets have been normalized so as to obtain the
cross sections (
) measured by Ohgama et al. [Citation35,Citation36] and Katabuchi et al. [Citation7] by adopting
= 6.6 mb at
=550 keV for
Zr [Citation35], 32.3 mb at 70 keV for
Zr [Citation36], 11.6 mb at 550 keV for
Zr [Citation36], 9.3 mb at 550 keV for
Zr [Citation35], and 19.2 mb at 21 keV for
Zr [Citation7]. The contributions of M2 and E2 components relative to E1 were estimated by
)
[Citation37] and
[Citation38], where
is the nuclear mass number. The intensities of
-rays decaying to the ground and first excited states from the primary compound state were multiplied by factors of 2 and 3 in
Zr, and by 3 and 1.5 in
Zr. illustrates the
-ray spectra from the
reaction of
Zr at
=550 keV. The total
-ray emission strength has been normalized with the measured cross section after scaling the
-ray emission spectra. In the
-ray spectra measured by Ohgama et al. [Citation36,Citation39] are well reproduced by the SLOs applied.
3. Results and comparison with experimental and evaluated data
Calculated results were compared with experimental data, the JENDL-4.0 evaluations in which our previous results [Citation14] were reflected, and the evaluated values in other major libraries ENDF/B-VIII.0 [Citation40], JEFF-3.2 (for the metastable states) [Citation41], and JEFF-3.3 [Citation42]. The experimental data were obtained from the EXFOR database [Citation43] that is compiled by the International Network of Nuclear Reaction Data Centers coordinated by the IAEA Nuclear Data Section.
- show the cross sections for
Zr. In - cross sections were calculated down to 10 keV in order to compare with the measured average cross sections, where the upper limits of resolved resonances are 171, 26, 71, 53.5 and 100 keV for
Zr in JENDL-4.0 [Citation1]. To obtain the
dependence of the measured cross sections by Ohgama et al. [Citation35,Citation36], the correction factor
of the spin cutoff function in the statistical model was adjusted in
Zr nuclei. It can be seen that the computed cross sections accord well with the experimental data. In the
Zr
Zr reaction, the present result is similar to the ENDF/B-VIII.0 and JENDL-4.0 evaluations. In
Zr
Zr, the
dependence of the present cross section is close to the ENDF/B-VIII.0 and JEFF-3.3 evaluations. The JENDL-4.0 data adopts the JENDL-3.3 evaluation [Citation28], whose energy dependence could not be obtained within our model calculation. In , it is seen that the measured
Zr
Zr data by Ohgama et al. [Citation36] are well reproduced by our calculation. In , we could not obtain the
Zr
Zr cross section which reproduces the experimental data for 550 keV by Ohgama et al. [Citation35] and for 2.45 MeV by Prajapati et al. [Citation8] simultaneously. In the
Zr target nuclei, the cross sections have been normalized at 550 keV using the data measured by Ohgama et al. [Citation35,Citation36] With these cross sections, the capture cross section of
Zr in measured by Poenitz [Citation44] are well reproduced around 1 MeV. In and , the plausible cross sections were obtained for
Zr.
In , the capture cross section of Zr, which is a long-lived fission product (LLFP) with a half-life (T
) of 1.61
10
years, was estimated by employing the global OMP of Kunieda et al. [Citation21] and nuclear-level parameters in . The cross section was normalized at 30 keV with the recommended value 95
10 mb of Mughabghab [Citation45]. JEFF-3.3 adopts the ENDF/B-VIII.0 evaluation. All the evaluations and our computation have the similar
dependence up to 1 MeV. Above 1 MeV the present cross section decreases more rapidly than other evaluations. This comes from the fact that the
= 949.8 keV excited state with
=4.5+ [Citation29,Citation46,Citation47] was included in the target
Zr. If this state is not included, the resulting cross section is close to the JEFF-3.2 evaluation.
- show the cross sections for
Zr. In
Zr, the cross sections for the metastable (m.s.) state are also shown. To obtain the cross sections, the correction factor
of the single-particle state density in the preequilibrium model was adjusted. Also, the
value was selected in
Zr to produce the
Zr
Zr cross section (
Zr:
= 587.82 keV,
= 1/2
, T
= 4.161 m [Citation47]). In , the
Zr
Zr cross sections are reproduced quantitatively by our calculation. In and , the calculated cross sections accord with the experimental data for
Zr.
- show the cross sections for
Zr. In this study the nuclear level parameters and correction factors for
Y have been adjusted by referring to the experimental
Zr
Y and
Zr
Y cross sections (
Y:
= 681.67 keV,
=7+, T
= 3.19 h.
Y:
= 908.97 keV,
= 9/2+, T
= 15.663 s [Citation47]). Similarly, the parameters for
Y were selected when evaluating the
Zr
Y,
Zr
Y and
Zr
Y cross sections (
Y:
= 555.58keV,
= 9/2+, T
= 49.71 m [Citation47]). In , the calculated
Zr
Y cross section is close to the ENDF/B-VIII.0 evaluation for
14 MeV. In the
Zr
Y reaction, the reproducibility of the measured data is slightly improved compared with the JEFF-3.2 evaluation. In , we could not reproduce the measured
Zr
Y cross section in
10 MeV. To increase the cross section in this energy region as with ENDF/B-VIII.0 and JEFF-3.3, the level density of
Y in the statistical model could be increased, but that led to the unacceptably large cross section for
10 MeV and overestimated the
Zr
Y and
Zr
Y () cross sections. In , we could obtain the
Zr
Y cross section which is almost consistent with the experimental data. In , the parameter values for the proton emission were selected to reproduce the experimental
Zr
Y and
Zr
Y () cross sections. The present
Zr
Y cross section is slightly larger than the measured cross section for
17 MeV.
- show the cross sections for
Zr. In each figure, the obtained
dependence is almost consistent with the experimental data. In and , the present
Zr
Y and
Zr
Y cross sections are close to the JENDL-4.0 evaluations. In , our result overlaps with the JEFF-3.3 evaluation. The
dependence of the measured
Zr
Y cross section could be reproduced by JEFF-3.3 and the present computation. The JENDL-4.0 evaluation underestimated the cross section.
- show the cross sections for
Zr, and gives the
Zr
Sr cross section. In , our
Zr
Sr (
Sr:
= 388.53 keV,
=1/2
, T
= 2.815 h [Citation47]) cross section is smaller than the experimental data for
15 MeV. We could not obtain the cross section which is almost flat for
12 MeV by the calculation. In and , the computed cross sections for
Zr target nuclei accord well with the experimental cross sections. In , the
Zr
Sr cross section has been evaluated so as to be consistent with the experimental data by Ikeda et al. [Citation48] as with ENDF/B-VIII.0. It is noted that the present result was obtained by multiplying the preequiriblium pickup and knockout components by the factor (
) of 5. In , the computed
Zr
Sr cross section agrees with the measured data. The JENDL-4.0 evaluation underestimated the cross section.
Finally, we calculated the energy-differential and angle-energy double-differential cross sections (EDX and DDX) for emitted neutrons to test the validity of present work. The experimental data were taken from the EXFOR [Citation43] data base. shows the neutron EDXs. The EDXs (and DDXs) were derived from ENDF/B-VIII.0, JEFF-3.3 and JENDL-4.0 by using the modified version of the PLDDX code [Citation49]. In , elastic and inelastic scattering cross sections for discrete states were added to the spectra using the Gaussian distribution with the standard deviation of 0.3 MeV. It can be seen that the experimental EDXs are reproduced by the present calculations as well as other evaluations. shows the neutron DDXs for 14.1 MeV. The measured DDXs are reasonably reproduced by our calculation.
4. Summary and concluding remarks
Toward the revision of JENDL-4.0, neutron cross sections of Zr were computed in the energy range
between 200 keV and 20 MeV. The calculation was performed on the basis of nuclear reaction models which deal with the compound, preequilibrium, and direct processes. The cross sections were computed for total, elastic and inelastic scattering
,
,
,
,
, and
reactions. The results accord well with the experimental data in most reactions. The cross sections for the reactions
Zr
Y and
Zr
Sr underestimated in JENDL-4.0 were improved by the present calculation. The results better than our previous work were derived by adjusting parameter values more precisely with correction factors and by referring to more experimental data published after the JENDL-4.0 evaluation. Also, cross sections were estimated for the metastable states which had not been considered in the JENDL-4.0 evaluation. In the consistent computation, we could obtained the results which reproduce the experimental data in the reactions
Zr
Zr,
Zr
Y and
Zr
Sr. Moreover, we estimated the cross section for
Zr
Zr, which is important for the transmutation of LLFP, by using the parameter values obtained in the natural isotopes. These data will be applied for the revision of JENDL-4.0.
Acknowledgments
The author would like to thank Dr. K. Ohgama for kindly sending their numerical data of capture -ray spectra to us, and thank Dr. O. Iwamoto for providing CCONE version 0.8.5.1. The author is grateful to all members of JAEA/NDC for their helpful advice and encouragement during this work.
Disclosure statement
No potential conflict of interest was reported by the author.
References
- Shibata K, Iwamoto O, Nakagawa T, et al. JENDL-4.0: a new library for nuclear science and engineering. J Nucl Sci Technol. 2011;48:1–30.
- Ichihara A. Evaluation of neutron resonance parameters for Zr itotopes. In: Sanami T, Nishio K, Hagiwara M, et al. editors. Proceedings of the 1016 symposium on nuclear data. Tsukuba, Japan. Tokai (Japan): Japan Atomic Energy Agency; 2016 Nov 17-18. p. 103–108. (JAEA-Conf 2017-001).
- Tagliente G, Milazzo PM, Fujii K, et al. The 92Zr(n,γ) reaction and its implications for stellar nucleosynthesis. Phys Rev C. 2010;81:055801-1-055801-9.
- Tagliente G, Milazzo PM, Fujii K, et al. Neutron capture on 94 Zr: resonance parameters and Maxwellian-averaged cross sections. Phys Rev C. 2011;84:015801-1-015801-9.
- Tagliente G, Milazzo PM, Fujii K, et al. 96Zr(n,γ) measurement at the n_TOF facility at CERN. Phys Rev C. 2011;84:055802-1-055802-8.
- Semkova V, Bauge E, Plompen AJM, et al. Neutron activation cross sections for zirconium isotopes. Nucl Phys A. 2010;832:149–169.
- Katabuch T, Igashira M, Kiyanagi Y. Measurement of keV-neutron capture cross section of 96 Zr. J Nucl Sci Technol. 2011;48:744–750.
- Prajapati PM, Mukherjee S, Naik H, et al. Measurement of neutron-induced reaction cross sections in zirconium isotopes at thermal, 2.45 MeV and 9.85 MeV energies. Nucl Sci Eng. 2012;171:78–84.
- Champine B, Gooden ME, Krishichayan F, et al. Measurement of the 169Tm (n, 3n) 167Tm cross section and the associated branching ratios in the decay of 167Tm. Phys Rev C. 2016;93:014611-1-014611-8.
- Frehaut J. Le point sur les sections efficaces de reaction (n, xn) mesurées a Bruyères-le-Châtel [The (n, xn) reaction cross sections measured at Bruyères-le-Châtel]. Saclay (France): Commissariat à l’énergie atomique et aux énergies alternatives; 2016. (CEA-R-6447). French.
- Filatenkov AA. Neutron activation cross sections measured at KRI in neutron energy region 13.4-14.9 MeV. Vienna: International Atomic Energy Agency; 2016. (INDC(CCP)-0460 Rev).
- Suchiang D, Jeremiah JJ, Jyrwa BM, et al. Excitation functions of the 90, 91, 92, 94, 96 Zr(n,p) reactions from reaction threshold to 20 MeV. Ann Nucl Energy. 2014;65:97–100.
- Kara A. Excitation function calculations of neutron-induced reactions of some zirconium target isotopes. J Fusion Energ. 2017;36:230–233.
- Ichihara A, Kunieda S, Shibata K. Calculation of neutron cross sections on 90, 91, 92, 94, 96 Zr for JENDL-4. J Nucl Sci Technol. 2009;46:1076–1084.
- Iwamoto O. Development of a comprehensive code for nuclear data evaluation, CCONE, and validation using neutron-induced cross sections for uranium isotopes. J Nucl Sci Technol. 2007;44:687–697.
- Iwamoto O, Iwamoto N, Kunieda S, et al. The CCONE code system and its application to nuclear data evaluation for fission and other reactions. Nucl Data Sheets. 2016;131:259–288.
- Kalbach C. The Griffin model, complex particles and direct nuclear reactions. Z Phys A. 1977;283:401–411.
- Akkermans JM, Gruppelaar H. Analysis of continuum gamma-ray emission in precompound-decay reactions. Phys Lett. 1985;157B:95–100.
- Gruppelaar H, Reffo G. Some properties of the width fluctuation factor. Nucl Sci Eng. 1977;62:756–763.
- Moldauer PA. Statistics and the average cross section. Nucl Phys A. 1980;344:185–195.
- Kunieda S, Chiba S, Shibata K, et al. Coupled-channels optical model analyses of nucleon-induced reactions for medium and heavy nuclei in the energy region from 1 keV to 200 MeV. J Nucl Sci Technol. 2007;44:838–852.
- Avrigeanu V, Hodgson PE, Avrigeanu M. Global optical potentials for emitted alpha particles. Phys Rev C. 1994;49:2136–2141.
- Lohr JM, Haeberli W. Elastic scattering of 9-13 MeV vector polarized deuterons. Nucl Phys A. 1974;232:381–397.
- Perey CM, Perey FG. Compilation of phenomenological optical-model parameters 1954-1975. Atom Data Nucl Data Tables. 1976;17:1–101.
- Becchetti FD, Greenlees GW. A general set of 3He and triton optical-model potentials for A > 40, E < 40 MeV. In: Barshall HH, Haeberli W, editors. Proceedings of the third international symposium on polarization phenomena in nuclear reactions. Madison (WI): University of Wisconsin Press; 1970 Aug 31. p. 682–683.
- Wang Y, Rapaport J. Neutron scattering from 90, 91, 92, 94 Zr. Nucl Phys A. 1990;517:301–328.
- Nakagawa T, Shibata K, Chiba S, et al. Japanese evaluated nuclear data library version 3 revision-2: JENDL-3.2. J Nucl Sci Technol. 1995;32:1259–1271.
- Shibata K, Kawano T, Nakagawa T, et al. Japanese evaluated nuclear data library version 3 revision-3: JENDL-3.3. J Nucl Sci Technol. 2002;39:1125–1136.
- Capote R, Herman M, Obložinský P, et al. RIPL-reference input parameter library for calculation of nuclear reactions and nuclear data evaluations. Nucl Data Sheets. 2009;110:3107–3214.
- Gilbert A, Cameron AGW. A composite nuclear-level density formula with shell corrections. Can J Phys. 1965;43:1446–1496.
- Mengoni A, Nakajima Y. Fermi-gas model parameterization of nuclear level density. J Nucl Sci Technol. 1994;31:151–162.
- Kopecky J, Uhl M. Test of gamma-ray strength functions in nuclear reaction model calculations. Phys Rev C. 1990;41:1941–1955.
- Nuclear Data Section. Handbook for calculations of nuclear reaction data, reference input parameter library. Vienna: International Atomic Energy Agency; 1998. (IAEA-TECDOC-1034).
- Belgya T, Bersillon O, Capote Noy R, et al. Handbook for calculations of nuclear reaction data, RIPL-2. Vienna: International Atomic Energy Agency; 2006. (IAEA-TECDOC-1506).
- Ohgama K Systematic study on keV-neutron capture reaction cross sections and capture γ-ray spectra of Zr isotopes [ dissertation]. Tokyo: Tokyo Institute of Technology; 2004. Japanese.
- Ohgama K, Igashira M, Ohsaki T. Measurement of keV-neutron capture cross sections and capture gamma-ray spectra of 91, 92 Zr. J Nucl Sci Technol. 2005;42:333–340.
- Ichihara A, Iwamoto O, Chiba S, et al. Program POD; a computer code to calculate cross sections for neutron-induced nuclear reactions. Tokai (Japan): Japan Atomic Energy Agency; 2007. (JAEA-Data/Code 2007-012).
- Young PG, Arthur ED, Chadwick MB. Comprehensive nuclear model calculations: introduction to the theory and use of the GNASH code. Los Alamos, (US): Los Alamos National Laboratory; 1992. (LA-12343-MS).
- Ohgama K, Igashira M, Ohsaki T. Measurement of gamma rays from keV-neutron capture reaction by Zr-90,94. In: Woehr A, Aprahamian A, editors. Proceedings of the 12th international symposium on capture gamma-ray spectroscopy and related topics. Notre Dame, IN. Melville (NY): AIP Publishing; 2005 Sep 4-9. p. 373–377.
- Brown DA, Chadwick MB, Capote R, et al. ENDF/B-VIII.0: the 8th major release of the nuclear reaction data library with CIELO-project cross sections, new standards and thermal scattering data. Nucl Data Sheets. 2018;148:1–142.
- OECD Nuclear Energy Agency Data Bank [cited 2018 Feb 21]. Available from: http://www.oecd-nea.org/dbforms/data/eva/evatapes/jeff_32/
- OECD Nuclear Energy Agency Data Bank [cited 2018 Feb 21]. Available from: https://www.oecd-nea.org/dbdata/jeff/jeff33/
- Otuka N, Dupont E, Semkova V, et al. Towards a more complete and accurate experimental nuclear reaction data library (EXFOR): international collaboration between nuclear reaction data centers (NRDC). Nucl Data Sheets. 2014;120:272–276.
- Poenitz WP. Fast-neutron capture-cross-section measurements with the argonne national laboratory large-liquid-scintillator tank. In: Smith AB, Poenitz WP, editors. Proceedings of the NEANDC/NEACRP specialist’s meeting on fast-neutron capture cross sections. Argonne, IL: Argonne National Laboratory; 1982 Apr 20-23. p. 239–247. (ANL-83-4).
- Mughabghab SF. Atlas of neutron resonances: resonance parameters and thermal cross sections Z=1-100. Amsterdam: Elsevier; 2006.
- Baglin CM. Nuclear data sheets for A = 93. Nucl Data Sheets. 2011;112:1163–1389.
- National nuclear data center, Brookhaven National Laboratory [cited 2018 Feb 28]. Available from: http://www.nndc.bnl.gov/ensdf/index.jsp
- Ikeda Y, Konno C, Oishi K, et al. Activation cross section measurements for fusion reactor structural materials at neutron energy from 13.3 to 15.0 MeV using FNS facility. Tokai (Japan): Japan Atomic Energy Research Institute; 1988. (JAERI-1312).
- Fukahori T, Chiba S, Asami T. Comparison of double-differential neutron emission cross sections calculated from evaluated nuclear data libraries with experimental data. Tokai (Japan): Japan Atomic Energy Research Institute; 1992. (JAERI-M 92-053).